- Physics Article
- To Determine Refractive Index Of A Glass Slab Using A Travelling Microscope

To Determine Refractive Index of a Glass Slab Using a Travelling Microscope
A Travelling microscope is a compound microscope that is fitted on a vertical scale. It carries a vernier scale along the main scale and can be moved upward or downward. Below is an experiment to determine refractive index of a glass slab using a travelling microscope.
To determine the refractive index of a glass slab using a travelling microscope.
Materials Required
- 3 glass slabs of different thicknesses but the same material
- A travelling microscope
- Lycopodium powder
The principle behind glass slab
When a glass slab is placed on a horizontal surface, and its bottom surface is viewed from the top, it appears to be elevated due to refraction. The apparent thickness of the slab is determined by the distance between the apparent bottom and the top of the glass slab. The refractive index with respect to the medium and air is given as:
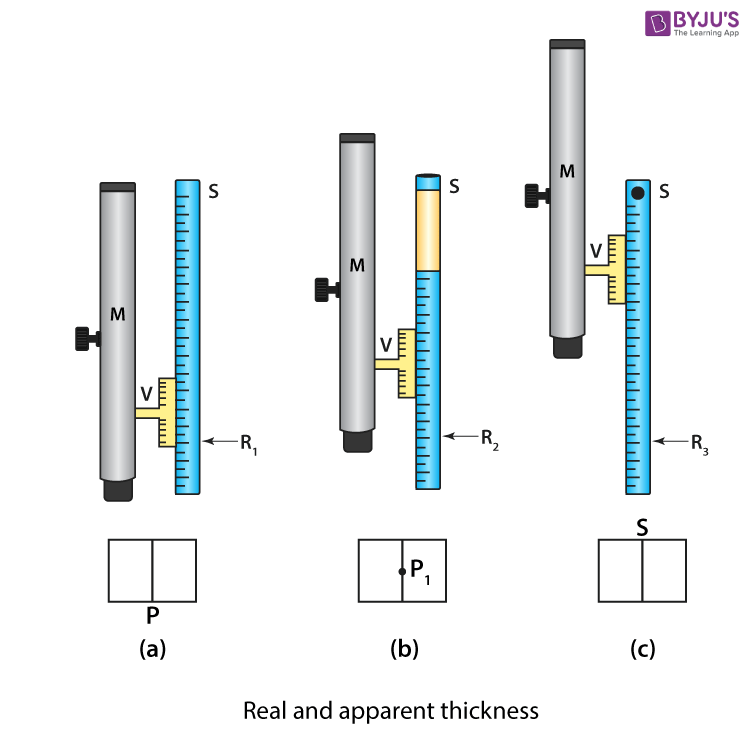
Read More: Refractive Index
Adjustment of a travelling microscope
- To get sufficient light, place the travelling microscope (M) near the window.
- To make the base of the microscope horizontal, adjust the levelling screw.
- For clear visibility of the cross wire, adjust the position of the eyepiece.
- For the vertical scale of the microscope, determine the vernier constant.
- Mark point P on the microscope’s base using black ink.
- To avoid the parallax between the cross-wires and the mark P, make the microscope vertical and focus on P.
- Let R 1 be the vernier scale and main scale reading on the vertical scale.
- Place the glass slab with the least thickness over the mark P.
- Let P 1 be the image of the cross mark. Move the microscope upwards and focus on P 1 .
- For reading, R 2 on the vertical scale repeat step 7.
- Sprinkle a few particles of lycopodium powder on the slab’s surface.
- To focus the particle near S, raise the microscope further upward.
- For reading, R 3 on the verticle scale repeat step 7.
- Repeat the above steps for different thickness glass slabs.
- Record the observations.
Observations and Calculations
Vernier constant for the vertical scale of microscope = ……..cm
Table for microscope readings
– R ) in cm | – R ) in cm | |||||
in cm | in cm | in cm | ||||
The ratio \(\begin{array}{l}\frac{R_{3}-R_{1}}{R_{3}-R_{2}}\end{array} \) is constant and gives the refractive index of the glass slab.
Precautions
- The parallax in a microscope should be removed properly.
- To avoid backlash error, the microscope should be moved upward.
Sources Of Error
- The scale used in the microscope might not be calibrated properly.
- The lycopodium powder layer on the glass slab might be thick.
Viva Questions
Q1. Define normal shift.
Ans: Normal shift is defined as the difference between actual depth and apparent depth.
Q2. What causes a normal shift?
Ans: Normal shift is caused due to the refraction of light.
Q3. What is the SI unit of normal shift?
Ans: The SI unit of normal shift is a metre.
Q4. What is apparent shift?
Ans: Apparent shift is defined as the difference between the object’s distance from the refracting surface and the image distance from the refracting surface.
Q5. On what factors does the apparent depth depend?
Ans: Following are the factors on which the apparent depth depend:
- Nature of the medium
- Thickness of medium
- Colour of light
|
|
|
|
|
|
|
Stay tuned with BYJU’S to learn more about other Physics-related experiments.
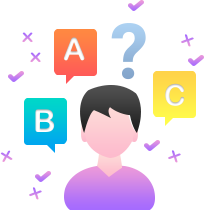
Put your understanding of this concept to test by answering a few MCQs. Click ‘Start Quiz’ to begin!
Select the correct answer and click on the “Finish” button Check your score and answers at the end of the quiz
Visit BYJU’S for all Physics related queries and study materials
Your result is as below
Request OTP on Voice Call
PHYSICS Related Links | |
Leave a Comment Cancel reply
Your Mobile number and Email id will not be published. Required fields are marked *
Post My Comment

Register with BYJU'S & Download Free PDFs
Register with byju's & watch live videos.
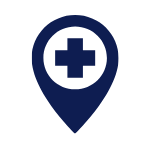
+91-8527246961
Attractive Offers
Upto 60% Off
- Bulk Order Enquiry!
Travelling Microscope: Exploring the World Beyond the Naked Eye
- May 20, 2024
Introduction to the Travelling Microscope
The history of microscopes and its evolution, how a travelling microscope works, applications of a travelling microscope, advantages and limitations of a travelling microscope, famous discoveries made with the help of a travelling microscope, tips for using a travelling microscope.
Welcome to the fascinating world of the travelling microscope! Prepare to embark on a journey that will take you beyond the limits of your naked eye and into a microscopic realm filled with wonder and discovery. In this blog post, we will delve into the history, workings, applications, advantages, limitations, and famous discoveries made possible by this incredible scientific instrument. So fasten your seatbelts and get ready for an adventure like no other as we explore the captivating world of the travelling microscope!
The history of microscopes is a fascinating journey that reveals humanity’s insatiable curiosity about the world around us. It all began in the late 16th century, when Dutch spectacle makers discovered that by placing two lenses together, they could magnify objects and unlock a hidden realm of tiny details. This groundbreaking discovery paved the way for the development of more sophisticated microscopes over the centuries. In 1665, Robert Hooke published his famous book “Micrographia,” which showcased detailed illustrations of insects and plant cells observed under a microscope. In the 19th century, advancements in lens manufacturing techniques led to improved image quality and increased magnification capabilities. Ernst Abbe’s breakthrough in developing an oil immersion objective lens further enhanced resolution and clarity. Then came along Antonie van Leeuwenhoek, often referred to as the father of microscopy. With his handmade single-lens microscopes, he made remarkable discoveries such as bacteria and spermatozoa — opening up new frontiers for scientific exploration. As technology advanced, so did microscopes. The introduction of electron microscopy in the mid-20th century revolutionized our understanding of cellular structures at an atomic level. Today, we have powerful instruments like scanning electron microscopes (SEMs) and transmission electron microscopes (TEMs), capable of revealing intricate details on nanoscale dimensions. The evolution of microscopes has been driven by not only technological advancements but also by scientists’ unrelenting quest for knowledge. From simple magnifying glasses to high-tech imaging devices, these tools have played an indispensable role in expanding our understanding across various fields – from biology to materials science. Indeed, it is awe-inspiring to think about how far we’ve come since those early days when pioneers first peered through their primitive lenses into a microscopic world waiting to be explored further!
How does a travelling microscope work? Let’s delve into the fascinating inner workings of this remarkable scientific instrument. At its core, a travelling microscope consists of a magnifying lens or set of lenses mounted on an adjustable arm that can be moved horizontally and vertically. This allows for precise positioning and focusing on the object being observed. The light source is crucial in illuminating the specimen under examination. Typically, a mirror reflects natural or artificial light onto the sample, enhancing visibility. To achieve greater magnification, additional lenses are often used in conjunction with the main objective lens. These lenses help to further enlarge the image formed by the primary lens, allowing for detailed examination at microscopic levels. A key feature of a travelling microscope is its ability to measure distances accurately. This is done using a calibrated screw mechanism that moves the stage holding the specimen horizontally or vertically. By noting how far it travels along its scale when observing different parts of an object, precise measurements can be obtained. In addition to measuring distances, these microscopes also have features like vernier scales or micrometers which allow for even more accurate measurements. These tools enable scientists and researchers to quantify their observations and record data with precision. By combining excellent optics with careful engineering design, travelling microscopes provide invaluable insights into our world at minuscule scales. From examining tiny organisms to analyzing intricate structures within materials, these instruments play a vital role in various scientific fields and continue to push boundaries in exploration and discovery.
The applications of a travelling microscope are vast and varied, making it an indispensable tool in various fields. One of its primary uses is in the field of biology, where it allows scientists to closely examine tiny organisms and cells. By magnifying these specimens, researchers can study their structures and functions in detail, paving the way for advancements in medicine and genetics. In addition to biology, the travelling microscope finds practical applications in other scientific disciplines as well. In materials science, it enables researchers to analyze the microstructure of different materials such as metals or polymers. This helps determine their quality, strength, and suitability for specific purposes like construction or manufacturing. Furthermore, archaeologists also rely on travelling microscopes when studying ancient artifacts. These powerful devices enable them to observe minute details like engravings or markings that may provide valuable insights into past civilizations. Another fascinating application lies within the world of art restoration. Travelling microscopes allow conservators to closely examine paintings and sculptures at a microscopic level. They can identify cracks or damages not visible to the naked eye and carry out delicate repair work with precision. Moreover, this versatile instrument plays a crucial role in forensic investigations by aiding experts in analyzing trace evidence such as hair fibers or fingerprints left at crime scenes. From scientific research to archaeological exploration and even criminal investigations – the applications of a travelling microscope extend far beyond what meets the eye! Its ability to reveal hidden worlds has revolutionized numerous fields, unlocking endless possibilities for discovery and understanding.
When it comes to exploring the microscopic world, a travelling microscope offers several advantages. Its portability allows scientists and researchers to take it with them on field trips or expeditions. This means they can study specimens in their natural habitats rather than relying solely on laboratory observations. A travelling microscope provides high magnification capabilities, allowing users to see intricate details that would otherwise be invisible to the naked eye. This is especially useful for studying tiny organisms or examining delicate structures such as cells or microorganisms. Additionally, a travelling microscope often comes with various attachments and accessories that enhance its functionality. These may include different lenses for varying levels of magnification, adjustable stages for precise positioning of samples, and built-in lighting systems for optimal illumination. However, like any tool, there are also limitations associated with using a travelling microscope. One major limitation is the restricted field of view due to its compact size. This means that only small specimens can be observed at one time and larger objects may need to be examined in parts. Another limitation is the need for proper calibration and alignment before each use. Any misalignment could lead to inaccurate measurements or blurry images which can hinder research outcomes. While portable microscopes have come a long way in terms of technology advancements over the years, they still cannot match up to more advanced laboratory-grade microscopes in terms of resolution or image quality.
- Antonie van Leeuwenhoek and Microorganisms: In the 17th century, Dutch scientist Antonie van Leeuwenhoek made groundbreaking discoveries using his homemade microscope. With this instrument, he observed microorganisms for the first time, including bacteria and protozoa. This discovery revolutionized our understanding of life on a microscopic level. 2. Robert Hooke and Cell Structure: English scientist Robert Hooke is known for his work in microscopy during the 17th century as well. He used a simple compound microscope to examine thin slices of cork and discovered tiny compartments which he called “cells.” This observation led to the development of cell theory, one of biology’s fundamental principles. 3. Louis Pasteur and Germ Theory: Louis Pasteur, a French chemist and microbiologist in the 19th century, utilized powerful microscopes to study fermentation processes. Through his experiments with microbes, he developed germ theory, which explained how diseases are caused by specific germs or microorganisms. 4. Gregor Mendel’s Genetics Studies: Gregor Mendel conducted groundbreaking experiments on pea plants in the mid-1800s that laid the foundation for modern genetics. Using a travelling microscope to observe plant traits at a microscopic level allowed him to formulate laws governing inheritance patterns. 5. Rosalind Franklin’s Work on DNA Structure: Rosalind Franklin played an instrumental role in uncovering DNA’s double helix structure; her X-ray crystallography images were critical evidence supporting this discovery by James Watson and Francis Crick in 1953. These are just some examples highlighting how travelling microscopes have been essential tools throughout history for scientific advancements that have shaped our understanding of biology and beyond!
Using a travelling microscope can be an exciting and fascinating experience, allowing you to explore the world beyond what the naked eye can see. To make the most of this powerful tool, here are some helpful tips: 1. Set up in a well-lit area: Proper lighting is crucial when using a travelling microscope. Make sure you have adequate light to illuminate your specimen and enhance visibility. 2. Clean the lenses: Before starting your observations, ensure that both the eyepiece and objective lenses are clean and free from dust or smudges. This will help maintain clarity in your images. 3. Adjust focus carefully: Take your time to adjust the focus properly for each observation. Move the fine focus knob slowly until you achieve a clear image without any blurriness. 4. Use slide preparations: When examining small specimens, it’s beneficial to prepare slides with thin sections or mounted samples for easier viewing under the microscope. 5. Experiment with different magnifications: Travelling microscopes often come with multiple objective lenses that provide various levels of magnification. Don’t hesitate to switch between them to get closer looks at different features of your specimen. 6. Keep records of observations: It’s essential to document your findings while using a travelling microscope so that you can refer back to them later on or share them with others who may be interested in your discoveries. Remember, practice makes perfect when it comes to using a travelling microscope effectively! So don’t be discouraged if you encounter difficulties initially – keep experimenting and exploring new subjects under its lens!
The travelling microscope is an incredible tool that has revolutionized our ability to explore and understand the world beyond what our naked eye can see. Throughout history, microscopes have evolved from simple optical devices to sophisticated instruments with advanced features. The travelling microscope, in particular, offers enhanced mobility and versatility, making it a valuable asset in various fields of study. With its ability to magnify and measure objects accurately, the travelling microscope finds applications in fields such as biology, geology, physics, and material science. Researchers rely on this instrument to observe microscopic organisms and structures, analyze minerals and crystals at high resolutions or perform precise measurements for scientific experiments. While there are numerous advantages associated with using a travelling microscope – such as portability, ease of use, and accurate measurements – it’s important to acknowledge its limitations as well. Factors like limited field of view or depth perception can pose challenges when working with certain samples or specimens. Over time, many groundbreaking discoveries have been made possible through the use of a travelling microscope. From identifying cells for medical research to unraveling mysteries of atomic structures in materials science – this remarkable instrument continues to push boundaries and expand our knowledge across various disciplines. For those venturing into the world of microscopy with a traveling scope for the first time, here are some tips: make sure you handle your equipment carefully; adjust lighting conditions accordingly; take advantage of different magnification levels; keep your slides clean; practice proper focusing techniques; document your observations effectively. All in all, the advent of the travelling microscope has opened up new vistas for exploration at an incredibly small scale. This powerful tool allows scientists, researchers, and enthusiasts alike, to delve deeper into nature’s intricate details. So, let’s embrace this incredible invention that helps us discover hidden worlds right before our eyes!
Leave a Reply Cancel reply
Your email address will not be published. Required fields are marked *
Save my name, email, and website in this browser for the next time I comment.
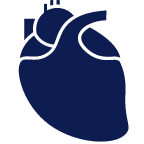
- Crucible Tongs
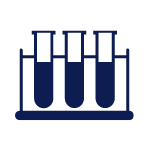
Username or email address *
Password *
Lost your password? Remember me
No account yet?
Talk to our experts
1800-120-456-456
- Determine Refractive Index of a Glass Slab using a Travelling Microscope
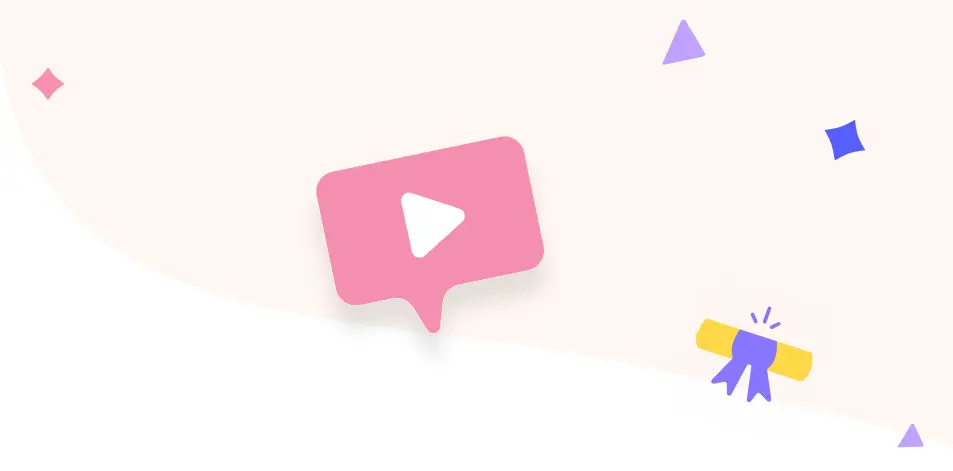
Refractive Index of a Glass Slab
Objective: We will design a system to measure the refractive index of a glass slab using a moving microscope.
Introduction: A glass slab is a piece of glass with thickness ranging from a few millimeters to several meters. The refractive index of a glass slab is the ratio of the index of refraction to the thickness of the glass. Measurement of the refractive index of glass is important for optical applications like optical fiber and photonic crystal.
Structure: There are various methods for measuring the refractive index. We will measure the refractive index using a moving microscope.
Step 1: What will we need?
( Note: the same items are also required to create a light microscope)
We will start the design by looking for an item that can be used as both a lens and a holder. The most ideal item would be a microscope lens since we can use it to focus light on the glass slab and to measure the distance. We can also use it as a holder if we have to insert another lens to take a refractive index measurement.
We can use a microscope lens holder to attach the microscope lens in place. The microscope lens we will use is called an objective lens. The objective lens has a central convex lens that magnifies an object to be magnified.
Since the lens holder is circular, we can make the convex lens circular. We can use a microscope objective lens as a holder because the thickness of the lens holder is quite small.
We can buy the lens holder at a local store. However, we may have to make it from scratch. We will need a microscope, a microscope objective lens, a stepper motor, a potentiometer, and an Arduino board. We will describe the building process in the next section.
Step 2: Building process
Step 2.1: Choosing a Microscope
We should buy a microscope to work with. First, we should consider the following when we are buying a microscope.
Whether it is a microscope made in a lab or one in a store. Amount of microscope that we want to buy. What brand name is most popular in stores, but can be bought for a cheaper price Next, we should decide where to mount the microscope. We should decide the size of the slab.
Also, if we use the same microscope for different experiments, we should decide whether we should buy a holder that can hold different lenses. If so, which size is most convenient?
Also, a microscope has to be able to magnify the object we are measuring the refractive index. We can decide whether the microscope has a magnification of 1x, 4x, 10x, 20x, and 40x.
2.1.1 Choosing a microscope
You can buy a microscope in the market.
Or, if you don't want to buy one, then you can ask your teacher for help. In any case, we can choose a microscope of the following three types.
Microscope made in a laboratory
Microscope made in a store
Electron microscope
Now we will start the buying process.
Microscope Made in a Lab
The easiest way to start the process of buying a microscope is to ask your teacher to help. In this case, your teacher is going to give you instructions on buying a microscope for your experiments. There are many types of microscopes. Your teacher will give you some instructions.
Microscopes Made in a Store
If you don't have enough money to buy the microscope made in a laboratory, then you can buy one in a store. First, you have to buy the microscope itself. To do this, you have to visit a store of the type you want. Once you have seen the types of microscopes, the price of each one, and its functions, you will be able to choose the microscope that you need.
Electron Microscope
An electron microscope is an extremely high-tech microscope. We can't get an electron microscope at home. We have to find the store of the type we want to buy.
The advantage of an electron microscope over a general microscope is the resolution. We can obtain the magnification of the electron microscope of a hundred thousand times the magnification of the microscope we normally use. There are many types of electron microscopes. You have to find the store of the type you want to buy.
How to Do a Test With a Microscope?
There are two methods to test how the microscope works:
Direct Observation
When you test a microscope, you have to place an object in the objective lens, which is the lens where we'll see the object. The objective lens is very big, so it is not possible to directly observe its function from the camera. You have to buy a microscope with a camera. If you have bought a microscope, you can test its performance by looking at an object through the objective lens.
In this way, you can test if you can correctly see the object you are going to use. It is easier to take pictures with a small lens. For example, it is easier to take a test with the 30X objective, and it is difficult to do a test with the 400X objective. It is better to test an objective lens that has a strong lens.
Test With an Object that You Already Have
If you already have an object, it is easy to test the working principle of a microscope. However, it is not easy to test the microscope's performance in this way. In this case, you have to find an object that has sharpness and contrast.
What is Sharpness?
If the object that you want to observe has sharpness, you can better observe its details. If there is no sharpness, you cannot observe the fine details of the object. There are many kinds of sharpness in the object, such as
Line (edge sharpness)
Curve (curve sharpness)
Point (dots sharpness)
There are also different types of sharpness in the observer's eyes, but this is not very important.
What is Contrast?
If the object that you want to observe has contrast, the edges of the object will be very clear and the objects can be distinguished. When you observe an object, it will be bright and dark, and we can judge whether the object is soft or hard. However, sometimes the object is not bright, and then it will be difficult to see the contrast.
How to test the Microscope?
You must try to find an object that has sharpness and contrast. If you do not find such an object, then you can buy one from a supermarket.
You can also refer to the specification of the microscope. First, make sure that you are familiar with how the microscope works. Second, make sure that you understand what a working principle means. If you are not familiar with a working principle, you must refer to the documentation. If you think it is appropriate, you can buy a kit or assemble the kit yourself. Third, we will learn how to use the microscope.
You must put a microscope slide (a white plate), a cover glass, a mirror, a camera lens, a light source, a tripod, a flashlight, a microscope, a micro-adjustment tool, a slide pen, and an adhesive tape in front of you.
Students often face trouble while conducting a travelling microscope experiment. Here, we will discuss the correct procedure to conduct this experiment, ensuring the best possible outcome.
However, before proceeding with the travelling microscope experiment class 12, let us learn some of the important factors necessary for the same.
Defining Refractive Index
Index of refraction, or refractive index is defined as the measure of the deviation of a light ray when it passes from one medium to another. In simpler terms, suppose you have a glass full of water. If you place it in sunlight, the light bends upon entering the water. If you measure the angle of such a bend, you will get its refractive index.
You can calculate a refractive index if the velocity of light c for a particular wavelength in empty space is known. Additionally, you must also know the value of ‘v’, which represents light’s velocity in a substance. In such a case, refractive index n = c/v
What is a Travelling Microscope?
Before you can use a travelling microscope experiment effectively, you must understand the functionality of such a device. Travelling microscopes act as simple microscopes, with one exception.
Where a simple microscope remains fixed for the duration of a study or experiment, a travelling microscope’s head is fitted onto a slider. Therefore, it can move along a scale, studying an object from various distances. Readings are taken by combining the readings from the Vernier and main scale.
Now, let us proceed to determine the refractive index of the glass slab using a travelling microscope.
Apparatus Necessary
Three glass slabs, each varying in thickness. Material for each slab must be identical.
Travelling microscope, and
Lycopodium powder
Theory for Refractive Index Experiment Report
Refractive Index (n) = Slab’s real thickness/slab’s apparent thickness
Procedure to Follow
To ensure accuracy in this refractive index of a glass slab using travelling microscope readings, follow the process mentioned below.
Step 1: Place a travelling microscope near a light source.
Step 2: Adjust screws to ensure that the base of this microscope is horizontal.
Step 3: Position the microscope horizontally, check the eyepiece to see whether the cross wires are visible clearly.
Step 4: Check the Vernier Constant of this scale when it is kept vertically.
Step 5: Use a marker to draw a mark at the microscope’s base. Consider this point as P.
Step 6: Now, focus the vertical microscope on point P in such a way that there is no chance of parallax between this image of P and the cross wires.
Step 7: Now, note the vernier scale, as well as the main scale reading. Consider this as R 1 .
Step 8: Place the thinnest glass slab on point P.
Step 9: Lift the microscope and focus the image of P 1 of the cross-mark.
Step 10: Make a note of the reading on the vertical scale (R 2 ).
Step 11: Sprinkle lycopodium powder on the slab.
Step 12: Lift the microscope further, focusing it on this particle near S.
Step 13: Make a note of R 3 on this vertical scale.
Step 14: Follow the same procedure to take readings of the other glass slabs.
Note down the results in a tabular format for increased ease of calculations.
Table for Readings
Serial Number | Vertical scale reading when it is focused on | Real Thickness | Apparent Thickness | Refractive Index | ||
R1 | R2 | R3 | ||||
1 | ||||||
2 | ||||||
3 |
Refractive Index Calculation = R3 – R1/R3 – R2
Mean Refractive Index = n1 + n2 + n3/3
Precaution- Ensure that you remove the parallax properly in step 6, failing which results of this travelling microscope experiment can be erroneous.
To know more about refractive index and experiments in general, consult our live online classes. Our experienced teachers guide you toward proper understanding with an expertly devised curriculum. Furthermore, now you can also download our Vedantu app for added convenience.
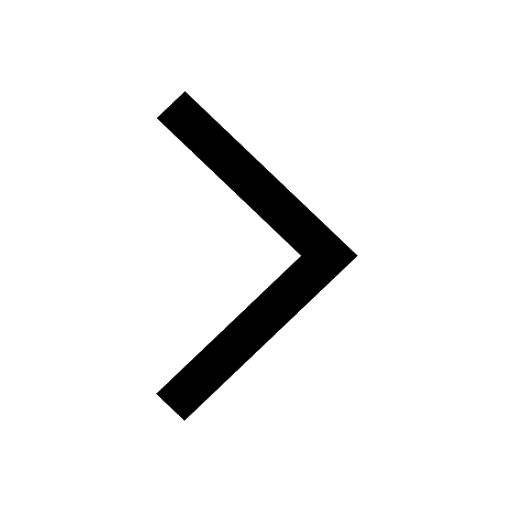
FAQs on Determine Refractive Index of a Glass Slab using a Travelling Microscope
1. How can I define a normal shift?
Normal shift is nothing but the difference between actual depth and apparent depth of an image.
2. What is the refractive index of a glass slab, which is hollow?
For such a glass, the refractive index or n is always 1.
3. What is the significance of lycopodium powder in this experiment?
Lycopodium powder ensures that the microscope’s focus is correct, and not at this bottom surface of this transparent slab.
4. Where can I find notes on the Refractive Index?
Students often face trouble while conducting a travelling microscope experiment. Here, we will discuss the correct procedure to conduct this experiment, ensuring the best possible outcome. Vedantu is a platform that aims at making students well prepared for the final exams and therefore it provides notes and answers to all the questions of previous year question papers obtained from expert teachers in the subject which can be downloaded either through the app or website.
5. Is physics tough?
Class 10 Physics consists of 10 interesting chapters which are all important in the field of physics as they lay the foundation for many diverse topics in further levels of education. Students panic due to the vast syllabus which results in stress and poor performance in exams. Preparing physics could be as easy yet interesting as playing your favorite game if the concepts are divided into small topics and experiments which could be practically done. Therefore physics isn’t tough but interesting provided proper attention is given.
NCERT Study Material
Stack Exchange Network
Stack Exchange network consists of 183 Q&A communities including Stack Overflow , the largest, most trusted online community for developers to learn, share their knowledge, and build their careers.
Q&A for work
Connect and share knowledge within a single location that is structured and easy to search.
How to measure the inner diameter of thin tube (0.5mm to 2mm)
Does anybody know what method or equipment could be used to measure the inner diameter of capillary tubes? They would be in the region of 0.5-2mm in diameter.
Edit: the tube is made of plastic and I have standard lab equipment available.
- experimental-physics
- measurements
- home-experiment

- $\begingroup$ If you are talking about metal capillary tubes, take a look at this article nvlpubs.nist.gov/nistpubs/jres/045/jresv45n4p283_A1b.pdf $\endgroup$ – J. Shupperd Commented Aug 27, 2016 at 15:44
- $\begingroup$ I have an idea, but for it to be a good one I need to know a few things: 1) What is the tube made of? 2) Would it be ok to get the tube wet? 3) How much length of tube do you have available? $\endgroup$ – DanielSank Commented Aug 27, 2016 at 20:26
- $\begingroup$ It would be helpful if you could tell us what material the tubes are made of, what length of tube you have available, and what other equipment you have. Micrometer, balance, accurate scale, microscope, ... What do you have? Is the tube transparent or not? Can you assume constant diameter? Do you know the material properties? Can your experiment destroy the tube? $\endgroup$ – Floris Commented Aug 28, 2016 at 12:38
4 Answers 4
The simplest method is probably to use a travelling microscope to measure the wall thickness at the ends of the tube, and vernier calliper or micrometer to measure outer diameter at a few points along the tube. The drawback is that this does not give an average value for wall thickness.
This article entitled "The Exact Measurement of Capillary Holes" describes a method using hydraulic resistance applying Poiseuille's Formula. The apparatus is a little complicated but fairly basic, and could probably be further simplified. It claims an accuracy of at least an order of magnitude better than the microscope method.
(Item #3 on page 1 of a Google Search "measure diameter capillary tube". The article cited in J Shupperd's answer was #1 in the same search. Hence my down-vote for lack of research effort.)

- $\begingroup$ That fourth-power of $r$ thing sure help, doesn't it? $\endgroup$ – dmckee --- ex-moderator kitten Commented Aug 27, 2016 at 23:58
- $\begingroup$ @dmckee : I didn't think about that when I posted this answer. It sounds like a good reason. $\endgroup$ – sammy gerbil Commented Aug 28, 2016 at 1:30
An easily applied lab technique would be to measure a length of tubing, and find its outer diameter with a micrometer, then weigh it. Knowing the density of stainless steel or glass (or whatever) completes the equation.
mass = density * length * (D_outer **2 - D_inner **2)* pi/4
- $\begingroup$ This depends very much on knowing the exact density of the plastic used. $\endgroup$ – sammy gerbil Commented Aug 28, 2016 at 21:37
- $\begingroup$ Yeah, even using a micrometer on a (possibly soft) plastic would make for some error bars. On the other hand, plastic parts aren't usually precise; I'd wonder, too, if the inner diameter is different when wet. $\endgroup$ – Whit3rd Commented Aug 29, 2016 at 4:04
I'm not a physicist so what I'm saying may be senseless. Since you used the tag home-experiment I thought of something you could do at home. What if you fill with a syringe containing water the tube until it's full? By knowing how long the tube is and how much water the syringe had initially you could calculate the diameter (I think at least). To block the possible backdraught you could pierce first a pencil eraser(the built-in to the pencil). And yes, you can find syringe needle smaller than 0.5mm.
Some reasonable methods have already been proposed, but you might just measure the height of water column in the capillary (at least if the capillary is transparent) and calculate the diameter ( https://en.wikipedia.org/wiki/Capillary_action#Height_of_a_meniscus )
- $\begingroup$ As long as you know the contact angle - which requires you to know the properties of the water and the surface VERY well. Also it is not stated whether these capillaries are transparent. $\endgroup$ – Floris Commented Aug 28, 2016 at 12:36
- $\begingroup$ @Floris: I agree with your comment. I would like just to note that I edited the answer to add the caveat on transparency (before you commented:-)) and that one can estimate the contact angle if the capillary is indeed transparent. $\endgroup$ – akhmeteli Commented Aug 28, 2016 at 12:48
Your Answer
Sign up or log in, post as a guest.
Required, but never shown
By clicking “Post Your Answer”, you agree to our terms of service and acknowledge you have read our privacy policy .
Not the answer you're looking for? Browse other questions tagged experimental-physics measurements home-experiment or ask your own question .
- Featured on Meta
- Announcing a change to the data-dump process
- Bringing clarity to status tag usage on meta sites
Hot Network Questions
- Can it be acceptable to take over CTRL + F shortcut in web app
- What does "if you ever get up this way" mean?
- Unable to upgrade from Ubuntu Server 22.04 to 24.04.1
- what should I do if my student has quarrel with my collaborator
- Marie-Sklodowska-Curie actions: publish a part of the proposal just after the deadline?
- What other marketable uses are there for Starship if Mars colonization falls through?
- Does it make sense for the governments of my world to genetically engineer soldiers?
- Does strong convergence of operators imply pointwise convergence of their unbounded inverses?
- How to translate the German word "Mitmenschlich(keit)"
- How many ways can you make change?
- Why is the stall speed of an aircraft a specific speed?
- In what instances are 3-D charts appropriate?
- How can coordinates be meaningless in General Relativity?
- How To "Fly" A Special Call Sign
- Did Gandalf know he was a Maia?
- Doesn't counting hole and electron current lead to double-counting of actual current?
- How to reconcile the effect of my time magic spell with my timeline
- Why does each state get two Senators?
- In Lord Rosse's 1845 drawing of M51, was the galaxy depicted in white or black?
- Sum of reciprocals of rough numbers
- How should I secure ceiling drywall with no edge backing?
- 5 deep Apex soql: System.QueryException: SOQL statements cannot query aggregate relationships more than 1 level away from the root entity object
- Avoiding USA "gambling tax"
- Is consciousness a prerequisite for knowledge?
- Open access
- Published: 04 September 2024
Anti- Toxoplasma gondii effects of XYP1-derived peptides and regulatory mechanisms of XYP1
- Jing Li 1 na1 ,
- Kaijuan Wu 1 na1 ,
- Xiaohua Liu 1 ,
- Dongqian Yang 1 ,
- Jing Xie 1 ,
- Yixiao Wang 1 ,
- Kang Liu 1 ,
- Zheng Wang 3 ,
- Wei Liu 4 &
- Liping Jiang 1 , 2
Parasites & Vectors volume 17 , Article number: 376 ( 2024 ) Cite this article
Metrics details
Toxoplasmosis, caused by Toxoplasma gondii , poses serious health issues for humans and animals. Individuals with impaired immune systems are more susceptible to severe toxoplasmosis. Pregnant women infected by T. gondii can face the possibility of birth defects and miscarriages. While pyrimethamine and sulfadiazine are commonly used drugs in clinical practice, concerns over their side effects and resistance are on the rise. A spider peptide XYP1 isolated from Lycosa coelestis had potent anti- T. gondii effects, but it had a high synthesis cost and strong cytotoxicity.
This study intended to modify XYP1 for producing derived peptides via amino acid truncation and substitution. The anti- T. gondii effect was evaluated by trypan blue staining assay and killing experiment of RH strain tachyzoites. The CCK8 and hemolysis assays were used to compare their safeties. The morphological changes of T. gondii were observed by scanning electron microscope and transmission electron microscope. In addition, the mechanism of XYP1 against T. gondii through RNA-sequencing was further explored.
In vivo and in vitro experiments revealed that XYP1-18 and XYP1-18-1 had excellent anti- T. gondii activity with lower cytotoxicity and hemolysis activity than XYP1. XYP1, XYP1-18, and XYP1-18-1 were able to disrupt the surface membrane integrity of T. gondii tachyzoites, forming pores and causing the disruption of organelles. Furthermore, RNA-sequencing analysis indicated that XYP1 could stimulate the host immune response to effectively eliminate T. gondii and lessen the host’s inflammatory reaction.
Conclusions
XYP1-18 had lower cytotoxicity and hemolysis activity than XYP1, as well as significantly extending the survival time of the mice. XYP1 played a role in host inflammation and immune responses, revealing its potential mechanism. Our research provided valuable insights into the development and application of peptide-based drugs, offering novel strategies and directions for treating toxoplasmosis.

Graphical Abstract
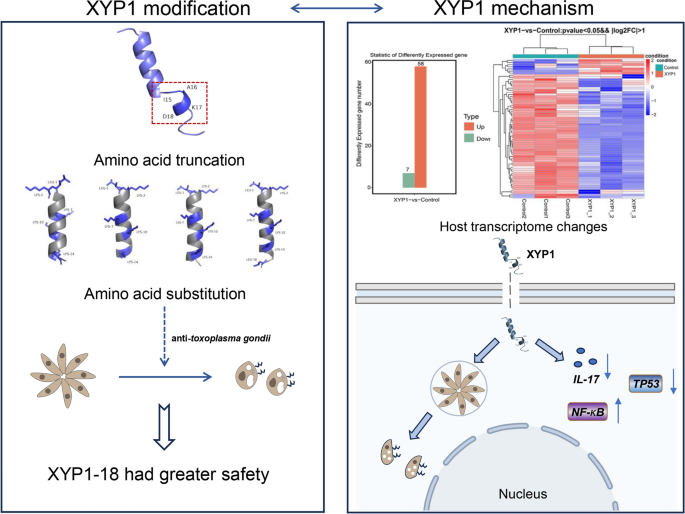
Toxoplasma gondii ( T. gondii ) is an important opportunistic pathogen belonging to the phylum Apicomplexa. T. gondii can cause toxoplasmosis, which is a zoonotic parasitic disease affecting both humans and animals [ 1 ]. Globally, approximately one-third of the population is estimated to be infected with T. gondii [ 1 , 2 ]. In immunocompromised patients, T. gondii infection can lead to significant morbidity and mortality. Currently, drugs such as pyrimethamine combined with sulfadiazine, trimethoprim combined with sulfamethoxazole, and spiramycin are mainly used in clinical treatment of toxoplasmosis [ 3 , 4 ]. While these treatments can alleviate symptoms and control the disease, the limitations and side effects of drug treatment need to be taken into account [ 5 ]. T. gondii also showed increasing resistance to sulfadiazine and pyrimethamine [ 6 ]. Therefore, it is crucial to explore new treatment methods and drugs for the effective management of toxoplasmosis.
In recent years, peptide drugs have emerged as a novel therapeutic approach with various applications such as antiinflammatory, antitumor, and antimicrobial properties, making them valuable in treating a wide range of diseases [ 7 , 8 , 9 ]. Peptides offer the advantage of specific targeting toward diseases while reducing the complexity of toxic side effects and drug metabolism, thus becoming a new focus in drug research for treating multiple diseases. Studies have identified peptides with anti- T. gondii activity. For instance, cal14.1a was a peptide extracted from Conus californicus , which can reduce the invasion and proliferation of T. gondii in host cells [ 10 ]. Additionally, longicin P4, an alkaline peptide extracted from Haemaphysalis longicornis , had shown efficacy in limiting the growth of T. gondii [ 11 ]. HPRP-A1 and HPRP-A2, α-helical cationic peptides extracted from Helicobacter pylori , can effectively reduce the survival rate of T. gondii tachyzoites and inhibit their adhesion and invasion of macrophages [ 12 ]. These indicated that peptides still hold great potential and application space in the fight against T. gondii infection.
Our earlier studies have indicated that a novel spider polypeptide XYP1 derived from the venom of Lycosa coelestis suppressed the invasion and proliferation of T. gondii and extended the survival time of mice infected with T. gondii [ 13 ]. Compared with the conventional drug sulfadiazine, the peptide XYP1 showed superior therapeutic efficacy and fewer side effects, thus holding significant promise for the treatment of T. gondii infection. However, limitations such as strong cytotoxicity and high synthesis cost restricted its clinical application.
Peptide truncation can not only save the synthesis cost, reduce reaction time, and enhance efficiency, but also can alter the bioactivity, stability, hydrophilicity, hydrophobicity, and toxicity of peptides. The beneficial effects of peptide truncation have been reflected in arasin 1 and linear chicken β-defensin-4 [ 14 , 15 , 16 ]. The distribution of hydrophobic and hydrophilic residues is a crucial factor influencing the activity of antimicrobial peptides (AMPs), with hydrophilic residues typically concentrated near the membrane surface of AMPs, while hydrophobic residues are distributed internally. This distribution pattern facilitates the interaction of AMPs with the cell membrane, so as to enhance their bactericidal activity [ 17 , 18 ].
In this study, XYP1 was truncated and replaced with amino acids to produce eight derived peptides. Two peptides, XYP1-18 and XYP1-18-1, with high anti- T. gondii activity were selected. XYP1-18 and XYP1-18-1 exhibited lower cytotoxicity and hemolysis compared with XYP1. Further in vivo and in vitro experiments indicated that XYP1-18 had similar activity as XYP1. The three peptides can damage the surface membrane and internal structure of T. gondii tachyzoites to different degrees. Additionally, we discovered that XYP1 can protect the host by reducing the inflammatory response and inducing immune response during T. gondii infection. In conclusion, our strategy may provide more insights and possibilities for peptide drug design and development, offering a novel approach for utilizing peptides to combat T. gondii infection in the future.
Parasites and cell culture
Tachyzoites of the RH-GFP-TgAtg8 and RH wild-type T. gondii strains were transmitted in human foreskin fibroblasts (HFFs). HFFs were growth in Dulbecco’s modified Eagle’s medium (DMEM, Gibco, USA) attached with 1% antibiotics (10 mg/mL streptomycin solution, 25 μg/mL amphotericin B, and 10,000 U/mL penicillin) (Sangon Biotech, China) and 10% fetal bovine serum (FBS, Invitrogen, USA) at 37 ℃ with the concentration of 5% CO 2. The different T. gondii strains were maintained in HFFs with DMEM, 2% FBS, and 1% antibiotics in the same environment [ 19 ].
The 6–8-week-old female BALB/c and Kunming mice used in this study were purchased from the Department of Laboratory Animals, Central South University in China.
Sequence and structure analysis of polypeptides
The various characteristics of polypeptides including isoelectric point, net charge, and average hydrophilicity were analyzed on the website ( https://www.expasy.org/ ) [ 20 ]. The tertiary structure of polypeptides was predicted by I-TASSER's online tool ( http://zhanglab.ccmb.med.umich.edu/I-TASSER/ ) [ 21 ]. The spiral round figure was constructed by Heliquest online website ( http://heliquest.ipmc.cnrs.fr/ ) [ 22 ].
Chemical synthesis and identification of XYP1-derived peptides
The derived peptides were synthesized by Fmoc solid phase polypeptide synthesis method [ 23 ]. It mainly included the following steps: deprotection of Fmoc group, coupling reaction, washing, detection using the Kaiser method, and cleavage. High performance liquid chromatography (HPLC) technique (Shimadzu, Japan) was used to purify peptides, and electrospray ionization mass spectrometry (ESI–MS) (Shimadzu, Japan) was used to identification of derived peptides.
Evaluation of anti -toxoplasma gondii effect
When T. gondii died, trypan blue was able to cross its cell membrane and enter the interior, making it blue. Trypan blue exclusion test was undertaken as mentioned before [ 24 ]. Firstly, 10 μM different XYP1-derived peptides were prepared, incubated with T. gondii for 2 h, and then the trypan blue dye was added. After 3–5 min, the survival rate of T. gondii was observed by optical microscope and the mortality rate was calculated.
The tachyzoites of RH-GFP-TgAtg8 strain did not fluorescein after death, which can be used to determine the difference in the anti- T. gondii activity of XYP1 and its derived peptides; 10 μM different derived peptides were incubated with tachyzoites of RH-GFP-TgAtg8 strain, respectively, and the fluorescence intensity and area were observed under fluorescence microscope (Leica DM IL LED, Germany).
Hemolysis and cytotoxicity assays
The hemolytic activity of peptides was determined by the amount of hemoglobin released from lysed human red blood cells [ 25 ]. Normal human blood was mixed 1:1 with Aldrin’s fluid, followed by centrifuging, and phosphate buffered saline (PBS) was added to make 1% red blood cell resuspension. The 50 μL resuspension was mixed with 50 μL PBS, 1% Triton X-100, 70% DMSO (Sigma, USA), and different concentrations of polypeptides, then incubated at 37 ℃ for 30 min. After centrifugation, the OD 540 value of the supernatant was measured by Microplate reader (Agilent, USA) and the hemolysis rate was calculated.
The CCK-8 method [ 26 ] was employed to assess the effect of different peptides on cytotoxicity, and 100 μL of cell culture solution containing 10 μM different peptides were added to each of the 96-well plates. After 24 h, 10 μL CCK-8 solution (APExBIO, USA) was added and incubated for another 2 h. The OD 540 value was then measured and the cell mortality was calculated.
Survival assay
The anti- T. gondii effect of peptides in vivo was investigated through a mouse survival experiment [ 27 ]. The experiment was divided into a PBS control group and different polypeptide groups. Each group of mice was intraperitoneally injected with 1000 RH strain tachyzoites. After 4 h, the pre-prepared 4 mg/mL peptides were injected into the mice. The survival time of mice in each group was recorded and analyzed statistically.
Electron microscopy technology
The scanning electron microscope (SEM) was used to observe the surface structure of T. gondii , while the transmission electron microscope (TEM) can reflect the morphological changes of T. gondii inside the cell [ 28 ]. The sample preparation process for SEM included: the preparation process of SEM consists of fixing the T. gondii sample with 4% glutaraldehyde solution and staying overnight at 4 ℃. A series of gradient dehydration treatments are performed, followed by dehydration in anhydrous ethanol. After dehydration, the tachyzoites were dried and coated, followed by SEM observation (Hitachi S-3400N, Japan).
TEM steps mainly include fixing, rinsing, dehydration, infiltration, embedding, etc. After the T. gondii samples were fixed in 4% glutaraldehyde, they were washed with a cacodylate buffer, and 1% osmium tetroxide was used to fix the sample at 4 ℃ for 2 h. The fixed samples were then washed and subjected to a series of gradient dewatering treatments before being embedded in epoxy resin and polymerized at 60 ℃. After polymerization, the sample was sliced and double stained. Finally, the internal structure of T. gondii tachyzoites treated with different polypeptides was observed by TEM (Tecnai G2 Spirit TWIN, USA).
RNA-seq analysis
The control group consisted of HFF cells infected with T. gondii without XYP1, and the experimental group was treated with XYP1 for 8 h. Total RNAs were extracted from the control and experimental groups and reverse to cDNA, with three replicates per group. After the steps of end repair, A-tailing, and adapter ligation, the samples were proceeded with polymerase chain reaction (PCR) amplification and Illumina transcriptome sequencing in the OE biotech Co., Ltd. (Shanghai, China). Trimmomatic software [ 29 ] was used to filter the raw data to obtain high-quality data information. Subsequently, the filtered data was aligned and assembled using HISAT2 [ 30 ] and StringTie [ 31 ] tool. The number of reads mapped to each gene in each sample was calculated.
DESeq software [ 31 ] was used to screen differentially expressed genes, and the screening conditions for differential genes were fold change > 2 and P value < 0.05. To further understand the function of differentially expressed genes, cluster analysis, gene ontology (GO) functional enrichment analysis [ 32 ] and Kyoto Encyclopedia of Genes and Genomes (KEGG) pathway enrichment analysis [ 33 ] were conducted on the selected genes. Additionally, the PPI network of differentially expressed proteins was constructed by the STRING website, and hub genes were identified by Cytoscape software.
Quantitative real-time PCR (qRT-PCR)
The total RNAs from different samples were extracted using the classical Trizol method. The PerfectStart Uni RT&qPCR Kit (TransGen Biotech, China) was used to efficiently reverse transcribe RNA into cDNA and synthesize the first strand. The qRT-PCR reaction system contains cDNA temple, forward and reverse primers, double stranded DNA fluorescent dye (SYBR Green I), DNA polymerase, dNTP and buffer. The PCR reaction was performed using a two-step amplification procedure: 94 ℃ for 30 s (pre-denaturation) followed by 40 cycles of 94 ℃ for 5 s and 60 ℃ for 30 s. Finally, the relative expression of each gene was calculated by 2 −∆∆Cq method.
Statistical analysis
The quantitative data was analyzed with the method of two-tailed Student’s t -test or two-way analysis of variance (ANOVA) with Tukey’s multiple comparisons in GraphPad 8.4 software. A P -value < 0.05 was considered to have a significant difference.
Sequence analysis and structural modification of XYP1
XYP1, as a novel anti- T. gondii polypeptide, showed good anti- T. gondii activity [ 13 ]. To further reduce the cytotoxicity and synthetic cost of XYP1, bioinformatic analyses and structural modifications were performed to optimize the structure and function of XYP1. On the basis of the predicted tertiary structure of XYP1 (KIKWFKAMKSIAKFIAKDQLKKHL, Fig. 1 A), the α-helix structure of the N-terminal was retained and truncated from the C-terminal, resulting in four truncated peptides: XYP1-15, XYP1-16, XYP1-17, and XYP1-18 (Fig. 1 ). The structure with hydrophobic and hydrophilic residues distributed on both sides is beneficial for enhancing the activity of antimicrobial peptides (AMPs). Therefore, according to the helical wheel projection diagrams of the truncated peptides, the amino acid substitutions were performed on the four peptides. Specifically, leucine (Leu, L) instead of lysine (Lys, K), K instead of isoleucine (Ile, I), K instead of alanine (Ala, A), serine (Ser, S) was replaced with K, phenylalanine (Phe, F) was replaced with lysine (K), and aspartic acid (Asp, D) was replaced with leucine (L). As shown in Fig. 1 , four peptides were eventually generated: XYP1-15-1 (LKKWFKKMKKIAKKI), XYP1-16-1 (LKKWFKKMKKIAKKIA), XYP1-17-1 (LKKWFKKMKKIAKKIAK), and XYP1-18-1 (LKKWFKKMKKIAKKIAKL). The properties and parameters of XYP1 and its derived peptides were shown in Additional file 1 : Table S1. The high-purity polypeptides were successfully synthesized by solid-phase synthesis. The results of ESI–MS (Additional file 1 : Figs. S1 and S2) showed that the actual molecular weight of each polypeptide was consistent with the theoretical molecular weight.
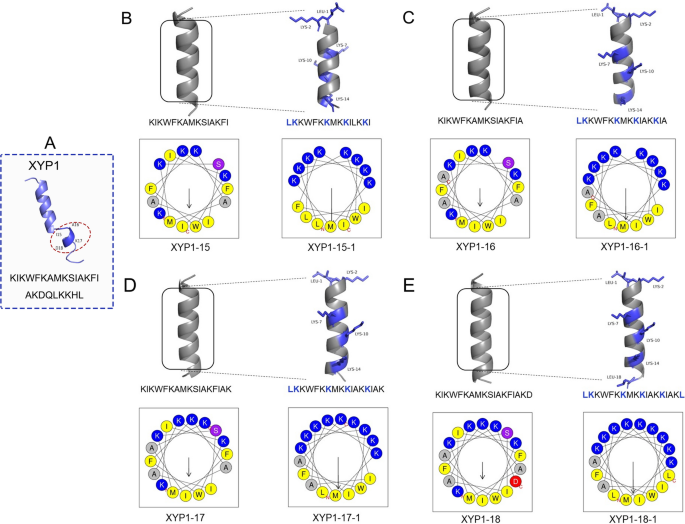
Structure diagram of XYP1 and its derived peptides. A The predicted tertiary structure diagram of XYP1, the dotted red boxes are the amino acids intended to be truncated. B The whole diagram is divided into four small pictures, with the left being the structure diagram and spiral wheel diagram of XYP1-15, and the right being the schematic diagram of XYP1-15-1; the residues after amino acid replacement of XYP1-15 are marked in blue. C The left diagram is the structure diagram and spiral wheel diagram of XYP1-16, and the right diagram exhibits XYP1-16-1; the residues after amino acid replacement of XYP1-16 are marked in blue. D The diagram on the left describes the structure and properties of XYP1-17, and the right diagram represents XYP1-17-1; blue marks the amino acids that have been replaced. E The figures on the left and right show the structure and properties of the derived peptides XYP1-18 and XYP1-18-1, respectively
The derived peptides XYP1-17, XYP1-18, and XYP1-18-1 exhibited anti- T. gondii activity
Our previous study has confirmed that 10 μM XYP1 can kill T. gondii tachyzoites. Therefore, trypan blue staining assay was used to compare the killing activity in vitro of 10 μM different derived peptides and XYP1. The result of XYP1 was consistent with the previous findings. Among these derived peptides, XYP1-17, XYP1-18, and XYP1-18-1 also exhibited good anti- T. gondii activity, with XYP1-18 being comparable to XYP1 (Fig. 2 A). To further demonstrate the anti- T. gondii activity of XYP1-17, XYP1-17-1, XYP1-18, and XYP1-18-1, the tachyzoites of RH-GFP-TgAtg8 T. gondii strain were co-incubated with 10 μM concentrations of these peptides. After a 2-h incubation, we observed their anti- T. gondii activity through fluorescence microscopy (Fig. 2 B). The fluorescence area and intensity of XYP1-17, XYP1-18, and XYP1-18-1 groups were significantly reduced compared with the control group (Fig. 2 C, D ). These results were consistent with the trypan blue staining assay.
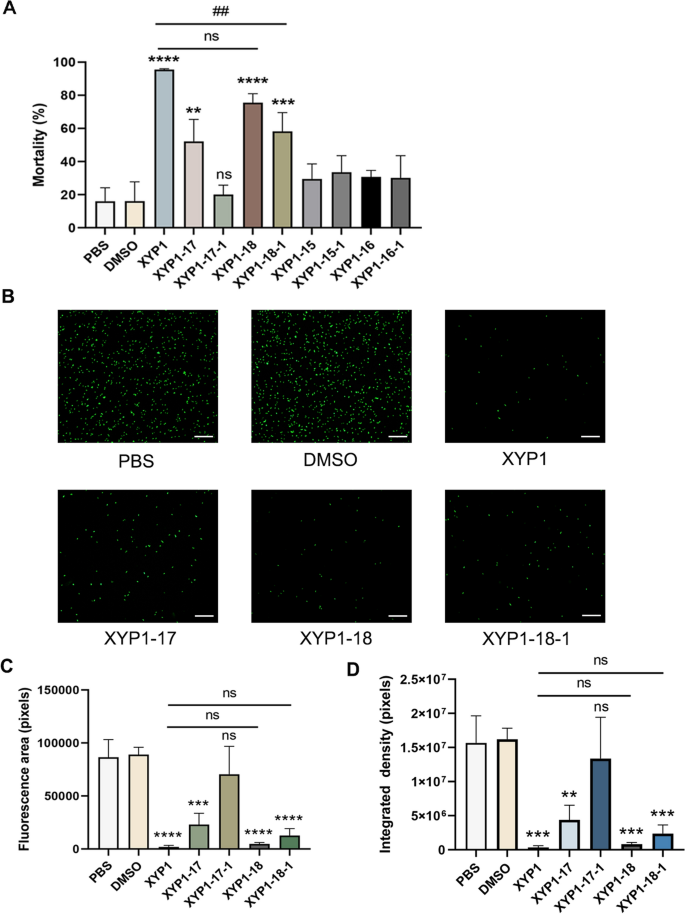
Inhibitory effect of XYP1 and its derived peptides on T. gondii in vitro. A Statistical diagram of toxoplasma mortality in each group in trypan blue experiment, the killing effect of PBS (negative control group), DMSO (negative control group), and XYP1 and its derived peptides (experimental group) on T. gondii at a concentration of 10 μM. * P < 0.05, ** P < 0.01, ## P < 0.01, *** P < 0.001, **** P < 0.0001, “ns” shows P > 0.05. B Fluorescence microscopy results of control group and XYP1 and derived peptides (XYP1-17, XYP1-18, XYP1-18-1), scale = 100 μm. C–D Statistical analysis of fluorescence area and fluorescence intensity of each group, * P < 0.05, ** P < 0.01, *** P < 0.001, **** P < 0.0001, “ns” shows P > 0.05
To explore the concentration dependence of the polypeptides against T. gondii , XYP1-derived peptide solutions with different concentration gradients (5 μM, 10 μM, 20 μM) were prepared and then mixed with T. gondii tachyzoites at room temperature. After incubation for 2 h, the anti- T. gondii effect was observed by fluorescence microscopy. The results showed that the killing effect of these three peptides on tachyzoites of RH-GFP-TgAtg8 strain was enhanced with increasing concentration (Fig. 3 A), and the fluorescence density of T. gondii became weaker (Fig. 3 B). Compared with the control group, the fluorescence intensity and fluorescence area of XYP1 and XYP1-18 at different concentrations were statistically different.

Safety assessment and survival experiments of XYP1-derived peptides. A , B The killing effect of derived peptides on T. gondii at different concentrations; Fig. A is the statistical analysis of fluorescence area of each group, Fig. B is the statistical analysis of fluorescence intensity of each group. * P < 0.05, ** P < 0.01, *** P < 0.001, **** P < 0.0001, “ns” shows P > 0.05. C Cytotoxic effects of XYP1, XYP1-18, and XYP1-18-1 at different concentrations on HFFs; each graph shows the IC 50 value of each polypeptide to the HFFs. D Hemolytic activity of XYP1, XYP1-18, and XYP1-18-1 polypeptides on human red blood cells, hemolysis rate is a measure of hemolytic activity. E Survival of mice infected with T. gondii after treatment with three peptides: XYP1, XYP1-18, and XYP1-18-1, all drugs were administered to mice at a dose of 4 mg/kg, * P < 0.05, ** P < 0.01, *** P < 0.001, **** P < 0.0001, “ns” shows P > 0.05
The security of XYP1-18 and XYP1-18-1 was higher than XYP1
According to the results of trypan blue staining and fluorescence microscopy, the peptides XYP1-18 and XYP1-18-1 had better anti- T. gondii effects. Therefore, these two peptides were selected for the cytotoxicity test. It was observed that the higher the concentration of XYP1, XYP1-18, and XYP1-18-1, the greater the toxicity to cells (Fig. 3 C). The IC 50 values of XYP1, XYP1-18, and XYP1-18-1 were 33.63 μM, 80.60 μM, and 45.60 μM, respectively. The IC 50 values of XYP1-18 and XYP1-18-1 were higher than XYP1, indicating higher safety of these two derived peptides.
Hemolysis assay was also used to evaluate the toxic effects of peptides on the host. When XYP1 concentrations ranged from 1.25 μM to 10 μM, almost no hemolysis reaction was observed. However, as the concentration increased, the hemolysis rate gradually increased (Fig. 3 D). In contrast, XYP1-18 caused almost no hemolysis at concentrations ranging from 1.25 μM to 80 μM, and only minor hemolysis occurred even at the highest concentration (160 μM). XYP1-18-1 had low hemolysis at 1.25 μM to 160 μM, with a maximum hemolysis rate of only 20% (Fig. 3 D). It was indicated that XYP1-18 and XYP1-18-1 were safer than XYP1 over a wider range of concentrations.
The efficacy of XYP1-18 in vivo was comparable to XYP1
The survival experiment was used to evaluate the therapeutic efficacy of several peptides against T. gondii infection in vivo. All mice in the control group died at 127.1 h. In contrast, XYP1 can significantly prolong the survival of mice to 208.5 h, XYP1-18 can be extended to 191 h, and XYP1-18-1 can only be extended to 145 h (Fig. 3 E). There is no significant difference between XYP1-18 and XYP1. XYP1-18-1 group had poor effect on prolonging the survival time of mice.
XYP1-18 and XYP1-18-1 can destroy the surface structure and organelles of T. gondii
The effects of XYP1, XYP1-18, and XYP1-18-1 on the surface structure of T. gondii tachyzoites were observed using SEM. Normal T. gondii tachyzoites were crescent-shaped, with a sharp front end and a rounded back end (Fig. 4 A, B ). The cell membrane was complete and there were many micropores on the surface. After being treated with 10 μM polypeptides for 2 h, various damages were observed on the surface membranes of tachyzoites. In the XYP1 group, pores formed on the cell membrane surface, and many tachyzoites occurred wrinkling or expansion of the membrane (Fig. 4 C, D ). Under the influence of XYP1-18, many tachyzoites disintegrated, with depressions appearing on the cell membrane surface (Fig. 4 E, F ). XYP1-18-1 caused the pores or depressions on the surface of the T. gondii cell membranes, and some tachyzoites disintegrated (Fig. 4 G, H ). These results indicated that the three peptides had varying degrees of impact on the surface morphology of T. gondii . Specifically, XYP1-18 and XYP1-18-1 had more pronounced destructive effects on T. gondii than XYP1.
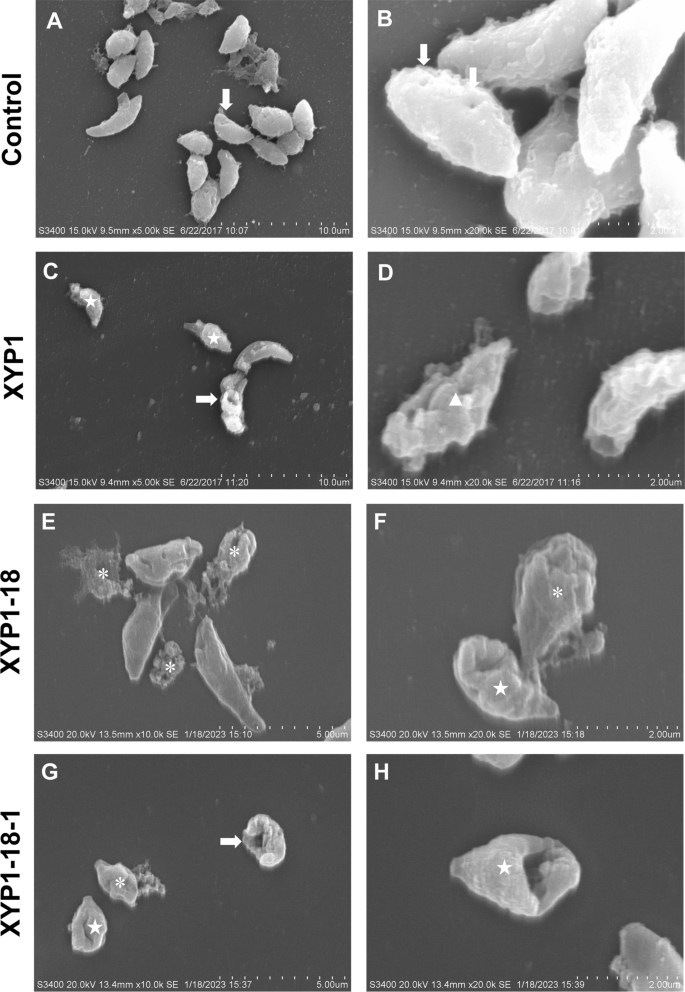
The SEM results of XYP1, XYP1-18, and XYP1-18-1 effects on the surface structure of T. gondii tachyzoites. A , B T. gondii tachyzoites were incubated with PBS buffer for 2 h. C , D T. gondii tachyzoites were incubated with XYP1 (10 μM) solution for 2 h. E , F T. gondii tachyzoites were incubated with XYP1-18 (10 μM) solution for 2 h. G , H T. gondii tachyzoites were incubated with XYP1-18-1 (10 μM) solution for 2 h. ↓: micropore; → : holes; ☆ : shrunken or sunken tachyzoites of T. gondii ; *: disintegrating T. gondii tachyzoites; enlarged tachyzoite. Scale: A = 10 μm; B = 2 μm; C = 10 μm; D = 2 μm; E = 5 μm; F = 2 μm; G = 5 μm; H = 2 μm
TEM was used to observe the organelle structure inside T. gondii . The organelles inside the untreated tachyzoites were intact (Fig. 5 A, B ). In the XYP1 group, the tachyzoites not only showed ruptures in the surface membrane, but also different degrees of vacuolization internally (Fig. 5 C, D ). With the treatment of XYP1-18, the majority of tachyzoites exhibited not only severe deformation and rupture of the surface membrane, but also serious shrinkage and vacuolization internally (Fig. 5 E, F ). In the XYP1-18-1 group, the internal structure of tachyzoites was even more severely damaged, with not only shrinkage and vacuolization, but also complete dissolution of the tachyzoites observed (Fig. 5 G, H ). In conclusion, these polypeptides had varying degrees of impact on the internal structure of T. gondii tachyzoites.
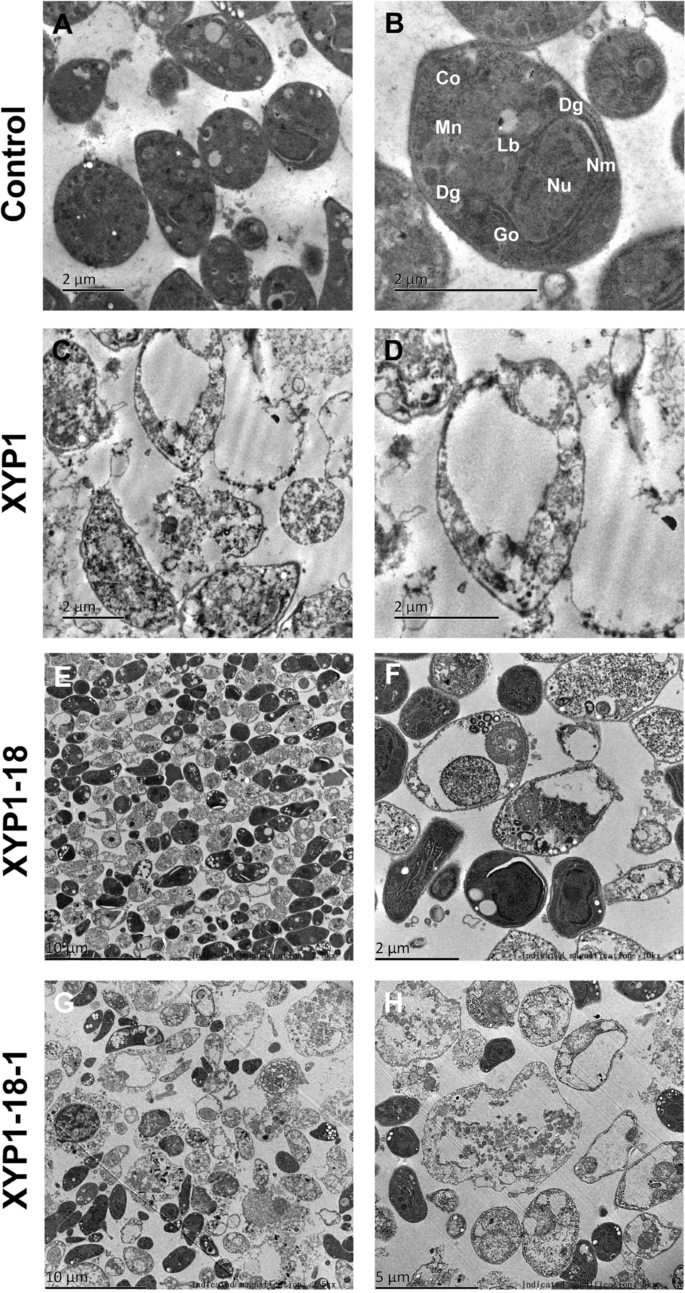
The TEM results of XYP1, XYP1-18, and XYP1-18-1 effects on the internal structure of tachyzoites of T. gondii. A , B T. gondii tachyzoites were incubated with PBS buffer for 2 h. C , D T. gondii tachyzoites were incubated with XYP1 (10 μM) solution for 2 h. E , F T. gondii tachyzoites were incubated with XYP1-18 (10 μM) solution for 2 h. G , H T. gondii tachyzoites were incubated with XYP1-18-1 (10 μM) solution for 2 h. Co: conoid; Dg: electron-dense granule; Go: Golgi complex; Lb: lipid body; Nu: nucleus; Nm: nuclear membrane. Scale: A = 2 μm; B = 2 μm; C = 2 μm; D = 2 μm; E = 10 μm; F = 2 μm; G = 10 μm; H = 5 μm
XYP1 affected the inflammatory and immune responses of host cells
Previous studies have initially explored the effects of XYP1 on T. gondii -related genes and pathways. To better understand the host cell response to T. gondii under the influence of XYP1, changes in genes and pathways before and after XYP1 treatment were analyzed by transcriptome technology. P < 0.05 and fold change > 2 were used to determine whether genes in the control group and the XYP1 group showed differential expression. A total of 65 genes had significant differences in expression, including 7 up-regulated genes and 58 down-regulated genes (Fig. 6 A). The M-versus-A (MA) map and volcano map represented significantly differential genes under differential screening conditions (Fig. 6 B, Additional file 1 : Fig. S3A). After clustering analysis of the differentially expressed genes (Fig. 6 C), it was observed that the samples from the control group and the XYP1 group clustered together in the same cluster, indicating that these genes exhibited similar expression patterns in the two sample groups and may have similar biological functions.
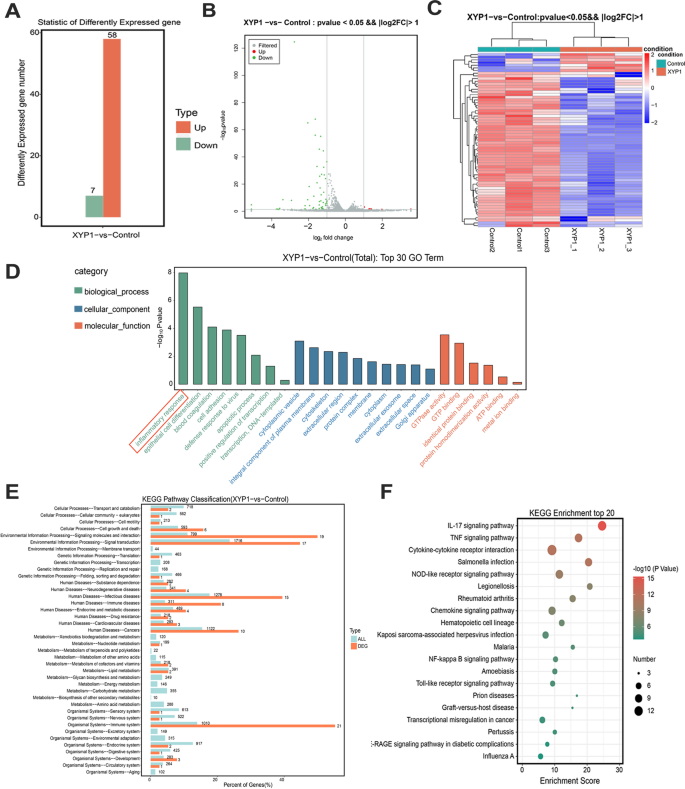
Effects of XYP1 on genes and pathways associated with host cells infected with T. gondii. A The number of differential genes up-regulated and down-regulated. B In the differentially expressed volcano map, green and red dots represent significantly different genes, and gray dots represent nonsignificantly different genes. C The results of cluster analysis for each difference group. Red indicates highly expressed genes, blue indicates genes that are low expression. D GO enrichment analysis Top30 bar chart. The horizontal coordinate is the GO entry name and the vertical coordinate is −log 10 P -value. E Distribution of differentially expressed genes and all genes in KEGG pathway. The horizontal axis is the ratio (%) between the genes annotated to each metabolic pathway and the total number of genes annotated to the KEGG pathway. F Bubble diagram of KEGG enrichment Top20, entries with larger bubbles contain more differential protein coding genes, and the redder the bubble color, the greater the difference
The 37 differentially expressed genes were classified into 3 main gene ontology (GO) categories, which were further assigned to 64 specific functional subcategories. As shown in Additional file 1 : Fig. S3B, a total of 448 significantly enriched GO terms were identified. Among them, 310 terms were enriched in biological process (BP), 65 terms in cellular component (CC), and 73 terms in molecular function (MF). In the top 30 GO terms (Fig. 6 D), the most significantly enriched term in BP was “inflammatory response,” in CC it was “vesicle,” and in MF it was “GTPase activity.” The KEGG enrichment results were summarized in Fig. 6 E. Immune-related pathways with a higher gene enrichment included the IL-17 signaling pathway, tumor necrosis factor signaling pathway, cytokine-cytokine receptor interaction, NOD(Nucleotide oligomerization domain)-like receptor signaling pathway, and NF-kappa B signaling pathway. The top 20 significantly enriched pathways were selected from the KEGG enrichment results for display in a bubble chart, as shown in Fig. 6 F.
Furthermore, protein–protein interaction (PPI) networks were mapped and the core interaction networks were predicted. As shown in Fig. 7 A, a darker green color indicates higher score of the nodes. MCC, DMNC, and radiality algorithms are used to screen the Top20 hub proteins (Fig. 7 B–D). The redder the color is, the higher the score of the nodes, and the more yellow–green the color is, the lower the score. In the MCC algorithm, the top three proteins were IL1B, IL-6, and CXCL-8; in the DMNC algorithm, the top three proteins were CCL-8, CSF3, and CXCL3; in the radiality algorithm, the top three proteins were IL1B, IL-6, and CXCL-8, consistent with the MCC results. Subsequently, the intersection was taken and a Venn diagram was plotted (Fig. 7 E), showing 19 common hub proteins (Additional file 1 : Table S2). MMC and radiality shared IL1B. There is ENTPD1 in the DMNC algorithm that has no intersection with the other two algorithms. The results of qPCR showed that the transcription levels of IL-17 and TP53 were down-regulated and NF-kB was up-regulated in the XYP1 treatment group (Fig. 7 F–H).
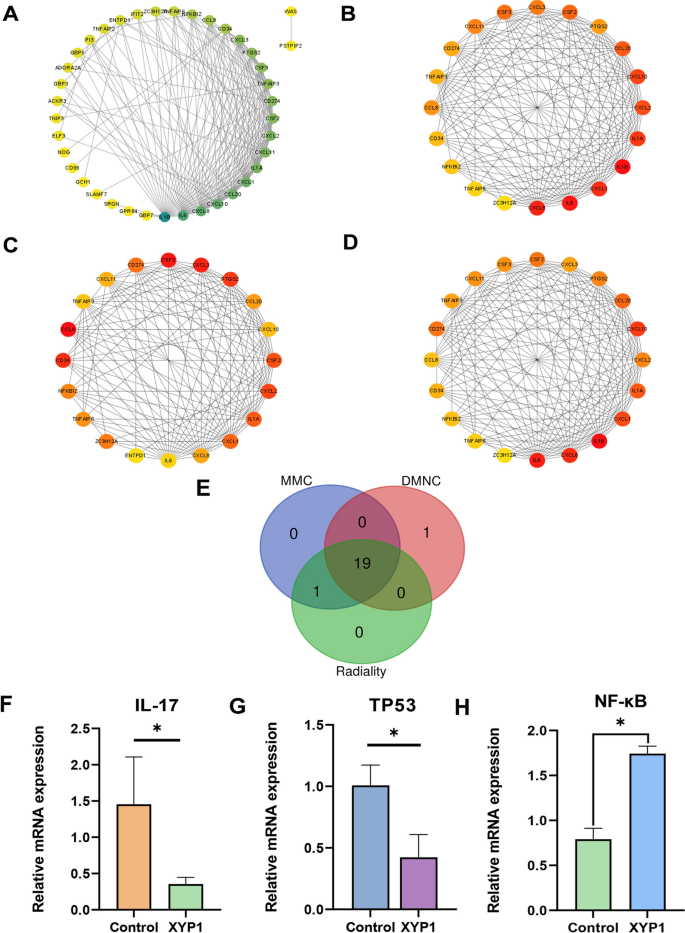
Differential expression protein interaction network analysis and qPCR validation. A Circular protein interaction network map of differentially expressed genes, the darker the green color, the higher the node score. B The top 20 hub genes based on MMC algorithm. C The top 20 hub genes based on DMNC algorithm. D The top 20 hub genes based on radiality algorithm. E Venn diagram of hub genes intersection of three algorithms. F – H Changes in transcription levels of IL-17 , tp53 , and NF-κB genes
When T. gondii infects humans, it often remains inapparent, but those with congenital infections or compromised immune systems may suffer severe symptoms [ 34 , 35 ]. The development of novel drugs is a current research focus, and venom peptides derived from animal toxins hold great potential as scaffolds for clinical applications, including cysteine-stabilized peptides and linear helical peptides [ 36 ]. In this study, eight derived peptides were successfully generated by amino acid truncation and replacement of XYP1, which originates from the venom of Lycosa coelestis . Preliminary results suggested that XYP1 might exert anti- T. gondii effect on the basis of an α-helical structure [ 13 ]. Phospholipids serve as a major source of negative charge in cell membranes, and active peptides with an α-helical structure often target phospholipids [ 37 ]. Therefore, the α-helix structure of the derived peptide is preserved. Because these peptides contain positively charged amino acid side chains, they can interact with phospholipids on the cell membrane by electrostatic interaction. The amphipathic α-helical structure formed by the peptides facilitates their insertion into the cell membrane [ 38 ]. When the peptide inserts into the cell membrane, its hydrophobic regions interact with the phospholipid bilayer, disrupting the integrity of the cell membrane, which leads to the formation of pores and gaps. These pores and gaps facilitate the leakage of cellular contents, thereby disrupting cellular homeostasis [ 39 , 40 ].
Another essential property of AMPs is amphipathy, which refers to the relative abundance of hydrophilic and hydrophobic residues. Studies have shown that indolicidin analogs with increased amphipathy and charge displayed lower hemolytic activity and maintained antimicrobial activity [ 41 , 42 ]. The distribution of hydrophobic and hydrophilic residues on both sides of the polypeptide plays a crucial role in the biological activity and bioavailability of AMPs [ 17 ]. By replacing individual amino acid residues, we successfully generated four new peptides that were structurally similar to truncated peptides but had more evenly distributed hydrophilic and hydrophobic residues, which may enhance their biological activity.
The initial screening experiments revealed that three peptides, XYP1-17, XYP1-18, and XYP1-18-1, exhibited much strong anti- T. gondii effects than the control group. Among them, XYP1-18 had similar anti- T. gondii activity to XYP1, while the other peptides had minimal effects. The lack of efficiency in XYP1-15 and XYP1-16 could be attributed to the absence of key functional residues induced by a greater number of truncated amino acids. Cationic amino acids, such as lysine (Lys) or arginine (Arg), are crucial for antimicrobial activity in peptide sequences. These common cationic amino acids are typically positioned on the hydrophilic side of the antimicrobial peptides [ 43 , 44 , 45 ]. There is a complex interaction among net charge, hydrophobicity, and amphipathicity [ 46 , 47 , 48 , 49 ]. These parameters play different roles in various peptide sequences, with optimal antimicrobial activity requiring the right balance of hydrophobicity, amphipathicity, and charge density. The truncated XYP1-15 and XYP1-16 significantly increased hydrophobicity and decreased net charge, while excessive hydrophobicity led to increased cytotoxicity and loss of activity, and decreased net charge may lead to decreased activity. Although the amphiphilicity and net charge of XYP1-15-1 and XYP1-16-1 increased, their hydrophobicity decreased, which may affect their anti- T. gondii activity.
The main difference between XYP1-17-1 and XYP1-18-1 were the lack of a leucine residue. Typically, higher hydrophobic leucine (Leu, L) can be substituted for lower hydrophobic amino acid residues to compensate for this loss [ 50 , 51 , 52 ]. Leucine side chains are relatively large, containing an isopropyl group that contributes to hydrophobic effects. These effects are crucial in protein folding and maintaining the structure of α-helices [ 53 ]. Further in vitro screening experiments had shown that XYP1-18 and XYP1-18-1 were the most effective derived-peptides, with lower cytotoxicity and hemolytic activity than XYP1, thus they have better potential as candidates for polypeptide therapy in toxoplasmosis.
We previously discovered that XYP1 can significantly down-regulate the MIC10 , HSP29 , and rpb-10 genes in T. gondii , which was associated with membrane component, invasion, and proliferation of T. gondii . However, it remains unclear how XYP1 affects the interactions between T. gondii and the host. In this study, RNA-seq was used to focus on the differential genes in the host before and after XYP1 treatment. In total, 65 genes showed significant differences in expression, with 7 up-regulated (10.77%) and 58 down-regulated (89.23%) genes. According to GO analysis, the most enriched biological process is the inflammatory response. Genes involved in this process include ADORA2A , NFKBIZ , ELF3 , and TNIP3 . This suggested that XYP1 may eliminate T. gondii by modulating the host cell’s immune system to trigger inflammatory response and immune response. When host cells were infected with T. gondii , it activated the immune system, leading to the release of inflammatory cytokines such as IL-6, IL-1β, IL-12, and TNF-α. These cytokines promote inflammation, activating the host cell’s immune function to combat T. gondii [ 54 , 55 , 56 , 57 , 58 ]. The NF-κB is a driver of inflammation response, and NFKBIZ is an inhibitor of NF-κB [ 59 ]. Our findings revealed that XYP1 may kill T. gondii by up-regulating the NF-κB regulated pathway. Additionally, on the basis of KEGG analysis, we found that the most significantly enriched pathways among down-regulated genes were the IL-17 signaling pathway, cytokine–cytokine receptor interaction, and TNF signaling pathway. The down-regulation of IL-17 was verified by qRT-PCR assay (Fig. 7 F). IL-17 production was associated with protective and pathogenic responses during T. gondii infection [ 60 ]. Thus, XYP1 might down-regulate the host cell IL-17 signaling pathway, reducing host pathological responses. These pathways were crucial for regulating various biological processes via cell-to-cell interactions and signals.
To sum up, the study of XYP1-derived peptides provided a promising treatment approach for T. gondii infection, with great potential for clinical application. While considerable progress has been made, there remain hurdles to overcome. For example, the use of peptide drugs has limitations such as a short half-life. Therefore, future research should focus on improving the properties and effectiveness of peptides to enhance their clinical value. Additionally, further investigation is needed to explore how XYP1 specifically regulates the immune response in host cells to better understand its anti- T. gondii mechanisms. In summary, this study lays the groundwork for the development of new anti- T. gondii drugs and offers important insights for the research and usage of peptide-based drugs.
This study successfully identified two derived peptides, XYP1-18 and XYP1-18-1, with anti- T. gondii activity through modifying XYP1. In comparison with the parent XYP1, these peptides exhibited lower cost and improved safety (low cytotoxicity and hemolytic activity). Survival assay results demonstrated that XYP1-18-1 also can prolong the survival time of T. gondii -infected mice. Furthermore, XYP1 can eliminate T. gondii by modulating the inflammatory and immune response of host cells. These discoveries provided a novel strategy and direction for treating T. gondii infection, as well as useful references for the development and application of peptide-based therapies.
Availability of data and materials
The HPLC and ESI–MS results of peptides generated during the current study is stored in the Figshare repository, ***:. The RNA-Seq data have been deposited in the Genome Sequence Archive (Genomics, Proteomics & Bioinformatics 2021) in National Genomics Data Center (Nucleic Acids Res 2022), China National Center for Bioinformation/Beijing Institute of Genomics, and Chinese Academy of Sciences (GSA-Human: HRA007277), and are publicly accessible at https://ngdc.cncb.ac.cn/gsa-human .
Abbreviations
Antimicrobial peptide
C-C motif chemokine
Colony-stimulating factor
C-X-C motif chemokine ligand
Dulbecco’s modified Eagle’s medium
Dimethyl sulfoxide
Electrospray Ionization mass spectrometry
Fetal bovine serum
Human foreskin fibroblast cell
High-performance liquid chromatography
Interleukin
Optical density
Phosphate buffered saline
Scanning electron microscope
Transmission electron microscope
Tumor protein 53
References
Montoya JG, Liesenfeld O. Toxoplasmosis. Lancet. 2004;363:1965–76. https://doi.org/10.1016/s0140-6736(04)16412-x .
Article CAS PubMed Google Scholar
Flegr J, Prandota J, Sovičková M, Israili ZH. Toxoplasmosis–A global threat. Correlation of latent toxoplasmosis with specific disease burden in a set of 88 countries. PLoS ONE. 2014;9:e90203. https://doi.org/10.1371/journal.pone.0090203 .
Article CAS PubMed PubMed Central Google Scholar
Alday PH, Doggett JS. Drugs in development for toxoplasmosis: advances, challenges, and current status. Drug Des Devel Ther. 2017;11:273–93. https://doi.org/10.2147/dddt.S60973 .
Zhang JL, Si HF, Sun JC, Lv K, Yan BQ, Li B, et al. Determination of myrislignan levels in BALB/c mouse plasma by LC-MS/MS and a comparison of its pharmacokinetics after oral and intraperitoneal administration. BMC Vet Res. 2021;17:275. https://doi.org/10.1186/s12917-021-02990-y .
Shammaa AM, Powell TG, Benmerzouga I. Adverse outcomes associated with the treatment of Toxoplasma infections. Sci Rep. 2021;11:1035. https://doi.org/10.1038/s41598-020-80569-7 .
Doliwa C, Xia D, Escotte-Binet S, Newsham EL, Sanya JS, Aubert D, et al. Identification of differentially expressed proteins in sulfadiazine resistant and sensitive strains of Toxoplasma gondii using difference-gel electrophoresis (DIGE). Int J Parasitol Drugs Drug Resist. 2013;3:35–44. https://doi.org/10.1016/j.ijpddr.2012.12.002 .
Article PubMed PubMed Central Google Scholar
Wang JJ, Dou XJ, Song J, Lyu YF, Zhu X, Xu L, et al. Antimicrobial peptides: promising alternatives in the post feeding antibiotic era. Med Res Rev. 2019;39:831–59. https://doi.org/10.1002/med.21542 .
Ramazi S, Mohammadi N, Allahverdi A, Khalili E, Abdolmaleki P. A review on antimicrobial peptides databases and the computational tools. Database. 2022:baac011. https://doi.org/10.1093/database/baac011 .
Ahmed TAE, Hammami R. Recent insights into structure-function relationships of antimicrobial peptides. J Food Biochem. 2019;43:e12546. https://doi.org/10.1111/jfbc.12546 .
Article PubMed Google Scholar
De León-Nava MA, Romero-Núñez E, Luna-Nophal A, Bernáldez-Sarabia J, Sánchez-Campos LN, Licea-Navarro AF, et al. In vitro effect of the synthetic cal14.1a Conotoxin, derived from Conus californicus , on the Human Parasite Toxoplasma gondii . Mar Drugs. 2016;14:66. https://doi.org/10.3390/md14040066 .
Tanaka T, Maeda H, Matsuo T, Boldbattar D, Umemiya-Shirafuji R, Kume A, et al. Parasiticidal activity of Haemaphysalis longicornis longicin P4 peptide against Toxoplasma gondii . Peptides. 2012;34:242–50. https://doi.org/10.1016/j.peptides.2011.07.027 .
Liu R, Ni Y, Song J, Xu Z, Qiu J, Wang L, et al. Research on the effect and mechanism of antimicrobial peptides HPRP-A1/A2 work against Toxoplasma gondii infection. Parasite Immunol. 2019;41:e12619. https://doi.org/10.1111/pim.12619 .
Liu Y, Tang Y, Tang X, Wu M, Hou S, Liu X, et al. Anti- Toxoplasma gondii effects of a novel spider peptide XYP1 in vitro and in vivo. Biomedicines. 2021;9:934. https://doi.org/10.3390/biomedicines9080934 .
Paulsen VS, Blencke HM, Benincasa M, Haug T, Eksteen JJ, Styrvold OB, et al. Structure-activity relationships of the antimicrobial peptide arasin 1 - and mode of action studies of the N-terminal, proline-rich region. PLoS ONE. 2013;8:e53326. https://doi.org/10.1371/journal.pone.0053326 .
Dong N, Ma QQ, Shan AS, Lv YF, Hu W, Gu Y, et al. Novel design of short antimicrobial peptides derived from the bactericidal domain of avian β-defensin-4. Protein Pept Lett. 2012;19:1212–9. https://doi.org/10.2174/092986612803217006 .
Dong N, Ma QQ, Shan AS, Wang L, Sun WY, Li YZ. Influence of truncation of avian β-defensin-4 on biological activity and peptide-membrane interaction. Protein Pept Lett. 2012;19:430–8. https://doi.org/10.2174/092986612799789323 .
Sani MA, Separovic F. How membrane-active peptides get into lipid membranes. Acc Chem Res. 2016;49:1130–8. https://doi.org/10.1021/acs.accounts.6b00074 .
Lei J, Sun LC, Huang SY, Zhu CH, Li P, He J, et al. The antimicrobial peptides and their potential clinical applications. Am J Transl Res. 2019;11:3919–31.
CAS PubMed PubMed Central Google Scholar
Dawant T, Wang W, Spriggs M, Magela de Faria Junior G, Horton L, Szafranski NM, et al. Isolation of Toxoplasma gondii in cell culture: an alternative to bioassay. Int J Parasitol. 2024;54(3–4):131–137. https://doi.org/10.1016/j.ijpara.2023.12.002 .
Duvaud S, Gabella C, Lisacek F, Stockinger H, Ioannidis V, Durinx C. Expasy, the Swiss bioinformatics resource portal, as designed by its users. Nucleic Acids Res. 2021;49:W216–27. https://doi.org/10.1093/nar/gkab225 .
Zhou X, Zheng W, Li Y, Pearce R, Zhang CX, Bell EW, et al. I-TASSER-MTD: a deep-learning-based platform for multi-domain protein structure and function prediction. Nat Protoc. 2022;17:2326–53. https://doi.org/10.1038/s41596-022-00728-0 .
Gautier R, Douguet D, Antonny B, Drin G. HELIQUEST: a web server to screen sequences with specific alpha-helical properties. Bioinformatics. 2008;24:2101–2. https://doi.org/10.1093/bioinformatics/btn392 .
Hansen PR, Oddo A. Fmoc solid-phase peptide synthesis. Methods Mol Biol. 2015;1348:33–50. https://doi.org/10.1007/978-1-4939-2999-3_5 .
Nguyen TT, Kamyingkird K, Phimpraphai W, Inpankaew T. Viability of Toxoplasma gondii tachyzoites in different conditions for parasite transportation. Vet World. 2022;15:198–204. https://doi.org/10.14202/vetworld.2022.198-204 .
Stark M, Liu LP, Deber CM. Cationic hydrophobic peptides with antimicrobial activity. Antimicrob Agents Chemother. 2002;46:3585–90. https://doi.org/10.1128/aac.46.11.3585-3590.2002 .
You FT, Li C, Zhang SC, Zhang QS, Hu ZY, Wang YH, et al. Sitagliptin inhibits the survival, stemness and autophagy of glioma cells, and enhances temozolomide cytotoxicity. Biomed Pharmacother. 2023;162:114555. https://doi.org/10.1016/j.biopha.2023.114555 .
Li ZH, Li C, Szajnman SH, Rodriguez JB, Moreno SNJ. Synergistic activity between statins and bisphosphonates against acute experimental toxoplasmosis. Antimicrob Agents Chemother. 2017;61(8):e02628–16. https://doi.org/10.1128/aac.02628-16 .
Si HF, Xu CY, Zhang JL, Zhang XK, Li B, Zhou XZ, et al. Licochalcone A: an effective and low-toxicity compound against Toxoplasma gondii in vitro and in vivo. Int J Parasitol Drugs Drug Resist. 2018;8:238–45. https://doi.org/10.1016/j.ijpddr.2018.02.006 .
Bolger AM, Lohse M, Usadel B. Trimmomatic: a flexible trimmer for Illumina sequence data. Bioinformatics. 2014;30:2114–20. https://doi.org/10.1093/bioinformatics/btu170 .
Kim D, Langmead B, Salzberg SL. HISAT: a fast spliced aligner with low memory requirements. Nat Methods. 2015;12:357–60. https://doi.org/10.1038/nmeth.3317 .
Pertea M, Pertea GM, Antonescu CM, Chang TC, Mendell JT, Salzberg SL. StringTie enables improved reconstruction of a transcriptome from RNA-seq reads. Nat Biotechnol. 2015;33:290–5. https://doi.org/10.1038/nbt.3122 .
Resource TGO. 20 years and still GOing strong. Nucleic Acids Res. 2019;47:D330–8. https://doi.org/10.1093/nar/gky1055 .
Article CAS Google Scholar
Kanehisa M, Furumichi M, Sato Y, Kawashima M, Ishiguro-Watanabe M. KEGG for taxonomy-based analysis of pathways and genomes. Nucleic Acids Res. 2023;51:D587–92. https://doi.org/10.1093/nar/gkac963 .
Eza DE, Lucas SB. Fulminant toxoplasmosis causing fatal pneumonitis and myocarditis. HIV Med. 2006;7:415–20. https://doi.org/10.1111/j.1468-1293.2006.00393.x .
Dubey JP, Murata FHA, Cerqueira-Cézar CK, Kwok OCH, Villena I. Congenital toxoplasmosis in humans: an update of worldwide rate of congenital infections. Parasitology. 2021;148:1406–16. https://doi.org/10.1017/s0031182021001013 .
Rádis-Baptista G. Cell-penetrating peptides derived from animal venoms and toxins. Toxins. 2021;13:147. https://doi.org/10.3390/toxins13020147 .
Bolhassani A, Jafarzade BS, Mardani G. In vitro and in vivo delivery of therapeutic proteins using cell penetrating peptides. Peptides. 2017;87:50–63. https://doi.org/10.1016/j.peptides.2016.11.011 .
Torres MDT, Sothiselvam S, Lu TK, de la Fuente-Nunez C. Peptide design principles for antimicrobial applications. J Mol Biol. 2019;431:3547–67. https://doi.org/10.1016/j.jmb.2018.12.015 .
Langenegger N, Nentwig W, Kuhn-Nentwig L. Spider venom: components, modes of action, and novel strategies in transcriptomic and proteomic analyses. Toxins. 2019;11:611. https://doi.org/10.3390/toxins11100611 .
Zhang C, Yang M. Antimicrobial peptides: from design to clinical application. Antibiotics. 2022;11:349. https://doi.org/10.3390/antibiotics11030349 .
Falla TJ, Hancock RE. Improved activity of a synthetic indolicidin analog. Antimicrob Agents Chemother. 1997;41:771–5. https://doi.org/10.1128/aac.41.4.771 .
Smirnova MP, Afonin VG, Shpen VM, Tiagotin IV, Kolodkin NI. [Structure-function relationship between analogues of the antibacterial peptide indolicidin. I. Synthesis and biological activity of analogues with increased amphipathicity and elevated net positive charge of the molecule]. Bioorg Khim. 2004;30:458–65. https://doi.org/10.1023/b:rubi.0000043782.21640.c2 .
Wang JJ, Chou SL, Yang ZY, Yang Y, Wang ZH, Song J, et al. Combating drug-resistant fungi with novel imperfectly amphipathic palindromic peptides. J Med Chem. 2018;61:3889–907. https://doi.org/10.1021/acs.jmedchem.7b01729 .
Zhu X, Dong N, Wang ZY, Ma Z, Zhang LC, Ma QQ, et al. Design of imperfectly amphipathic α-helical antimicrobial peptides with enhanced cell selectivity. Acta Biomater. 2014;10:244–57. https://doi.org/10.1016/j.actbio.2013.08.043 .
Cutrona KJ, Kaufman BA, Figueroa DM, Elmore DE. Role of arginine and lysine in the antimicrobial mechanism of histone-derived antimicrobial peptides. FEBS Lett. 2015;589:3915–20. https://doi.org/10.1016/j.febslet.2015.11.002 .
Magana M, Pushpanathan M, Santos AL, Leanse L, Fernandez M, Ioannidis A, et al. The value of antimicrobial peptides in the age of resistance. Lancet Infect Dis. 2020;20:e216–30. https://doi.org/10.1016/s1473-3099(20)30327-3 .
Kindrachuk J, Napper S. Structure-activity relationships of multifunctional host defence peptides. Mini Rev Med Chem. 2010;10:596–614. https://doi.org/10.2174/138955710791383983 .
Rosenfeld Y, Lev N, Shai Y. Effect of the hydrophobicity to net positive charge ratio on antibacterial and anti-endotoxin activities of structurally similar antimicrobial peptides. Biochemistry. 2010;49:853–61. https://doi.org/10.1021/bi900724x .
Li SA, Xiang Y, Wang YJ, Liu J, Lee WH, Zhang Y. Naturally occurring antimicrobial peptide OH-CATH30 selectively regulates the innate immune response to protect against sepsis. J Med Chem. 2013;56:9136–45. https://doi.org/10.1021/jm401134n .
Yin LM, Edwards MA, Li J, Yip CM, Deber CM. Roles of hydrophobicity and charge distribution of cationic antimicrobial peptides in peptide-membrane interactions. J Biol Chem. 2012;287:7738–45. https://doi.org/10.1074/jbc.M111.303602 .
Chen Y, Guarnieri MT, Vasil AI, Vasil ML, Mant CT, Hodges RS. Role of peptide hydrophobicity in the mechanism of action of alpha-helical antimicrobial peptides. Antimicrob Agents Chemother. 2007;51:1398–406. https://doi.org/10.1128/aac.00925-06 .
Azmi S, Srivastava S, Mishra NN, Tripathi JK, Shukla PK, Ghosh JK. Characterization of antimicrobial, cytotoxic, and antiendotoxin properties of short peptides with different hydrophobic amino acids at “a” and “d” positions of a heptad repeat sequence. J Med Chem. 2013;56:924–39. https://doi.org/10.1021/jm301407k .
Zhu BY, Zhou NE, Kay CM, Hodges RS. Packing and hydrophobicity effects on protein folding and stability: effects of beta-branched amino acids, valine and isoleucine, on the formation and stability of two-stranded alpha-helical coiled coils/leucine zippers. Protein Sci. 1993;2:383–94. https://doi.org/10.1002/pro.5560020310 .
Li DY, Wu MH. Pattern recognition receptors in health and diseases. Signal Transduct Target Ther. 2021;6:291. https://doi.org/10.1038/s41392-021-00687-0 .
Quinn SR, O’Neill LA. A trio of microRNAs that control Toll-like receptor signalling. Int Immunol. 2011;23:421–5. https://doi.org/10.1093/intimm/dxr034 .
Scanga CA, Aliberti J, Jankovic D, Tilloy F, Bennouna S, Denkers EY, et al. Cutting edge: MyD88 is required for resistance to Toxoplasma gondii infection and regulates parasite-induced IL-12 production by dendritic cells. J Immunol. 2002;168:5997–6001. https://doi.org/10.4049/jimmunol.168.12.5997 .
Deckert-Schlüter M, Albrecht S, Hof H, Wiestler OD, Schlüter D. Dynamics of the intracerebral and splenic cytokine mRNA production in Toxoplasma gondii -resistant and -susceptible congenic strains of mice. Immunology. 1995;85:408–18.
PubMed PubMed Central Google Scholar
Nagineni CN, Pardhasaradhi K, Martins MC, Detrick B, Hooks JJ. Mechanisms of interferon-induced inhibition of Toxoplasma gondii replication in human retinal pigment epithelial cells. Infect Immun. 1996;64:4188–96. https://doi.org/10.1128/iai.64.10.4188-4196.1996 .
Coto E, Reguero JR, Avanzas P, Pascual I, Martín M, Hevia S, et al. Gene variants in the NF-KB pathway (NFKB1, NFKBIA, NFKBIZ) and risk for early-onset coronary artery disease. Immunol Lett. 2019;208:39–43. https://doi.org/10.1016/j.imlet.2019.02.007 .
Hunter CA, Sibley LD. Modulation of innate immunity by Toxoplasma gondii virulence effectors. Nat Rev Microbiol. 2012;10:766–78. https://doi.org/10.1038/nrmicro2858 .
Download references
Acknowledgements
We express our gratitude to all participants involved in this research.
The work was supported by the National Natural Science Foundation of China (32170510) and Hunan graduate research innovation project (QL20230021).
Author information
Jing Li and Kaijuan Wu contributed equally to this work.
Authors and Affiliations
Department of Parasitology, School of Basic Medical Sciences, Central South University, Changsha, 410013, Hunan, China
Jing Li, Kaijuan Wu, Xiaohua Liu, Dongqian Yang, Jing Xie, Yixiao Wang, Kang Liu & Liping Jiang
China-Africa Research Center of Infectious Diseases, Central South University, Changsha, 410013, Hunan, China
Liping Jiang
Department of Vascular Surgery, The Third Xiangya Hospital, Central South University, Changsha, 410013, Hunan, China
Hunan Key Laboratory of Traditional Chinese Veterinary Medicine, Hunan Agricultural University, Changsha, 410128, China
You can also search for this author in PubMed Google Scholar
Contributions
L.J., J.L., and K.W. participated in the conception and design of this study. J.L., K.W., X.L., D.Y., J.X., Y.W., and K.L. performed the experiments and analyzed the results. Z.W. and W.L. provided guidance and advice on transcriptome analysis. J.L. and K.W. wrote the manuscript. L.J. reviewed the manuscript and acquired the funding support. All authors made contributions to the final version and approved the submission.
Corresponding author
Correspondence to Liping Jiang .
Ethics declarations
Ethics approval and consent to participate.
This study was approved by the Ethics Committee of the School of Basic Medical Science, Central South University, Changsha, China (protocol code: 2020KT-11), and complied with national regulations on the ethical treatment of experimental animals.
Consent for publication
Not applicable.
Competing interests
The authors declare no competing interests.
Additional information
Publisher’s note.
Springer Nature remains neutral with regard to jurisdictional claims in published maps and institutional affiliations.
Supplementary Information
Additional file 1: table s1..
The properties and parameters of XYP1 and its derived peptide. Table S2. The hub proteins of intersection of three algorithms. Figure S1. The ESI–MS results of XYP1-15, XYP1-15-1, XYP1-16, XYP1-16-1. The abscissa is the mass charge ratio (m/z), and the ordinate is the ionic strength. Figure S2. The ESI–MS results of XYP1-17, XYP1-17-1, XYP1-18, XYP1-18-1. Figure S3. GO enrichment analysis of differential genes. (A) MA map of differentially expressed genes. The X -axis is the normalized average expression of genes in all samples involved in the comparison, and the Y -axis is log2Fold Change. Red indicates significant differential genes. (B) Comparison map of differentially expressed genes and distribution of all genes at GO level. Blue represents all the gene-enriched GO entries, red represents the differential gene-enriched GO entries, horizontal axis represents the entry name, and vertical axis represents the number of genes corresponding to the entry and its percentage.
Rights and permissions
Open Access This article is licensed under a Creative Commons Attribution 4.0 International License, which permits use, sharing, adaptation, distribution and reproduction in any medium or format, as long as you give appropriate credit to the original author(s) and the source, provide a link to the Creative Commons licence, and indicate if changes were made. The images or other third party material in this article are included in the article's Creative Commons licence, unless indicated otherwise in a credit line to the material. If material is not included in the article's Creative Commons licence and your intended use is not permitted by statutory regulation or exceeds the permitted use, you will need to obtain permission directly from the copyright holder. To view a copy of this licence, visit http://creativecommons.org/licenses/by/4.0/ . The Creative Commons Public Domain Dedication waiver ( http://creativecommons.org/publicdomain/zero/1.0/ ) applies to the data made available in this article, unless otherwise stated in a credit line to the data.
Reprints and permissions
About this article
Cite this article.
Li, J., Wu, K., Liu, X. et al. Anti- Toxoplasma gondii effects of XYP1-derived peptides and regulatory mechanisms of XYP1. Parasites Vectors 17 , 376 (2024). https://doi.org/10.1186/s13071-024-06455-7
Download citation
Received : 28 May 2024
Accepted : 16 August 2024
Published : 04 September 2024
DOI : https://doi.org/10.1186/s13071-024-06455-7
Share this article
Anyone you share the following link with will be able to read this content:
Sorry, a shareable link is not currently available for this article.
Provided by the Springer Nature SharedIt content-sharing initiative
- XYP1-derived peptides
- Toxoplasmosis
- Anti- Toxoplasma gondii activity
- Transcriptome analysis
Parasites & Vectors
ISSN: 1756-3305
- Submission enquiries: [email protected]

IMAGES
VIDEO
COMMENTS
A Travelling microscope is a compound microscope that is fitted on a vertical scale. It carries a vernier scale along the main scale and can be moved upward or downward. Below is an experiment to determine refractive index of a glass slab using a travelling microscope.
When an object placed in optically denser medium (glass slab) is viewed from a rarer medium (air), it appears to be at a depth less than the real depth due t...
A travelling microscope, a glass slab, lycopodium powder/chalk dust and a paper. 141414 Fig. E 14.1 Formation of image I of a point 0 in a glass slab ... Y ou may use a thin glass beaker for this experiment. 2. You have three slabs of same dimensions - the first one being hollow and completely filled with water , the second one is made of ...
these are physics lab video recordings for practical knowledge , link for channel introduction. https://youtu.be/AxbrYWI-DpgLinks of different video : -Chan...
To determine refractive index of the glass slab using travelling microscope for more detailed explanation of readings you can watch video : https://youtu.be/...
The following is an experiment utilising a travelling microscope to determine the refractive index of a glass slab. The refractive index is a measurement of how far a light ray deviates when it pauses between two materials. It's a one-dimensional integer that determines the speed of light. The refractive index is defined as the ratio of light ...
Tips for Using a Travelling Microscope. Using a travelling microscope can be an exciting and fascinating experience, allowing you to explore the world beyond what the naked eye can see. To make the most of this powerful tool, here are some helpful tips: 1. Set up in a well-lit area: Proper lighting is crucial when using a travelling microscope.
Refractive Index Calculation = R3 - R1/R3 - R2. Mean Refractive Index = n1 + n2 + n3/3. Precaution-Ensure that you remove the parallax properly in step 6, failing which results of this travelling microscope experiment can be erroneous.To know more about refractive index and experiments in general, consult our live online classes.
The Travelling Microscope. The sliding carriage of the traveling microscope rides on carefully machined ways, pushed by a nut under the carriage which rides on the micrometer screw. The nut must not fit tightly on the screw or it will bind; hence there is always some slack built into the mechanism. When the nut is being pulled to the right ...
Travelling Microscope consists of an ordinary compound microscope which slides along a graduated vertical pillar, attached to horizontal base resting on the leveling screws. The main scales divisions along with vernier scale divisions are marked on the horizontal base and the vertical pillar as shown in fig.3. The microscope can be moved up and
let us see the setup for this experiment. this is the setup for the experiment, I need only one microscope travelling microscope and I have a beakers. And beakers; there is a you can see this there is an object this is marked say it is a S, it is a some marking at the bottom of the beaker, inside bottom. that that is our object.
68. An experiment is performed to find the refractive index of glass using a travelling microscope. In this experiment distances are measured by (a) a screw gauge provided on the microscope (b) a vernier scale provided on the microscope (c) a standard laboratory scale (d) a meter scale provided on the microscope. 69.
This video demonstrates how to take a reading using travelling microscope for students who enrolled in SP015 and DP014 courses.
A traveling microscope. E—eyepiece, O—objective, K—knob for focusing, V—vernier, R—rails, S—screw for fine position adjustment. A travelling microscope is an instrument for measuring length with a resolution typically in the order of 0.01mm. The precision is such that better-quality instruments have measuring scales made from Invar to avoid misreadings due to thermal effects.
A travelling microscope is a device which measures. length with a resolution in order of 0.01mm. The. precision is such that better-qualit y instruments h ave. measuring scales made from Invar ...
To determine the refractive index of a glass slab using a travelling microscope.; Practical : 16. To assemble a household circuit comparing three bulbs, three (on - off) switches, a free a power source.; Practical : 17. To assemble the component of a given electric circuit
Travelling Microscope - Free download as PDF File (.pdf), Text File (.txt) or read online for free. The document describes an experiment to determine the refractive index of a glass slab using a travelling microscope by measuring the apparent and real depths of an ink mark with and without the glass slab, calculating the refractive index as the real depth divided by the apparent depth ...
In this video you will get full information about Travelling Microscope :-(i) Least Count of Travelling Microscope(ii) How to take readings from Travelling M...
A travelling microscope is an instrument for measuring length with a resolution typically in the order of 0.01mm. The precision is such that better-quality instruments have measuring scales made from Invar to avoid misreadings due to thermal effects. The instrument comprises a microscope mounted on two rails fixed to, or part of a very rigid bed. The position of the microscope can be varied ...
The Travelling Microscope. The sliding carriage of the traveling microscope rides on carefully machined ways, pushed by a nut under the carriage which rides on the micrometer screw. The nut must not fit tightly on the screw or it will bind; hence there is always some slack built into the mechanism. When the nut is being pulled to the right ...
The apparatus is a little complicated but fairly basic, and could probably be further simplified. It claims an accuracy of at least an order of magnitude better than the microscope method. (Item #3 on page 1 of a Google Search "measure diameter capillary tube". The article cited in J Shupperd's answer was #1 in the same search.
travelling microscope se readings kaise lete haito determine refractive index of glass slab using travelling microscopecbseclass 12 physics practical
The anti-T. gondii effect was evaluated by trypan blue staining assay and killing experiment of RH strain tachyzoites. The CCK8 and hemolysis assays were used to compare their safeties. The morphological changes of T. gondii were observed by scanning electron microscope and transmission electron microscope.
This experiment consists of two parts:1. Measuring height of the water column entered in the capillary tube. a) Focus on the water meniscus. b) Fo...