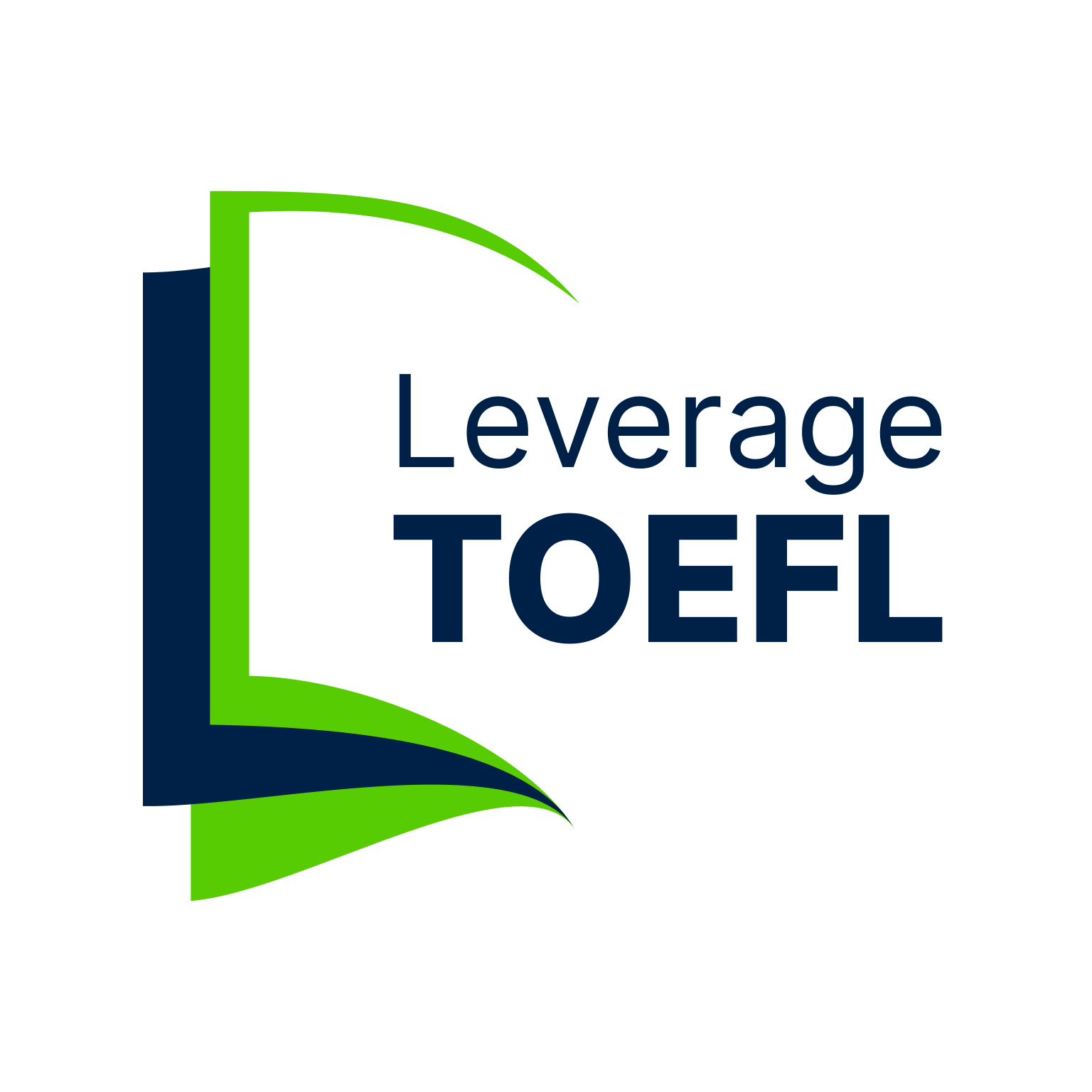

45,000+ students realised their study abroad dream with us. Take the first step today
Here’s your new year gift, one app for all your, study abroad needs, start your journey, track your progress, grow with the community and so much more.

Verification Code
An OTP has been sent to your registered mobile no. Please verify
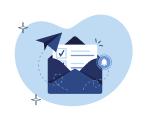
Thanks for your comment !
Our team will review it before it's shown to our readers.

Essay on Scientific Discoveries
- Updated on
- Feb 7, 2024
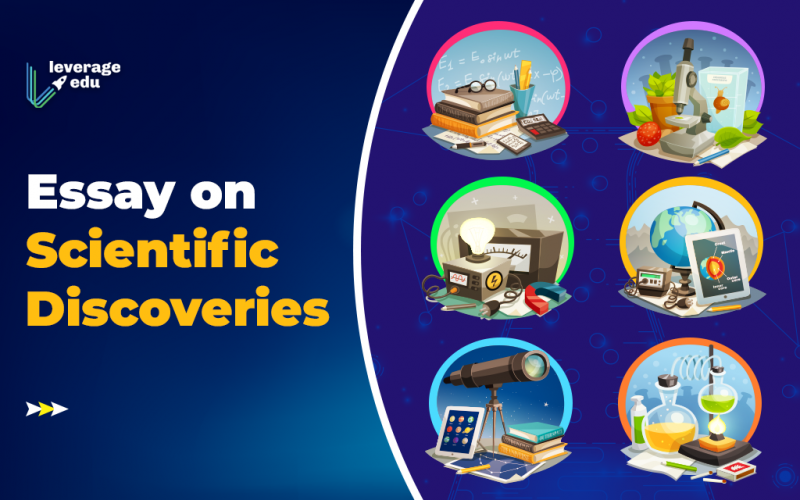
Writing and speaking skills are the most important skills in the world. It shows how well a student will convey his or her ideas, experiences and thoughts. Essays are one of the most popular forms of writing to ascertain an applicant’s general knowledge, experiences, writing style and language skills. It is used in many entrance exams like SAT, IELTS, TOEFL and in college applications as well. From a very early age, school curriculums have been encouraging students to write essays and give speeches. Sometimes the topics provided to students can be complicated. So, today we have come up to help the students with an essay on Scientific Discoveries.
Check out our 200+ Essay Topics for School Students in English
Five Qualities of A Good Essay
Before we provide you with an essay on scientific discoveries. Let’s learn about essay writing. Writing an essay is a difficult thing. The writing should be rich in content plus should not bore its readers. Here are the five qualities a perfect essay should have:-
- Focus: All of your writing should come under one single topic. No matter how vast your essay is, it should always revolve around the topic of the essay. Avoid unnecessary details.
- Development: Every paragraph of your essay should centre the topic of your essay. Try to use examples, details and descriptions.
- Free composition: Always follow a basic structure. Before finalising your essay, jot down the points you would like to mention and then make a series. Do not surprise the reader with complicated words, try to keep it as simple as possible.
- Correctness: Make sure your essay is free from any grammatical errors, spelling mistakes, mismatched sentences, etc. Always use standard English and complete sentences.
- Introduction and Conclusion: The introduction and the conclusion of the writing are the most important parts of the essay. The first impression is always the last, and so is the introduction of your writing. After reading the first two or three lines, if the reader gets bored, he may not read your whole essay. So make sure your essay contains a crispy beginning. Alternatively, make the conclusion so strong and effective that the reader never forgets your essay. Don’t feel afraid to use quotes, catchy lines, slogans and all. They are the cherry on the cake for your essay.
Also Read: Essay on Yoga Day
Also Read: Speech on Yoga Day
Also Read: Essay on Athletics in 100, 200 and 300 Words
Sample Essay on Scientific Discoveries
Here is an example of an essay on scientific discoveries to help them out in their school assignments.
Everything around us is a great discovery. Be it a necessity, comfort, or luxury, they all came from different scientific discoveries that took place over some time. Starting from a small pin to a big ship, everything is just a mere invention to make the lives of humans easier. Scientistic discoveries take place in every arena of thought so before we talk about these inventions. Let’s examine what is science. What is science? Science is a system for acquiring knowledge. We use observations, and experimentation to come to a conclusion and explain any natural phenomenon. In simple language, science is the systematic field of study or knowledge gained from experimentations, observations and some accepted facts. And so scientific discoveries have done miracles in human lives. Scientific discoveries and inventions have made our lives easier and more comfortable than we could have ever imagined. Scientific equipment accomplishes lengthy tasks in just minutes. Be it in the health sector, education, transportation, and more. All the inventions are just the gifts of science. Nowadays we are in a situation where without science, we cannot imagine our survival. In the absence of Science, no country, and no single person would have made progress. Scientific discoveries and inventions are machines that accomplish any task of humans either fully or partially. According to the business dictionary, the word ‘invention’ is “a new scientific or technical idea and the means of its embodiment or accomplishment. To be patentable, an invention must be novel, have utility, and be non-obvious. To be called an invention, an idea only needs to be proven as workable. But to be called an innovation, it must also be replicable at an economical cost and must satisfy a specific need. That’s why only a few inventions lead to innovations because not all of them are economically feasible.” Wikipedia further says, “An invention is a unique or novel device, method, composition or process. It may be an improvement upon a machine or product or a new process for creating an object or a result. An invention that achieves a unique function or result may be a radical breakthrough. Such works are novel and not obvious to others skilled in the same field.” These definitions made us clear about how important scientific discovery is for us. Due to science, we can get all kinds of things we desire for. Electricity is a miracle that gives us light even in the dark. It further helps us to run industries conserve the environment and control pollution . A cricket match is going on in America and we can watch it. Why? Inventions! Nowadays medical science is doing its best all over the world. Let us not forget computers, which is the greatest invention of mankind. However, it is rightly said that every coin has two sides. Scientific discoveries and inventions have given us a lot and at the same time created a lot of disadvantages too. Nowadays people have become so dependent on technology that even walking has become difficult. Inventions made people so lazy, especially the young generation. All they could think about now is sitting at their home, with their computers and tablets on.
Gone are the days when people used to go out, play and have actual fun in life. Also, scientific inventions have made people jobless. Employers are substituting their employees with heavy machines. And this is the sad reality everywhere. Along with a luxurious life, technology has made our lives more complicated. People nowadays catch the disease early due to no exercise and sitting in front of their computer the whole day. The biggest and most disastrous inventions are weapons, guns and bombs. What’s worse than taking the life of people? It has ruined unity, peace and harmony all over the world. Scientific discoveries and inventions have contributed so much that my essay would never be enough to explain it. Ultimately, I would like to say that do not take up the monstrous side. Try the blessing of discoveries and make your life better in every aspect.
Also Read: Essay on Information Technology in 400 Words
Check out our Popular Essay Topics for Students
So this was all regarding an essay on scientific discoveries. For more information on such interesting topics, visit our essay writing page and follow Leverage Edu.
Team Leverage Edu
Leave a Reply Cancel reply
Save my name, email, and website in this browser for the next time I comment.
Contact no. *
Good work thanks
Thank you, Deepesh!
Good work🙏 sir thanks for sharing
Thank u it was very helpful
Thank you this helped me a lot
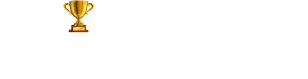
Leaving already?
8 Universities with higher ROI than IITs and IIMs
Grab this one-time opportunity to download this ebook
Connect With Us
45,000+ students realised their study abroad dream with us. take the first step today..

Resend OTP in

Need help with?
Study abroad.
UK, Canada, US & More
IELTS, GRE, GMAT & More
Scholarship, Loans & Forex
Country Preference
New Zealand
Which English test are you planning to take?
Which academic test are you planning to take.
Not Sure yet
When are you planning to take the exam?
Already booked my exam slot
Within 2 Months
Want to learn about the test
Which Degree do you wish to pursue?
When do you want to start studying abroad.
January 2025
September 2025
What is your budget to study abroad?
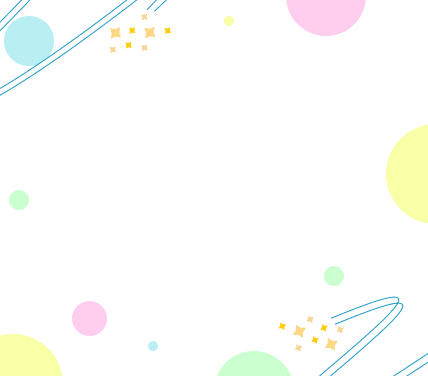
How would you describe this article ?
Please rate this article
We would like to hear more.
- The Magazine
- Stay Curious
- The Sciences
- Environment
- Planet Earth
10 Scientific Discoveries That Changed The World
Dna, gravity, and germ theory are a few of the key findings in history that forever shifted the course of human civilization. learn how these scientific discoveries changed the world..
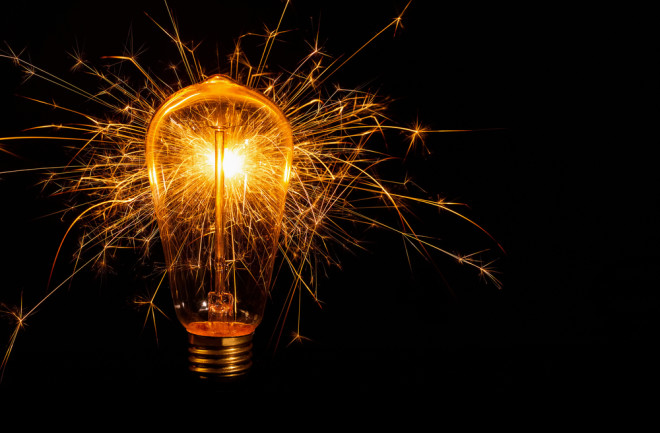
The only constant is change. At least, that’s what the Greek philosopher Heraclitus is credited to have said. And while science and philosophy don’t always go hand in hand, there is some truth to Heraclitus’ notion. Change is inevitable and, in some cases, necessary for our species to evolve . While some change happens automatically, like the tides going in and out, some changes bloomed from scientific discoveries.
Using fire to cook food and keep warm propelled our ancestors toward the foundations of early settlements and continued the growth of civilization. Using fire to shape metals for weapons and building materials led to more and more discoveries and more and more advancements. While many advances shaped humanity, we’ve focused on ten significant scientific discoveries that changed the world.
The discovery of DNA didn’t so much change the world as it did our understanding of it — more so, our understanding of life. DNA is a term we’ve only started using in the 20th century, though its initial discovery dates back decades into the 19th century.
What Is DNA?
DNA is the molecule that encodes genetic information for all living things. It plays a key role in passing traits from parents to offspring and is the primary component of chromosomes in the cell nuclei of complex organisms.
Who Discovered DNA?
Many people think scientists James Watson and Francis Crick discovered DNA in the 1950s. Nope, not so fast. DNA was actually first discovered in 1869 by Swiss physician Friedrich Miescher . He identified what he referred to as “nuclein” in blood cells. Several other researchers have worked on projects around identifying DNA up until Watson and Crick.
What Does DNA Stand For?
The term nuclein eventually evolved into what we know as DNA, the shorthand for deoxyribonucleic acid. German biochemist Albrecht Kossel , who would later go on to win the Nobel Prize, is often credited with the name.
Levene’s Polynucleotide Model
Other scientists, such as Phoebus Levene , built on Miescher’s work over the years. Levene didn't know how DNA's nucleotide components were arranged. He proposed the polynucleotide model, correctly suggesting that nucleic acids are chains of nucleotides, each with a base, a sugar, and a phosphate group.
Watson and Crick's Double-Stranded Helix
Watson and Crick and “their” groundbreaking discovery in the field of genetics accurately identified DNA’s double-stranded helix structure, connected by hydrogen bonds. For their discovery, Watson and Crick won a Nobel Prize in 1962 and worldwide acclaim.
Though Watson and Crick won a Nobel Prize, years later, we’ve learned that the duo likely took research without permission from chemist Rosalind Franklin . Thanks to her research, the double helix structure was realized, though her Nobel Prize was not.
In 2014, Watson auctioned off his Nobel Prize medal for over $4 million. The buyer was a Russian billionaire who returned it to Watson a year later. In 2019, Watson was stripped of his honorary titles because of racist comments.
Read More: DNA in Unlikely Places Helps Piece Together Ancient Humans' Family Trees
2. Earth in Motion
While it may be common knowledge that Earth spins on an axis and revolves around the sun, at one point, this idea was extremely outlandish. How could the planet move and we not feel it? Thanks to a few clever scientists, the Earth in Motion theory became more than a wild idea.
What Is Earth in Motion?
Earth in motion refers to the understanding that Earth is not stationary but moves in different ways. Earth rotates on an axis and revolves around a star.
Earth’s Rotation
Earth rotates on its axis , which is an imaginary line running from the North Pole to the South Pole. This rotation is responsible for the day-night cycle, with one complete rotation taking about 24 hours.
Earth’s Revolution
Earth revolves around the Sun, completing one orbit approximately every 365 days. This revolution, combined with the tilt of the Earth's axis, leads to the changing seasons.
Who Discovered Earth's Motion?
The discovery and acceptance of Earth's motion was a gradual process involving several key figures in the history of science.
Aristarchus Hypothesis of Earth’s Motion
An ancient Greek astronomer, Aristarchus of Samos, was one of the first to suggest that Earth orbits the Sun . This view was not widely accepted in his time as it was believed Earth was the center of the Universe, and stars, planets, and the sun all revolved around our planet.
Copernicus Creates the First Model of Earth’s Motion
Mathematician and astronomer Nicolaus Copernicus is often credited with proposing the first heliocentric model of the universe. In 1543, he published his great work, On the Revolutions of the Heavenly Spheres , which explained his theories.
Among them was that day and night was created by the Earth spinning on its axis. Copernican heliocentrism replaced the conventionally accepted Ptolemaic theory , which asserted that the Earth was stationary. Copernicus’ work was largely unknown during his lifetime but later gained support.
Galileo Galilei’s Telescopic Observations
Galileo Galilei agreed with Copernicus’ theory and proved it through his telescopic observations. In 1610, he observed phases of Venus and the moons of Jupiter, which were strong evidence against the Earth-centered model of the universe.
Galileo agreed with Copernicus’ theory and proved it by using a telescope to confirm that the different phases Venus went through resulted from orbiting around the sun.
Johannes Kepler’s Planetary Laws
German mathematician Johannes Kepler formulated a series of laws detailing the orbits of planets around the Sun. These laws, which remain relevant today, provided mathematical equations for accurately predicting planetary movements in line with the Copernican theory.
Why Don’t We Feel Earth Spinning?
According to researchers at the California Institute of Technology (CalTech), Earth spins smoothly and at a consistent speed. If Earth were to change speeds at any time, we’d feel it.
Read More: Earth's Rotation Has Slowed Down Over Billions of Years
3. Electricity
Did benjamin franklin discover electricity.
It’s a common misconception that Ben Franklin discovered electricity with his famous kite experiment. But his 1752 experiment, which used a key and kite, instead demonstrated that lightning is a form of electricity . Another myth is that Franklin was struck by lightning. He wasn’t, but the storm did charge the kite.
Who First Observed Electricity?
Back in 600 B.C.E., it was the ancient Greek philosopher Thales of Miletus who first observed static electricity when fur was rubbed against fossilized tree resin, known as amber.
Who Invented Electricity?
British scientist and doctor William Gilbert coined the word “electric,” derived from the Greek word for amber. Regarded as the “father of electricity,” Gilbert was also the first person to use the terms magnetic pole, electric force, and electric attraction. In 1600, his six-volume book set, De Magnete , was published. Among other ideas, it included the hypothesis that Earth itself is a magnet.
Read More: Ben Franklin: Founding Father, Citizen Scientist
4. Germ Theory of Disease
What is the germ theory of disease.
Germ theory is a scientific principle in medicine that attributes the cause of many diseases to microorganisms, such as bacteria and viruses, that invade and multiply within the human body. This theory was a significant shift from previous beliefs about disease causation.
Who Invented the Germ Theory?
Louis Pasteur discovered germ theory when he demonstrated that living microorganisms caused fermentation , which could make milk and wine turn sour. From there, his experiments revealed that these microbes could be destroyed by heating them — a process we now know as pasteurization.
This advance was a game changer, saving people from getting sick from the bacteria in unpasteurized foods , such as eggs, milk, and cheeses. Before Pasteur, everyday people and scientists alike believed that disease came from inside the body.
Pasteur’s work proved that germ theory was true and that disease was the result of microorganisms attacking the body. Because of Pasteur, attitudes changed, and became more accepting of germ theory.
How Did Koch’s Postulates Contribute to Germ Theory?
The German physician and microbiologist Robert Koch played a crucial role in establishing a systematic methodology for proving the causal relationship between microbes and diseases .
He formulated Koch's postulates and applied these principles to identify the bacteria responsible for tuberculosis and cholera, among other diseases.
Together, Pasteur and Koch laid the foundation for bacteriology as a science and dramatically shifted the medical community's understanding of infectious diseases. Their work led to improved hygiene, the development of vaccines, and the advancement of public health measures.
Read More: Why Do Some People Get Sick All the Time, While Others Stay in Freakishly Good Health?
Who Discovered Gravity?
Isaac Newton didn’t really get hit on the head with an apple, as far as we know. But seeing an apple fall from a tree did spark an idea that would lead the mathematician and physicist to discover gravity at the age of just 23.
He pondered about how the force pulls objects straight to the ground, as opposed to following a curved path, like a fired cannonball. Gravity was the answer — a force that pulls objects toward each other.
How Does Gravity Work?
The greater the mass an object has, the greater the force or gravitational pull. When objects are farther apart, the weaker the force. Newton’s work and his understanding of gravity are used to explain everything from the trajectory of a baseball to the Earth’s orbit around the sun. But Newton’s discoveries didn’t stop there.
Newton’s Laws of Motion
In 1687, Newton published his book Principia , which expanded on his laws of universal gravitation and his three laws of motion. His work laid the foundation for modern physics.
Building on the discovery, advancements in the field of electricity continued.
In 1800, Italian physicist Alessandro Volta created the first voltaic pile , an early form of an electric battery.
Einstein’s Theory of General Relativity
In 1915, Einstein proposed the theory of general relativity . This theory redefined gravity not as a force but as a curvature of spacetime caused by the presence of mass and energy.
According to Einstein, massive objects cause a distortion in the fabric of space and time, similar to how a heavy ball placed on a trampoline causes it to warp. Other objects move along the curves in spacetime created by this distortion.
Both Newton and Einstein significantly advanced our understanding of gravity. Their theories marked critical milestones in the field of physics and have had far-reaching implications in science and technology.
Read More: 5 Eccentric Facts About Isaac Newton
6. Antibiotics
Much like Germ Theory revolutionized modern medicine, so too did the invention of antibiotics. This discovery would go on to save countless lives.
When Were Antibiotics Invented?
According to the Microbiology Society , humans have been using some form of antibiotics for millennia. It was only in recent history that humans realized that bacteria caused certain infections and that we could now provide readily available treatment.
In 1909, German physician Paul Ehrlich noticed that certain chemical dyes did not color certain bacteria cells as it did for others. Because of this, he believed that it would be possible to kill certain bacteria without killing the other cells around it. Ehrlich went on to discover the cure for syphilis, which many in the scientific community refer to as the first antibiotic. However, Ehrlich referred to his discovery as chemotherapy because it used chemicals to treat a disease. Ehrlich is referred to as the “Father of Immunology” for his discoveries.
Ukrainian-American microbiologist Selman Waksman coined the term “antibiotic” about 30 years later, according to the Microbiology Society.
Who Discovered Penicillin?
One of the most recognizable antibiotics known today is penicillin. Health professionals prescribe millions of patients with this antibiotic each year. However, one of the most well-known antibiotics was discovered by accident.
In 1928, after some time away from the lab, Alexander Fleming — a Scottish microbiologist — discovered that a fungus Penicillium notatum had contaminated a culture plate with Staph bacteria. Fleming noticed that the fungus had created bacteria-free areas on the plate. After multiple trials, Fleming was able to successfully prove that P. notatum prevented the growth of Staph. Soon the antibiotic was ready for mass production and helped save many lives during World War Two.
What Is Penicillin Used For?
Penicillin is used to treat infections caused by bacteria. The medication works by stopping and preventing the growth of bacteria.
Read More: Antibiotic-Resistant Bacteria: What They Are and How Scientists Are Combating Them
7. The Big Bang Theory
The Big Bang Theory is one of the most widely accepted theories on the beginning of the universe. The theory claims that about 13.7 billion years ago, all matter of the universe was condensed into one small point. After a massive explosion, the contents of the universe burst forth and expanded and continue to expand today.
Who Came Up With the Big Bang Theory?
This first mention of the Big Bang came from Georges Lemaître, a Belgian cosmologist and Catholic priest. Initially, in 1927, Lemaître published a paper about General Relativity and solutions to the equations around it. Though it mostly went unnoticed.
Though many scientists didn’t believe that the universe was expanding, a group of cosmologists was beginning to go against the grain. After Edwin Hubble noticed that galaxies further away from our own seemed to be pulling away faster than those closer to us, the idea of the universe expanding seemed to make more sense. Lemaître’s 1927 paper was recognized, and the term Big Bang appeared in Lemaître’s 1931 paper on the subject.
What Is the Hubble Space Telescope?
Edwin Hubble’s discovery that galaxies are moving away from our own, dubbed Hubble’s Law, is on a long list of his many discoveries. Though this discovery helped add evidence to the Big Bang Theory, this discovery was hindered by the same thing that had been distributing telescopes since their inception: Earth’s atmosphere. According to NASA , Earth’s atmosphere distorts light, limiting how far a telescope can see, even on a clear night.
Because of this, researchers, specifically Lyman Spitzer , suggested putting a telescope in space, just beyond Earth’s atmosphere and into its orbit. After a few attempts in the 1960s and 70s, NASA, along with contributions from the European Space Agency (ESA), launched a space telescope on April 24, 1990 . The Hubble Space Telescope, named for the pioneering cosmologist, became the strongest telescope known to humankind until the 2021 launch of the James Webb Space Telescope .
What Is The Cosmic Microwave Background?
The Big Bang emitted large amounts of primeval light , according to the ESA. Over time, this light “cooled” and was no longer visible. However, researchers are able to detect what is known as Cosmic Microwave Background (CMB), which is, according to the ESA, the cooled remnant of the first light to travel through the universe. Some researchers even refer to CMB as an echo of the Big Bang.
Read More: Did the Big Bang Happen More Than Once?
8. Vaccines
“An ounce of prevention is worth a pound of cure,” Benjamin Franklin once said. A statement that, at the time, applied to making towns safer against fires. However, the same statement can be true for health and wellness. The advent of vaccines has helped prevent several serious diseases and keep people safe. Thanks to vaccines, people rarely get diseases like polio, and smallpox has been eradicated .
What Is a Vaccine?
According to the Centers for Disease Control (CDC), a vaccine is a method of protection that introduces a small amount of disease to the human body so that the body can form an immune response should that disease try to enter the body again.
Basically, through a vaccine, the human body is exposed to a small out of a disease so that the immune system can build a defense against it.

When Was the First Vaccine Created?
According to the World Health Organization (WHO), Dr. Edward Jenner created the first vaccine in 1796 by using infected material from a cowpox sore — a disease similar to smallpox. He inoculated an 8-year-old boy named James Phipps with the matter and found that the boy, though he didn’t feel well at first, recovered from the illness.
A few months later, Jenner tested Phipps with material from a smallpox sore and found that Phipps did not get ill at all. From there, the smallpox vaccine prevented countless deaths in the centuries to come.
When Was the Polio Vaccine Invented?
From 1796 to 1945, doctors and scientists worked hard to create vaccines for other serious illnesses like the Spanish Flu, yellow fever, and influenza. One of these doctors was Jonas Salk. After Salk helped develop an influenza vaccine in 1945, he began working on the Polio vaccine. Between 1952 and 1955, Salk finished the vaccine, and clinical trials began. Salk’s vacation method required a needle and syringe, though, by 1960, Albert Sabin had created a different delivery method for the polio vaccine. Sabin’s version could be administered by drops or on a sugar cube.
Read More: The History of the Polio Vaccine
9. Evolution
What is evolution .
Evolution is a theory that suggests that organisms change and adapt to their environment on a genetic level from one generation to the next. This can take millions of years through methods such as natural selection. An animal’s color or beak may alter over time depending on the changes in their environment, helping them hide from predators or better capture prey.
Who Is the Father of Evolution?
After studying animals in the Galapagos , particularly the finches, a naturalist named Charles Darwin determined that the birds — who all resided on different Galapagos islands — were the same or similar species but had distinct characteristics. Darwin noted that the finches from each island had different beaks. These beaks helped the finches forage for their main food source on their specific island. Some had larger beaks for cracking open nuts and seeds, while others had smaller and more narrow beaks for finding insects.
These observations earned Charles Darwin the title of the Father of Evolution. Though the theory of evolution has changed since Darwin published On the Origin of Species in 1859, he helped lay the framework for modern scientists.
Is Evolution a Theory or Fact?
The long-held belief for thousands of years was that the world and all of its organisms were created by one power. But, as science has advanced, there is clear evidence to argue against that.
The answer to this question is complicated because evolution is both fact and theory. According to the National Center for Science Education , scientific understanding needs both theories and facts. There is proof that organisms have changed or evolved over time, and scientists now have the means to study and identify how those changes happen.
Read More : 7 Things You May Not Know About Charles Darwin
What Does CRISPR Stand For?
According to the National Human Genome Research Institute, CRISPR stands for Clustered Regularly Interspaced Short Palindromic Repeats. Researchers use this technology to modify the DNA of a living organism.
Who Discovered CRISPR?
There are several people involved and decades of research into the discovery of CRISPR . These researchers include Yoshizumi Ishino, Francisco Mojica, and the duo who recently won the Nobel Prize in Chemistry for CRISPR, Jennifer Doudna and Emmanuelle Charpentier.
What Is CRISPR?
CRISPR is a technology that can edit genes or even turn a gene “on” or “off.” Researchers have described CRISPR as a molecular scissors that clips apart DNA, then replaces, deletes, or modifies genes. According to a 2018 study, scientists can use this technology to help replace certain genes that may cause diseases such as cancer or heritable diseases like Duchenne muscular dystrophy — a degenerative disorder that can cause premature death.
How Does CRISPR Work?
In short, scientists use CRISPR technology to find specific pieces of DNA inside of a cell. Scientists then alter that piece of DNA or replace it with a different DNA sequence. CRISPR technology also ensures that the changed gene passes on to the next offspring through gene drive.
Read More: CRISPR Gene-Editing Technology Enters the Body — and Space
This article was originally published on Oct. 22, 2021 and has since been updated with new information from the Discover staff.
- earth science
Already a subscriber?
Register or Log In
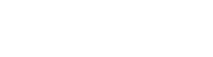
Keep reading for as low as $1.99!
Sign up for our weekly science updates.
Save up to 40% off the cover price when you subscribe to Discover magazine.

The Top Ten Scientific Discoveries of the Decade
Breakthroughs include measuring the true nature of the universe, finding new species of human ancestors, and unlocking new ways to fight disease
/https://tf-cmsv2-smithsonianmag-media.s3.amazonaws.com/accounts/headshot/bennett.jpg)
Jay Bennett
Former associate web editor, science.
:focal(800x601:801x602)/https://tf-cmsv2-smithsonianmag-media.s3.amazonaws.com/filer/d4/a8/d4a88985-6b69-41f0-85b3-4425e6c98596/science_rules5.jpg)
Millions of new scientific research papers are published every year , shedding light on everything from the evolution of stars to the ongoing impacts of climate change to the health benefits (or determents) of coffee to the tendency of your cat to ignore you . With so much research coming out every year, it can be difficult to know what is significant, what is interesting but largely insignificant, and what is just plain bad science . But over the course of a decade, we can look back at some of the most important and awe-inspiring areas of research, often expressed in multiple findings and research papers that lead to a true proliferation of knowledge. Here are ten of the biggest strides made by scientists in the last ten years.
New Human Relatives
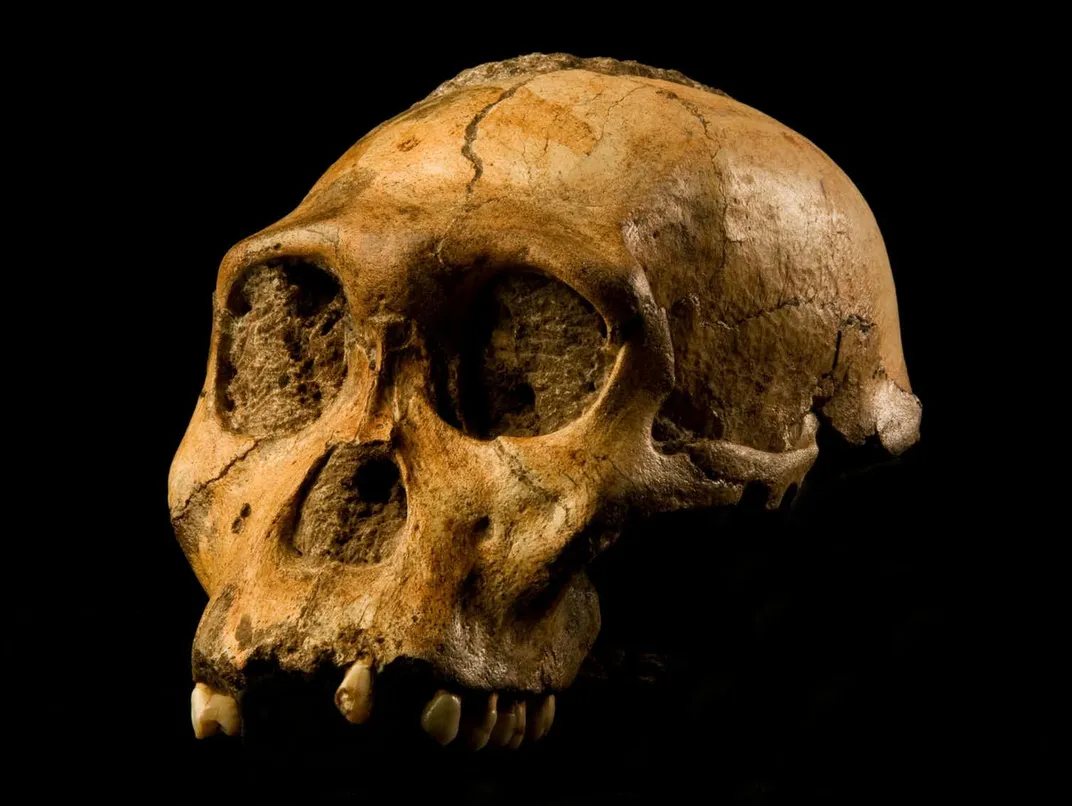
The human family tree expanded significantly in the past decade, with fossils of new hominin species discovered in Africa and the Philippines. The decade began with the discovery and identification of Australopithecus sediba , a hominin species that lived nearly two million years ago in present-day South Africa. Matthew Berger, the son of paleoanthropologist Lee Berger, stumbled upon the first fossil of the species, a right clavicle, in 2008, when he was only 9 years old. A team then unearthed more fossils from the individual, a young boy, including a well-preserved skull, and A. sediba was described by Lee Berger and colleagues in 2010 . The species represents a transitionary phase between the genus Australopithecus and the genus Homo , with some traits of the older primate group but a style of walking that resembled modern humans.
Also discovered in South Africa by a team led by Berger, Homo naledi lived much more recently, some 335,000 to 236,000 years ago, meaning it may have overlapped with our own species, Homo sapiens. The species, first discovered in the Rising Star Cave system in 2013 and described in 2015 , also had a mix of primitive and modern features, such as a small brain case (about one-third the size of Homo sapiens ) and a large body for the time, weighing approximately 100 pounds and standing up to five feet tall. The smaller Homo luzonensis (three to four feet tall) lived in the Philippines some 50,000 to 67,000 years ago , overlapping with several species of hominin. The first H. luzonensis fossils were originally identified as Homo sapiens, but a 2019 analysis determined that the bones belonged to an entirely unknown species.
These three major finds in the last ten years suggest that the bones of more species of ancient human relatives are likely hidden in the caves and sediment deposits of the world, waiting to be discovered.
Taking Measure of the Cosmos
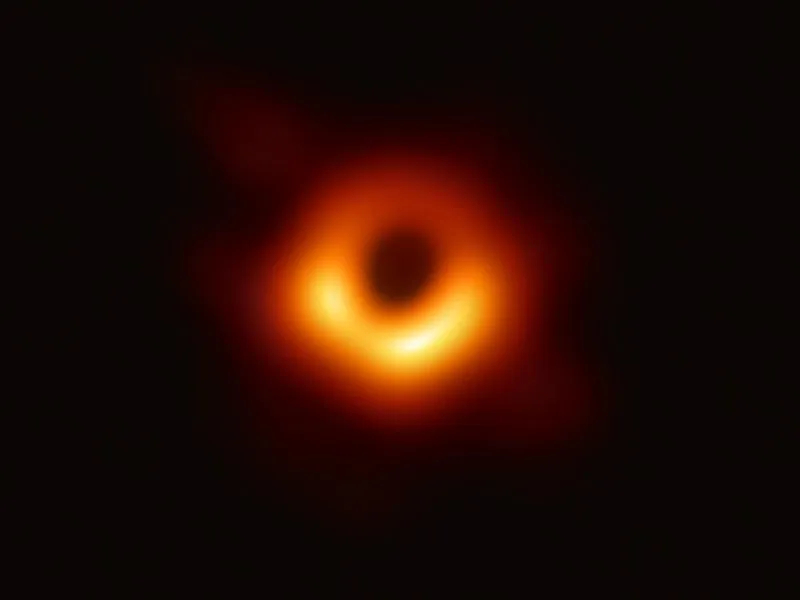
When Albert Einstein first published the general theory of relativity in 1915, he likely couldn’t have imagined that 100 years later, astronomers would test the theory’s predictions with some of the most sophisticated instruments ever built—and the theory would pass each test. General relativity describes the universe as a “fabric” of space-time that is warped by large masses. It’s this warping that causes gravity, rather than an internal property of mass as Isaac Newton thought.
One prediction of this model is that the acceleration of masses can cause “ripples” in space-time, or the propagation of gravitational waves. With a large enough mass, such as a black hole or a neutron star, these ripples may even be detected by astronomers on Earth. In September 2015, the LIGO and Virgo collaboration detected gravitational waves for the first time, propagating from a pair of merging black holes some 1.3 billion light-years away. Since then, the two instruments have detected several additional gravitational waves , including one from a two merging neutron stars.
Another prediction of general relativity—one that Einstein himself famously doubted —is the existence of black holes at all, or points of gravitational collapse in space with infinite density and infinitesimal volume. These objects consume all matter and light that strays too close, creating a disk of superheated material falling into the black hole. In 2017, the Event Horizon Telescope collaboration —a network of linked radio telescopes around the world—took observations that would later result in the first image of the environment around a black hole, released in April 2019 .
The Hottest Years on Record
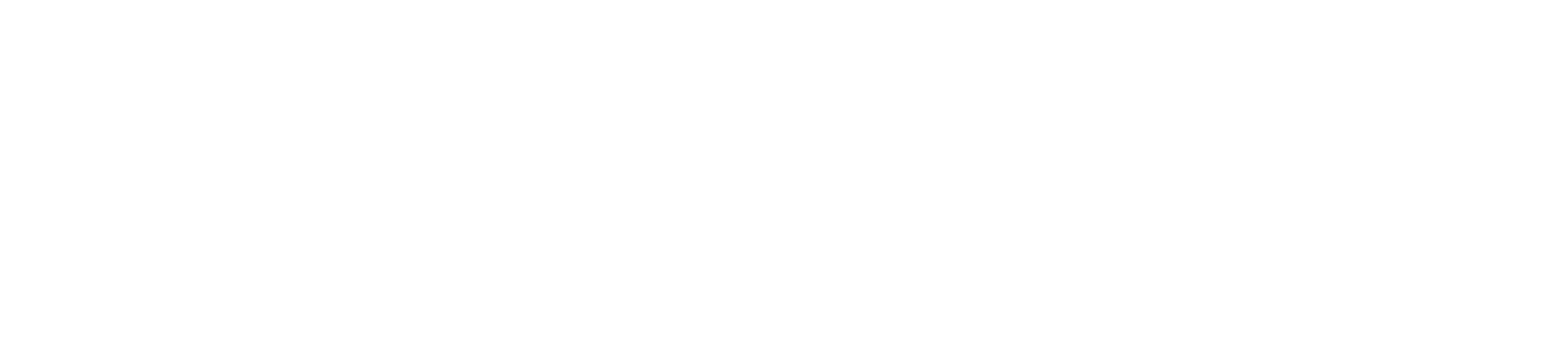
Scientists have been predicating the effects of burning coal and fossil fuels on the temperature of the planet for over 100 years. A 1912 issue of Popular Mechanics contains an article titled “ Remarkable Weather of 1911: The Effect of the Combustion of Coal on the Climate—What Scientists Predict for the Future ,” which has a caption that reads: “The furnaces of the world are now burning about 2,000,000,000 tons of coal a year. When this is burned, uniting with oxygen, it adds about 7,000,000,000 tons of carbon dioxide to the atmosphere yearly. This tends to make the air a more effective blanket for the earth and to raise its temperature. The effect may be considerable in a few centuries.”
Just one century later, and the effect is considerable indeed. Increased greenhouse gases in the atmosphere have produced hotter global temperatures, with the last five years (2014 to 2018) being the hottest years on record . 2016 was the hottest year since the National Oceanic and Atmospheric Administration (NOAA) started recording global temperature 139 years ago. The effects of this global change include more frequent and destructive wildfires, more common droughts, accelerating polar ice melt and increased storm surges. California is burning, Venice is flooding, urban heat deaths are on the rise, and countless coastal and island communities face an existential crisis—not to mention the ecological havoc wreaked by climate change, stifling the planet’s ability to pull carbon back out of the atmosphere.
In 2015, the United Nations Framework Convention on Climate Change (UNFCCC) reached a consensus on climate action, known as the Paris Agreement. The primary goal of the Paris Agreement is to limit global temperature increases to 1.5 degrees Celsius over pre-industrial levels . To achieve this goal, major societal transformations will be required, including replacing fossil fuels with clean energy such as wind, solar and nuclear; reforming agricultural practices to limit emissions and protect forested areas; and perhaps even building artificial means of pulling carbon dioxide out of the atmosphere.
Editing Genes
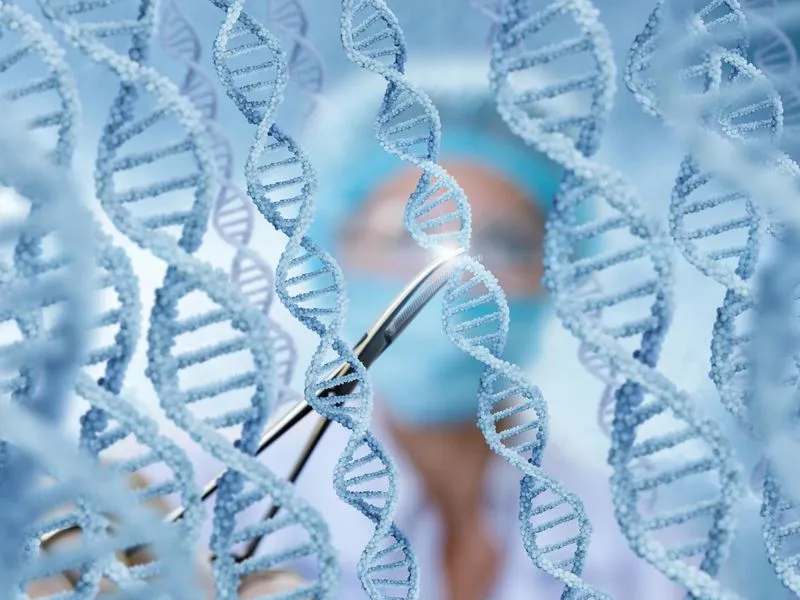
Ever since the double-helix structure of DNA was revealed in the early 1950s , scientists have hypothesized about the possibility of artificially modifying DNA to change the functions of an organism. The first approved gene therapy trial occurred in 1990, when a four-year-old girl had her own white blood cells removed, augmented with the genes that produce an enzyme called adenosine deaminase (ADA), and then reinjected into her body to treat ADA deficiency, a genetic condition that hampers the immune system’s ability to fight disease. The patient’s body began producing the ADA enzyme, but new white blood cells with the corrected gene were not produced, and she had to continue receiving injections .
Now, genetic engineering is more precise and available than ever before, thanks in large part to a new tool first used to modify eukaryotic cells (complex cells with a nucleus) in 2013 : CRISPR-Cas9. The gene editing tool works by locating a targeted section of DNA and “cutting” out that section with the Cas9 enzyme. An optional third step involves replacing the deleted section of DNA with new genetic material. The technique can be used for a wide range of applications, from increasing the muscle mass of livestock, to producing resistant and fruitful crops, to treating diseases like cancer by removing a patient’s immune system cells, modifying them to better fight a disease, and reinjecting them into the patient’s body.
In late 2018, Chinese researchers led by He Jiankui announced that they had used CRISPR-Cas9 to genetically modify human embryos, which were then transferred to a woman’s uterus and resulted in the birth of twin girls—the first gene-edited babies. The twins’ genomes were modified to make the girls more resistant to HIV, although the genetic alterations may have also resulted in unintended changes . The work was widely condemned by the scientific community as unethical and dangerous, revealing a need for stricter regulations for how these powerful new tools are used, particularly when it comes to changing the DNA of embryos and using those embryos to birth live children.
Mysteries of Other Worlds Revealed
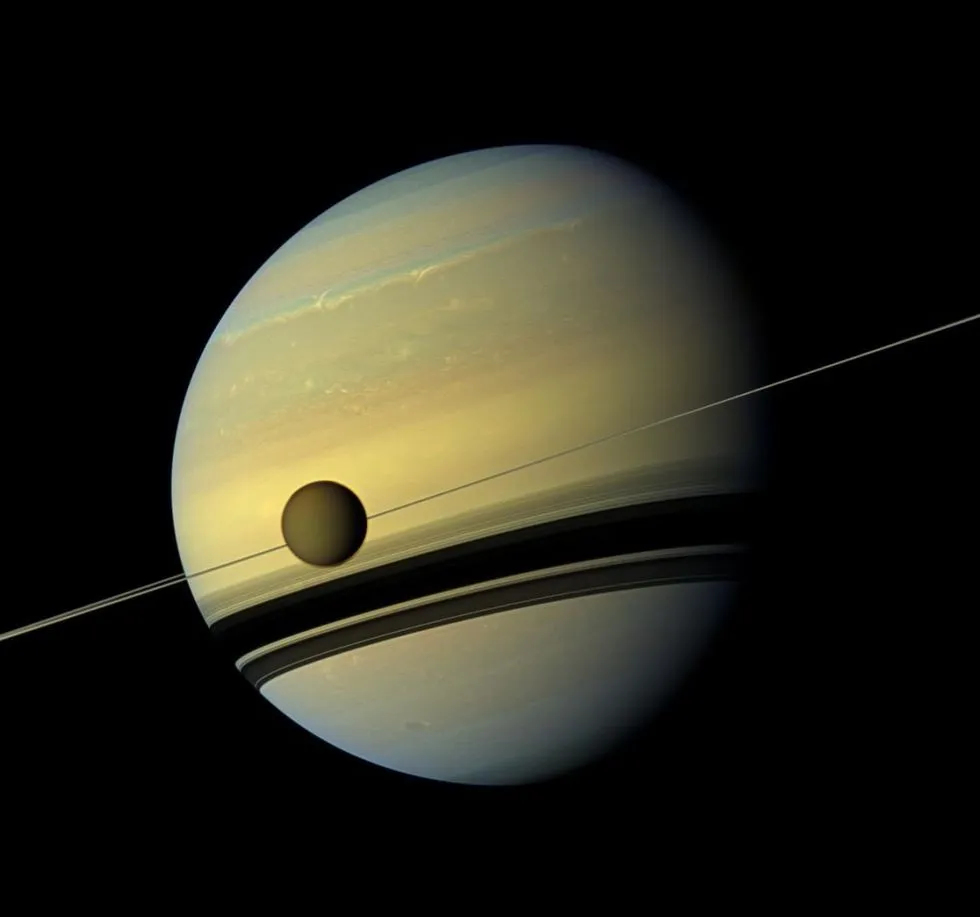
Spacecraft and telescopes have revealed a wealth of information about worlds beyond our own in the last decade. In 2015, the New Horizons probe made a close pass of Pluto, taking the first nearby observations of the dwarf planet and its moons. The spacecraft revealed a surprisingly dynamic and active world, with icy mountains reaching up to nearly 20,000 feet and shifting plains that are no more than 10 million years old—meaning the geology is constantly changing. The fact that Pluto—which is an average of 3.7 billion miles from the sun , about 40 times the distance of Earth—is so geologically active suggests that even cold, distant worlds could get enough energy to heat their interiors, possibly harboring subsurface liquid water or even life.
A bit closer to home, the Cassini spacecraft orbited Saturn for 13 years , ending its mission in September 2017 when NASA intentionally plunged the spacecraft into the atmosphere of Saturn so it would burn up rather than continue orbiting the planet once it had exhausted its fuel. During its mission, Cassini discovered the processes that feed Saturn’s rings , observed a global storm encircle the gas giant, mapped the large moon Titan and found some of the ingredients for life in the plumes of icy material erupting from the watery moon Enceladus. In 2016, a year before the end of the Cassini mission, the Juno spacecraft arrived at Jupiter, where it has been measuring the magnetic field and atmospheric dynamics of the largest planet in the solar system to help scientists understand how Jupiter—and everything else around the sun—originally formed.
In 2012, the Curiosity rover landed on Mars, where it has made several significant discoveries, including new evidence of past water on the red planet , the presence of organic molecules that could be related to life, and mysterious seasonal cycles of methane and oxygen that hint at a dynamic world beneath the surface. In 2018, the European Space Agency announced that ground-penetrating radar data from the Mars Express spacecraft provided strong evidence that a liquid reservoir of water exists underground near the Martian south pole .
Meanwhile, two space telescopes, Kepler and TESS, have discovered thousands of planets orbiting other stars. Kepler launched in 2009 and ended its mission in 2018, revealing mysterious and distant planets by measuring the decrease in light when they pass in front of their stars. These planets include hot Jupiters, which orbit close to their stars in just days or hours; mini Neptunes, which are between the size of Earth and Neptune and may be gas, liquid, solid or some combination; and super Earths, which are large rocky planets that astronomers hope to study for signs of life. TESS, which launched in 2018, continues the search as Kepler’s successor. The space telescope has already discovered hundreds of worlds , and it could find 10,000 or even 20,000 before the end of the mission.
Fossilized Pigments Reveal the Colors of Dinosaurs
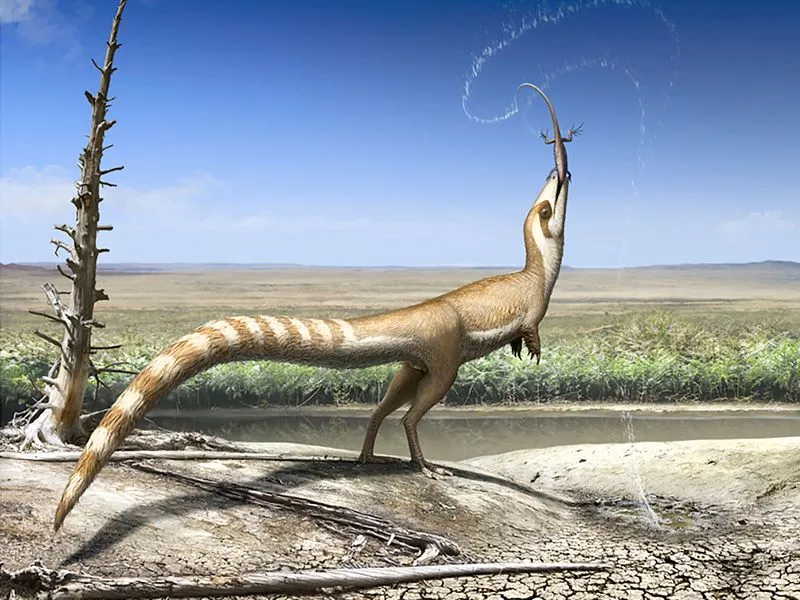
The decade began with a revolution in paleontology as scientists got their first look at the true colors of dinosaurs. First, in January 2010, an analysis of melanosomes—organelles that contain pigments—in the fossilized feathers of Sinosauropteryx , a dinosaur that lived in China some 120 to 125 million years ago, revealed that the prehistoric creature had “reddish-brown tones” and stripes along its tail . Shortly after, a full-body reconstruction revealed the colors of a small feathered dinosaur that lived some 160 million years ago , Anchiornis , which had black and white feathers on its body and a striking plume of red feathers on its head.
The study of fossilized pigments has continued to expose new information about prehistoric life, hinting at potential animal survival strategies by showing evidence of countershading and camouflage . In 2017, a remarkably well-preserved armored dinosaur which lived about 110 million years ago, Borealopelta , was found to have reddish-brown tones to help blend into the environment . This new ability to identify and study the colors of dinosaurs will continue to play an important role in paleontological research as scientists study the evolution of past life.
Redefining the Fundamental Unit of Mass
/https://tf-cmsv2-smithsonianmag-media.s3.amazonaws.com/filer/4d/4f/4d4f416e-6e5d-41fe-b6f7-3a79044a0aab/wattbalance_rsi_cover-1.jpg)
In November 2018, measurement scientists around the world voted to officially changed the definition of a kilogram , the fundamental unit of mass. Rather than basing the kilogram off of an object—a platinum-iridium alloy cylinder about the size of a golf ball—the new definition uses a constant of nature to set the unit of mass. The change replaced the last physical artifact used to define a unit of measure. (The meter bar was replaced in 1960 by a specific number of wavelengths of radiation from krypton, for example, and later updated to define a meter according to the distance light travels in a tiny fraction of a second .)
By using a sophisticated weighing machine known as a Kibble balance, scientists were able to precisely measure a kilogram according to the electromagnetic force required to hold it up. This electric measurement could then be expressed in terms of Planck’s constant, a number originally used by Max Planck to calculate bundles of energy coming from stars .
The kilogram was not the only unit of measure that was recently redefined. The changes to the International System of Units, which officially went into effect in May 2019 , also changed the definition for the ampere, the standard unit of electric current; the kelvin unit of temperature; and the mole, a unit of amount of substance used in chemistry. The changes to the kilogram and other units will allow more precise measurements for small amounts of material, such as pharmaceuticals, as well as give scientists around the world access to the fundamental units, rather than defining them according to objects that must be replicated and calibrated by a small number of labs.
First Ancient Human Genome Sequenced
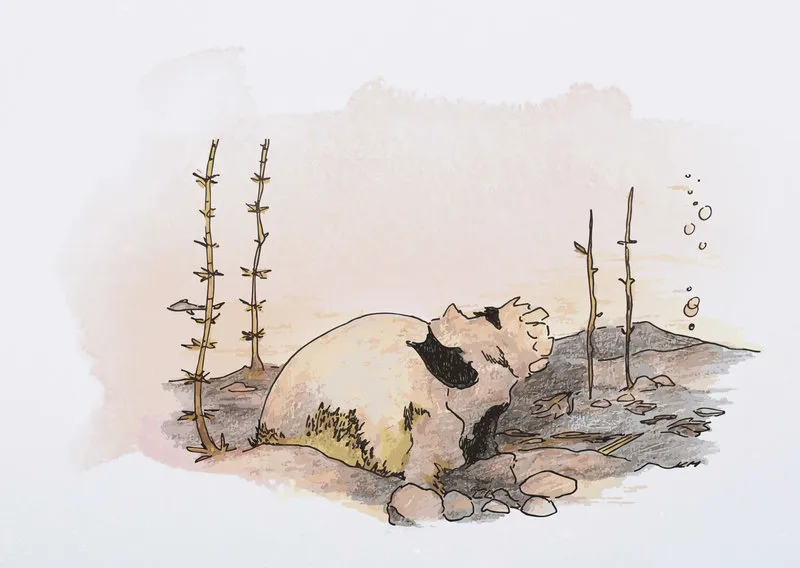
In 2010, scientists gained a new tool to study the ancient past and the people who inhabited it. Researchers used a hair preserved in permafrost to sequence the genome of a man who lived some 4,000 years ago in what is now Greenland , revealing the physical traits and even the blood type of a member of one of the first cultures to settle in that part of the world. The first nearly complete reconstruction of a genome from ancient DNA opened the door for anthropologists and geneticists to learn more about the cultures of the distant past than ever before.
Extracting ancient DNA is a major challenge. Even if genetic material such as hair or skin is preserved, it is often contaminated with the DNA of microbes from the environment, so sophisticated sequencing techniques must be used to isolate the ancient human’s DNA. More recently, scientists have used the petrous bone of the skull , a highly dense bone near the ear, to extract ancient DNA.
Thousands of ancient human genomes have been sequenced since the first success in 2010, revealing new details about the rise and fall of lost civilizations and the migrations of people around the globe . Studying ancient genomes has identified multiple waves of migration back and forth across the frozen Bering land bridge between Siberia and Alaska between 5,000 and 15,000 years ago. Recently, the genome of a young girl in modern Denmark was sequenced from a 5,700-year-old piece of birch tar used as chewing gum, which also contained her mouth microbes and bits of food from one of her last meals.
A Vaccine and New Treatments to Fight Ebola
/https://tf-cmsv2-smithsonianmag-media.s3.amazonaws.com/filer/94/69/946983e3-aa54-4eeb-add1-1eea8092071c/gettyimages-1183891526.jpg)
This decade included the worst outbreak of Ebola virus diseases in history. The epidemic is believed to have begun with a single case of an 18-month-old-boy in Guinea infected by bats in December 2013. The disease quickly spread to neighboring countries, reaching the capitals of Liberia and Sierra Leone by July 2014, providing an unprecedented opportunity for the transmission of the disease to a large number of people. Ebola virus compromises the immune system and can cause massive hemorrhaging and multiple organ failure. Two and a half years after the initial case, more than 28,600 people had been infected, resulting in at least 11,325 deaths, according to the CDC .
The epidemic prompted health officials to redouble their efforts to find an effective vaccine to fight Ebola. A vaccine known as Ervebo, made by the pharmaceutical company Merck, was tested in a clinical trial in Guinea performed toward the end of the outbreak in 2016 that proved the vaccine effective. Another Ebola outbreak was declared in the Democratic Republic of the Congo in August 2018, and the ongoing epidemic has spread to become the deadliest since the West Africa outbreak, with 3,366 reported cases and 2,227 deaths as of December 2019 . Ervebo has been used in the DRC to fight the outbreak on an expanded access or “compassionate use” basis . In November 2019, Ervebo was approved by the European Medicines Agency (EMA) , and a month later it was approved in the U.S. by the FDA .
In addition to a preventative vaccine, researchers have been seeking a cure for Ebola in patients who have already been infected by the disease. Two treatments, which involve a one-time delivery of antibodies to prevent Ebola from infecting a patient’s cells, have recently shown promise in a clinical trial in the DRC . With a combination of vaccines and therapeutic treatments, healthcare officials hope to one day eradicate the viral infection for good .
CERN Detects the Higgs Boson
Over the past several decades, physicists have worked tirelessly to model the workings of the universe, developing what is known as the Standard Model. This model describes four basic interactions of matter, known as the fundamental forces . Two are familiar in everyday life: the gravitational force and the electromagnetic force. The other two, however, only exert their influence inside the nuclei of atoms: the strong nuclear force and the weak nuclear force.
Part of the Standard Model says that there is a universal quantum field that interacts with particles, giving them their masses. In the 1960s, theoretical physicists including François Englert and Peter Higgs described this field and its role in the Standard Model. It became known as the Higgs field, and according to the laws of quantum mechanics, all such fundamental fields should have an associated particle, which came to be known as the Higgs boson.
Decades later, in 2012, two teams using the Large Hadron Collider at CERN to conduct particle collisions reported the detection of a particle with the predicted mass of the Higgs boson, providing substantial evidence for the existence of the Higgs field and Higgs boson. In 2013, the Nobel Prize in Physics was awarded to Englert and Higgs “for the theoretical discovery of a mechanism that contributes to our understanding of the origin of mass of subatomic particles, and which recently was confirmed through the discovery of the predicted fundamental particle.” As physicists continue to refine the Standard Model, the function and discovery of the Higgs boson will remain a fundamental part of how all matter gets its mass, and therefore, how any matter exists at all.
Get the latest Science stories in your inbox.
/https://tf-cmsv2-smithsonianmag-media.s3.amazonaws.com/accounts/headshot/bennett.jpg)
Jay Bennett | | READ MORE
Jay Bennett was the associate web editor, science, for Smithsonian .

- Table of Contents
- Random Entry
- Chronological
- Editorial Information
- About the SEP
- Editorial Board
- How to Cite the SEP
- Special Characters
- Advanced Tools
- Support the SEP
- PDFs for SEP Friends
- Make a Donation
- SEPIA for Libraries
- Entry Contents
Bibliography
Academic tools.
- Friends PDF Preview
- Author and Citation Info
- Back to Top
Scientific Discovery
Scientific discovery is the process or product of successful scientific inquiry. Objects of discovery can be things, events, processes, causes, and properties as well as theories and hypotheses and their features (their explanatory power, for example). Most philosophical discussions of scientific discoveries focus on the generation of new hypotheses that fit or explain given data sets or allow for the derivation of testable consequences. Philosophical discussions of scientific discovery have been intricate and complex because the term “discovery” has been used in many different ways, both to refer to the outcome and to the procedure of inquiry. In the narrowest sense, the term “discovery” refers to the purported “eureka moment” of having a new insight. In the broadest sense, “discovery” is a synonym for “successful scientific endeavor” tout court. Some philosophical disputes about the nature of scientific discovery reflect these terminological variations.
Philosophical issues related to scientific discovery arise about the nature of human creativity, specifically about whether the “eureka moment” can be analyzed and about whether there are rules (algorithms, guidelines, or heuristics) according to which such a novel insight can be brought about. Philosophical issues also arise about the analysis and evaluation of heuristics, about the characteristics of hypotheses worthy of articulation and testing, and, on the meta-level, about the nature and scope of philosophical analysis itself. This essay describes the emergence and development of the philosophical problem of scientific discovery and surveys different philosophical approaches to understanding scientific discovery. In doing so, it also illuminates the meta-philosophical problems surrounding the debates, and, incidentally, the changing nature of philosophy of science.
1. Introduction
2. scientific inquiry as discovery, 3. elements of discovery, 4. pragmatic logics of discovery, 5. the distinction between the context of discovery and the context of justification, 6.1 discovery as abduction, 6.2 heuristic programming, 7. anomalies and the structure of discovery, 8.1 discoverability, 8.2 preliminary appraisal, 8.3 heuristic strategies, 9.1 kinds and features of creativity, 9.2 analogy, 9.3 mental models, 10. machine discovery, 11. social epistemology and discovery, 12. integrated approaches to knowledge generation, other internet resources, related entries.
Philosophical reflection on scientific discovery occurred in different phases. Prior to the 1930s, philosophers were mostly concerned with discoveries in the broad sense of the term, that is, with the analysis of successful scientific inquiry as a whole. Philosophical discussions focused on the question of whether there were any discernible patterns in the production of new knowledge. Because the concept of discovery did not have a specified meaning and was used in a very wide sense, almost all discussions of scientific method and practice could potentially be considered as early contributions to reflections on scientific discovery. In the course of the 18 th century, as philosophy of science and science gradually became two distinct endeavors with different audiences, the term “discovery” became a technical term in philosophical discussions. Different elements of scientific inquiry were specified. Most importantly, during the 19 th century, the generation of new knowledge came to be clearly and explicitly distinguished from its assessment, and thus the conditions for the narrower notion of discovery as the act or process of conceiving new ideas emerged. This distinction was encapsulated in the so-called “context distinction,” between the “context of discovery” and the “context of justification”.
Much of the discussion about scientific discovery in the 20 th century revolved around this distinction It was argued that conceiving a new idea is a non-rational process, a leap of insight that cannot be captured in specific instructions. Justification, by contrast, is a systematic process of applying evaluative criteria to knowledge claims. Advocates of the context distinction argued that philosophy of science is exclusively concerned with the context of justification. The assumption underlying this argument is that philosophy is a normative project; it determines norms for scientific practice. Given this assumption, only the justification of ideas, not their generation, can be the subject of philosophical (normative) analysis. Discovery, by contrast, can only be a topic for empirical study. By definition, the study of discovery is outside the scope of philosophy of science proper.
The introduction of the context distinction and the disciplinary distinction between empirical science studies and normative philosophy of science that was tied to it spawned meta-philosophical disputes. For a long time, philosophical debates about discovery were shaped by the notion that philosophical and empirical analyses are mutually exclusive. Some philosophers insisted, like their predecessors prior to the 1930s, that the philosopher’s tasks include the analysis of actual scientific practices and that scientific resources be used to address philosophical problems. They maintained that it is a legitimate task for philosophy of science to develop a theory of heuristics or problem solving. But this position was the minority view in philosophy of science until the last decades of the 20 th century. Philosophers of discovery were thus compelled to demonstrate that scientific discovery was in fact a legitimate part of philosophy of science. Philosophical reflections about the nature of scientific discovery had to be bolstered by meta-philosophical arguments about the nature and scope of philosophy of science.
Today, however, there is wide agreement that philosophy and empirical research are not mutually exclusive. Not only do empirical studies of actual scientific discoveries in past and present inform philosophical thought about the structure and cognitive mechanisms of discovery, but works in psychology, cognitive science, artificial intelligence and related fields have become integral parts of philosophical analyses of the processes and conditions of the generation of new knowledge. Social epistemology has opened up another perspective on scientific discovery, reconceptualizing knowledge generation as group process.
Prior to the 19 th century, the term “discovery” was used broadly to refer to a new finding, such as a new cure, an unknown territory, an improvement of an instrument, or a new method of measuring longitude. One strand of the discussion about discovery dating back to ancient times concerns the method of analysis as the method of discovery in mathematics and geometry, and, by extension, in philosophy and scientific inquiry. Following the analytic method, we seek to find or discover something – the “thing sought,” which could be a theorem, a solution to a geometrical problem, or a cause – by analyzing it. In the ancient Greek context, analytic methods in mathematics, geometry, and philosophy were not clearly separated; the notion of finding or discovering things by analysis was relevant in all these fields.
In the ensuing centuries, several natural and experimental philosophers, including Avicenna and Zabarella, Bacon and Boyle, the authors of the Port-Royal Logic and Newton, and many others, expounded rules of reasoning and methods for arriving at new knowledge. The ancient notion of analysis still informed these rules and methods. Newton’s famous thirty-first query in the second edition of the Opticks outlines the role of analysis in discovery as follows: “As in Mathematicks, so in Natural Philosophy, the Investigation of difficult Things by the Method of Analysis, ought ever to precede the Method of Composition. This Analysis consists in making Experiments and Observations, and in drawing general Conclusions from them by Induction, and admitting of no Objections against the Conclusions, but such as are taken from Experiments, or other certain Truths … By this way of Analysis we may proceed from Compounds to Ingredients, and from Motions to the Forces producing them; and in general, from Effects to their Causes, and from particular Causes to more general ones, till the Argument end in the most general. This is the Method of Analysis” (Newton 1718, 380, see Koertge 1980, section VI). Early modern accounts of discovery captured knowledge-seeking practices in the study of living and non-living nature, ranging from astronomy and physics to medicine, chemistry, and agriculture. These rich accounts of scientific inquiry were often expounded to bolster particular theories about the nature of matter and natural forces and were not explicitly labeled “methods of discovery ”, yet they are, in fact, accounts of knowledge generation and proper scientific reasoning, covering topics such as the role of the senses in knowledge generation, observation and experimentation, analysis and synthesis, induction and deduction, hypotheses, probability, and certainty.
Bacon’s work is a prominent example. His view of the method of science as it is presented in the Novum Organum showed how best to arrive at knowledge about “form natures” (the most general properties of matter) via a systematic investigation of phenomenal natures. Bacon described how first to collect and organize natural phenomena and experimentally produced facts in tables, how to evaluate these lists, and how to refine the initial results with the help of further trials. Through these steps, the investigator would arrive at conclusions about the “form nature” that produces particular phenomenal natures. Bacon expounded the procedures of constructing and evaluating tables of presences and absences to underpin his matter theory. In addition, in his other writings, such as his natural history Sylva Sylvarum or his comprehensive work on human learning De Augmentis Scientiarium , Bacon exemplified the “art of discovery” with practical examples and discussions of strategies of inquiry.
Like Bacon and Newton, several other early modern authors advanced ideas about how to generate and secure empirical knowledge, what difficulties may arise in scientific inquiry, and how they could be overcome. The close connection between theories about matter and force and scientific methodologies that we find in early modern works was gradually severed. 18 th - and early 19 th -century authors on scientific method and logic cited early modern approaches mostly to model proper scientific practice and reasoning, often creatively modifying them ( section 3 ). Moreover, they developed the earlier methodologies of experimentation, observation, and reasoning into practical guidelines for discovering new phenomena and devising probable hypotheses about cause-effect relations.
It was common in 20 th -century philosophy of science to draw a sharp contrast between those early theories of scientific method and modern approaches. 20 th -century philosophers of science interpreted 17 th - and 18 th -century approaches as generative theories of scientific method. They function simultaneously as guides for acquiring new knowledge and as assessments of the knowledge thus obtained, whereby knowledge that is obtained “in the right way” is considered secure (Laudan 1980; Schaffner 1993: chapter 2). On this view, scientific methods are taken to have probative force (Nickles 1985). According to modern, “consequentialist” theories, propositions must be established by comparing their consequences with observed and experimentally produced phenomena (Laudan 1980; Nickles 1985). It was further argued that, when consequentialist theories were on the rise, the two processes of generation and assessment of an idea or hypothesis became distinct, and the view that the merit of a new idea does not depend on the way in which it was arrived at became widely accepted.
More recent research in history of philosophy of science has shown, however, that there was no such sharp contrast. Consequentialist ideas were advanced throughout the 18 th century, and the early modern generative theories of scientific method and knowledge were more pragmatic than previously assumed. Early modern scholars did not assume that this procedure would lead to absolute certainty. One could only obtain moral certainty for the propositions thus secured.
During the 18 th and 19 th centuries, the different elements of discovery gradually became separated and discussed in more detail. Discussions concerned the nature of observations and experiments, the act of having an insight and the processes of articulating, developing, and testing the novel insight. Philosophical discussion focused on the question of whether and to what extent rules could be devised to guide each of these processes.
Numerous 19 th -century scholars contributed to these discussions, including Claude Bernard, Auguste Comte, George Gore, John Herschel, W. Stanley Jevons, Justus von Liebig, John Stuart Mill, and Charles Sanders Peirce, to name only a few. William Whewell’s work, especially the two volumes of Philosophy of the Inductive Sciences of 1840, is a noteworthy and, later, much discussed contribution to the philosophical debates about scientific discovery because he explicitly distinguished the creative moment or “happy thought” as he called it from other elements of scientific inquiry and because he offered a detailed analysis of the “discoverer’s induction”, i.e., the pursuit and evaluation of the new insight. Whewell’s approach is not unique, but for late 20 th -century philosophers of science, his comprehensive, historically informed philosophy of discovery became a point of orientation in the revival of interest in scientific discovery processes.
For Whewell, discovery comprised three elements: the happy thought, the articulation and development of that thought, and the testing or verification of it. His account was in part a description of the psychological makeup of the discoverer. For instance, he held that only geniuses could have those happy thoughts that are essential to discovery. In part, his account was an account of the methods by which happy thoughts are integrated into the system of knowledge. According to Whewell, the initial step in every discovery is what he called “some happy thought, of which we cannot trace the origin; some fortunate cast of intellect, rising above all rules. No maxims can be given which inevitably lead to discovery” (Whewell 1996 [1840]: 186). An “art of discovery” in the sense of a teachable and learnable skill does not exist according to Whewell. The happy thought builds on the known facts, but according to Whewell it is impossible to prescribe a method for having happy thoughts.
In this sense, happy thoughts are accidental. But in an important sense, scientific discoveries are not accidental. The happy thought is not a wild guess. Only the person whose mind is prepared to see things will actually notice them. The “previous condition of the intellect, and not the single fact, is really the main and peculiar cause of the success. The fact is merely the occasion by which the engine of discovery is brought into play sooner or later. It is, as I have elsewhere said, only the spark which discharges a gun already loaded and pointed; and there is little propriety in speaking of such an accident as the cause why the bullet hits its mark.” (Whewell 1996 [1840]: 189).
Having a happy thought is not yet a discovery, however. The second element of a scientific discovery consists in binding together—“colligating”, as Whewell called it—a set of facts by bringing them under a general conception. Not only does the colligation produce something new, but it also shows the previously known facts in a new light. Colligation involves, on the one hand, the specification of facts through systematic observation, measurements and experiment, and on the other hand, the clarification of ideas through the exposition of the definitions and axioms that are tacitly implied in those ideas. This process is extended and iterative. The scientists go back and forth between binding together the facts, clarifying the idea, rendering the facts more exact, and so forth.
The final part of the discovery is the verification of the colligation involving the happy thought. This means, first and foremost, that the outcome of the colligation must be sufficient to explain the data at hand. Verification also involves judging the predictive power, simplicity, and “consilience” of the outcome of the colligation. “Consilience” refers to a higher range of generality (broader applicability) of the theory (the articulated and clarified happy thought) that the actual colligation produced. Whewell’s account of discovery is not a deductivist system. It is essential that the outcome of the colligation be inferable from the data prior to any testing (Snyder 1997).
Whewell’s theory of discovery clearly separates three elements: the non-analyzable happy thought or eureka moment; the process of colligation which includes the clarification and explication of facts and ideas; and the verification of the outcome of the colligation. His position that the philosophy of discovery cannot prescribe how to think happy thoughts has been a key element of 20 th -century philosophical reflection on discovery. In contrast to many 20 th -century approaches, Whewell’s philosophical conception of discovery also comprises the processes by which the happy thoughts are articulated. Similarly, the process of verification is an integral part of discovery. The procedures of articulation and test are both analyzable according to Whewell, and his conception of colligation and verification serve as guidelines for how the discoverer should proceed. To verify a hypothesis, the investigator needs to show that it accounts for the known facts, that it foretells new, previously unobserved phenomena, and that it can explain and predict phenomena which are explained and predicted by a hypothesis that was obtained through an independent happy thought-cum-colligation (Ducasse 1951).
Whewell’s conceptualization of scientific discovery offers a useful framework for mapping the philosophical debates about discovery and for identifying major issues of concern in 20 th -century philosophical debates. Until the late 20 th century, most philosophers operated with a notion of discovery that is narrower than Whewell’s. In more recent treatments of discovery, however, the scope of the term “discovery” is limited to either the first of these elements, the “happy thought”, or to the happy thought and its initial articulation. In the narrower conception, what Whewell called “verification” is not part of discovery proper. Secondly, until the late 20 th century, there was wide agreement that the eureka moment, narrowly construed, is an unanalyzable, even mysterious leap of insight. The main disagreements concerned the question of whether the process of developing a hypothesis (the “colligation” in Whewell’s terms) is, or is not, a part of discovery proper – and if it is, whether and how this process is guided by rules. Much of the controversies in the 20 th century about the possibility of a philosophy of discovery can be understood against the background of the disagreement about whether the process of discovery does or does not include the articulation and development of a novel thought. Philosophers also disagreed on the issue of whether it is a philosophical task to explicate these rules.
In early 20 th -century logical empiricism, the view that discovery is or at least crucially involves a non-analyzable creative act of a gifted genius was widespread. Alternative conceptions of discovery especially in the pragmatist tradition emphasize that discovery is an extended process, i.e., that the discovery process includes the reasoning processes through which a new insight is articulated and further developed.
In the pragmatist tradition, the term “logic” is used in the broad sense to refer to strategies of human reasoning and inquiry. While the reasoning involved does not proceed according to the principles of demonstrative logic, it is systematic enough to deserve the label “logical”. Proponents of this view argued that traditional (here: syllogistic) logic is an inadequate model of scientific discovery because it misrepresents the process of knowledge generation as grossly as the notion of an “aha moment”.
Early 20 th -century pragmatic logics of discovery can best be described as comprehensive theories of the mental and physical-practical operations involved in knowledge generation, as theories of “how we think” (Dewey 1910). Among the mental operations are classification, determination of what is relevant to an inquiry, and the conditions of communication of meaning; among the physical operations are observation and (laboratory) experiments. These features of scientific discovery are either not or only insufficiently represented by traditional syllogistic logic (Schiller 1917: 236–7).
Philosophers advocating this approach agree that the logic of discovery should be characterized as a set of heuristic principles rather than as a process of applying inductive or deductive logic to a set of propositions. These heuristic principles are not understood to show the path to secure knowledge. Heuristic principles are suggestive rather than demonstrative (Carmichael 1922, 1930). One recurrent feature in these accounts of the reasoning strategies leading to new ideas is analogical reasoning (Schiller 1917; Benjamin 1934, see also section 9.2 .). However, in academic philosophy of science, endeavors to develop more systematically the heuristics guiding discovery processes were soon eclipsed by the advance of the distinction between contexts of discovery and justification.
The distinction between “context of discovery” and “context of justification” dominated and shaped the discussions about discovery in 20 th -century philosophy of science. The context distinction marks the distinction between the generation of a new idea or hypothesis and the defense (test, verification) of it. As the previous sections have shown, the distinction among different elements of scientific inquiry has a long history but in the first half of the 20 th century, the distinction between the different features of scientific inquiry turned into a powerful demarcation criterion between “genuine” philosophy and other fields of science studies, which became potent in philosophy of science. The boundary between context of discovery (the de facto thinking processes) and context of justification (the de jure defense of the correctness of these thoughts) was now understood to determine the scope of philosophy of science, whereby philosophy of science is conceived as a normative endeavor. Advocates of the context distinction argue that the generation of a new idea is an intuitive, nonrational process; it cannot be subject to normative analysis. Therefore, the study of scientists’ actual thinking can only be the subject of psychology, sociology, and other empirical sciences. Philosophy of science, by contrast, is exclusively concerned with the context of justification.
The terms “context of discovery” and “context of justification” are often associated with Hans Reichenbach’s work. Reichenbach’s original conception of the context distinction is quite complex, however (Howard 2006; Richardson 2006). It does not map easily on to the disciplinary distinction mentioned above, because for Reichenbach, philosophy of science proper is partly descriptive. Reichenbach maintains that philosophy of science includes a description of knowledge as it really is. Descriptive philosophy of science reconstructs scientists’ thinking processes in such a way that logical analysis can be performed on them, and it thus prepares the ground for the evaluation of these thoughts (Reichenbach 1938: § 1). Discovery, by contrast, is the object of empirical—psychological, sociological—study. According to Reichenbach, the empirical study of discoveries shows that processes of discovery often correspond to the principle of induction, but this is simply a psychological fact (Reichenbach 1938: 403).
While the terms “context of discovery” and “context of justification” are widely used, there has been ample discussion about how the distinction should be drawn and what their philosophical significance is (c.f. Kordig 1978; Gutting 1980; Zahar 1983; Leplin 1987; Hoyningen-Huene 1987; Weber 2005: chapter 3; Schickore and Steinle 2006). Most commonly, the distinction is interpreted as a distinction between the process of conceiving a theory and the assessment of that theory, specifically the assessment of the theory’s epistemic support. This version of the distinction is not necessarily interpreted as a temporal distinction. In other words, it is not usually assumed that a theory is first fully developed and then assessed. Rather, generation and assessment are two different epistemic approaches to theory: the endeavor to articulate, flesh out, and develop its potential and the endeavor to assess its epistemic worth. Within the framework of the context distinction, there are two main ways of conceptualizing the process of conceiving a theory. The first option is to characterize the generation of new knowledge as an irrational act, a mysterious creative intuition, a “eureka moment”. The second option is to conceptualize the generation of new knowledge as an extended process that includes a creative act as well as some process of articulating and developing the creative idea.
Both of these accounts of knowledge generation served as starting points for arguments against the possibility of a philosophy of discovery. In line with the first option, philosophers have argued that neither is it possible to prescribe a logical method that produces new ideas nor is it possible to reconstruct logically the process of discovery. Only the process of testing is amenable to logical investigation. This objection to philosophies of discovery has been called the “discovery machine objection” (Curd 1980: 207). It is usually associated with Karl Popper’s Logic of Scientific Discovery .
The initial state, the act of conceiving or inventing a theory, seems to me neither to call for logical analysis not to be susceptible of it. The question how it happens that a new idea occurs to a man—whether it is a musical theme, a dramatic conflict, or a scientific theory—may be of great interest to empirical psychology; but it is irrelevant to the logical analysis of scientific knowledge. This latter is concerned not with questions of fact (Kant’s quid facti ?) , but only with questions of justification or validity (Kant’s quid juris ?) . Its questions are of the following kind. Can a statement be justified? And if so, how? Is it testable? Is it logically dependent on certain other statements? Or does it perhaps contradict them? […]Accordingly I shall distinguish sharply between the process of conceiving a new idea, and the methods and results of examining it logically. As to the task of the logic of knowledge—in contradistinction to the psychology of knowledge—I shall proceed on the assumption that it consists solely in investigating the methods employed in those systematic tests to which every new idea must be subjected if it is to be seriously entertained. (Popper 2002 [1934/1959]: 7–8)
With respect to the second way of conceptualizing knowledge generation, many philosophers argue in a similar fashion that because the process of discovery involves an irrational, intuitive process, which cannot be examined logically, a logic of discovery cannot be construed. Other philosophers turn against the philosophy of discovery even though they explicitly acknowledge that discovery is an extended, reasoned process. They present a meta-philosophical objection argument, arguing that a theory of articulating and developing ideas is not a philosophical but a psychological or sociological theory. In this perspective, “discovery” is understood as a retrospective label, which is attributed as a sign of accomplishment to some scientific endeavors. Sociological theories acknowledge that discovery is a collective achievement and the outcome of a process of negotiation through which “discovery stories” are constructed and certain knowledge claims are granted discovery status (Brannigan 1981; Schaffer 1986, 1994).
The impact of the context distinction on 20 th -century studies of scientific discovery and on philosophy of science more generally can hardly be overestimated. The view that the process of discovery (however construed) is outside the scope of philosophy of science proper was widely shared amongst philosophers of science for most of the 20 th century. The last section shows that there were some attempts to develop logics of discovery in the 1920s and 1930s, especially in the pragmatist tradition. But for several decades, the context distinction dictated what philosophy of science should be about and how it should proceed. The dominant view was that theories of mental operations or heuristics had no place in philosophy of science and that, therefore, discovery was not a legitimate topic for philosophy of science. Until the last decades of the 20 th century, there were few attempts to challenge the disciplinary distinction tied to the context distinction. Only during the 1970s did the interest in philosophical approaches to discovery begin to increase again. But the context distinction remained a challenge for philosophies of discovery.
There are several lines of response to the disciplinary distinction tied to the context distinction. Each of these lines of response opens a philosophical perspective on discovery. Each proceeds on the assumption that philosophy of science may legitimately include some form of analysis of actual reasoning patterns as well as information from empirical sciences such as cognitive science, psychology, and sociology. All of these responses reject the idea that discovery is nothing but a mystical event. Discovery is conceived as an analyzable reasoning process, not just as a creative leap by which novel ideas spring into being fully formed. All of these responses agree that the procedures and methods for arriving at new hypotheses and ideas are no guarantee that the hypothesis or idea that is thus formed is necessarily the best or the correct one. Nonetheless, it is the task of philosophy of science to provide rules for making this process better. All of these responses can be described as theories of problem solving, whose ultimate goal is to make the generation of new ideas and theories more efficient.
But the different approaches to scientific discovery employ different terminologies. In particular, the term “logic” of discovery is sometimes used in a narrow sense and sometimes broadly understood. In the narrow sense, “logic” of discovery is understood to refer to a set of formal, generally applicable rules by which novel ideas can be mechanically derived from existing data. In the broad sense, “logic” of discovery refers to the schematic representation of reasoning procedures. “Logical” is just another term for “rational”. Moreover, while each of these responses combines philosophical analyses of scientific discovery with empirical research on actual human cognition, different sets of resources are mobilized, ranging from AI research and cognitive science to historical studies of problem-solving procedures. Also, the responses parse the process of scientific inquiry differently. Often, scientific inquiry is regarded as having two aspects, viz. generation and assessments of new ideas. At times, however, scientific inquiry is regarded as having three aspects, namely generation, pursuit or articulation, and assessment of knowledge. In the latter framework, the label “discovery” is sometimes used to refer just to generation and sometimes to refer to both generation and pursuit.
One response to the challenge of the context distinction draws on a broad understanding of the term “logic” to argue that we cannot but admit a general, domain-neutral logic if we do not want to assume that the success of science is a miracle (Jantzen 2016) and that a logic of scientific discovery can be developed ( section 6 ). Another response, drawing on a narrow understanding of the term “logic”, is to concede that there is no logic of discovery, i.e., no algorithm for generating new knowledge, but that the process of discovery follows an identifiable, analyzable pattern ( section 7 ).
Others argue that discovery is governed by a methodology . The methodology of discovery is a legitimate topic for philosophical analysis ( section 8 ). Yet another response assumes that discovery is or at least involves a creative act. Drawing on resources from cognitive science, neuroscience, computational research, and environmental and social psychology, philosophers have sought to demystify the cognitive processes involved in the generation of new ideas. Philosophers who take this approach argue that scientific creativity is amenable to philosophical analysis ( section 9.1 ).
All these responses assume that there is more to discovery than a eureka moment. Discovery comprises processes of articulating, developing, and assessing the creative thought, as well as the scientific community’s adjudication of what does, and does not, count as “discovery” (Arabatzis 1996). These are the processes that can be examined with the tools of philosophical analysis, augmented by input from other fields of science studies such as sociology, history, or cognitive science.
6. Logics of discovery after the context distinction
One way of responding to the demarcation criterion described above is to argue that discovery is a topic for philosophy of science because it is a logical process after all. Advocates of this approach to the logic of discovery usually accept the overall distinction between the two processes of conceiving and testing a hypothesis. They also agree that it is impossible to put together a manual that provides a formal, mechanical procedure through which innovative concepts or hypotheses can be derived: There is no discovery machine. But they reject the view that the process of conceiving a theory is a creative act, a mysterious guess, a hunch, a more or less instantaneous and random process. Instead, they insist that both conceiving and testing hypotheses are processes of reasoning and systematic inference, that both of these processes can be represented schematically, and that it is possible to distinguish better and worse paths to new knowledge.
This line of argument has much in common with the logics of discovery described in section 4 above but it is now explicitly pitched against the disciplinary distinction tied to the context distinction. There are two main ways of developing this argument. The first is to conceive of discovery in terms of abductive reasoning ( section 6.1 ). The second is to conceive of discovery in terms of problem-solving algorithms, whereby heuristic rules aid the processing of available data and enhance the success in finding solutions to problems ( section 6.2 ). Both lines of argument rely on a broad conception of logic, whereby the “logic” of discovery amounts to a schematic account of the reasoning processes involved in knowledge generation.
One argument, elaborated prominently by Norwood R. Hanson, is that the act of discovery—here, the act of suggesting a new hypothesis—follows a distinctive logical pattern, which is different from both inductive logic and the logic of hypothetico-deductive reasoning. The special logic of discovery is the logic of abductive or “retroductive” inferences (Hanson 1958). The argument that it is through an act of abductive inferences that plausible, promising scientific hypotheses are devised goes back to C.S. Peirce. This version of the logic of discovery characterizes reasoning processes that take place before a new hypothesis is ultimately justified. The abductive mode of reasoning that leads to plausible hypotheses is conceptualized as an inference beginning with data or, more specifically, with surprising or anomalous phenomena.
In this view, discovery is primarily a process of explaining anomalies or surprising, astonishing phenomena. The scientists’ reasoning proceeds abductively from an anomaly to an explanatory hypothesis in light of which the phenomena would no longer be surprising or anomalous. The outcome of this reasoning process is not one single specific hypothesis but the delineation of a type of hypotheses that is worthy of further attention (Hanson 1965: 64). According to Hanson, the abductive argument has the following schematic form (Hanson 1960: 104):
- Some surprising, astonishing phenomena p 1 , p 2 , p 3 … are encountered.
- But p 1 , p 2 , p 3 … would not be surprising were an hypothesis of H ’s type to obtain. They would follow as a matter of course from something like H and would be explained by it.
- Therefore there is good reason for elaborating an hypothesis of type H—for proposing it as a possible hypothesis from whose assumption p 1 , p 2 , p 3 … might be explained.
Drawing on the historical record, Hanson argues that several important discoveries were made relying on abductive reasoning, such as Kepler’s discovery of the elliptic orbit of Mars (Hanson 1958). It is now widely agreed, however, that Hanson’s reconstruction of the episode is not a historically adequate account of Kepler’s discovery (Lugg 1985). More importantly, while there is general agreement that abductive inferences are frequent in both everyday and scientific reasoning, these inferences are no longer considered as logical inferences. Even if one accepts Hanson’s schematic representation of the process of identifying plausible hypotheses, this process is a “logical” process only in the widest sense whereby the term “logical” is understood as synonymous with “rational”. Notably, some philosophers have even questioned the rationality of abductive inferences (Koehler 1991; Brem and Rips 2000).
Another argument against the above schema is that it is too permissive. There will be several hypotheses that are explanations for phenomena p 1 , p 2 , p 3 …, so the fact that a particular hypothesis explains the phenomena is not a decisive criterion for developing that hypothesis (Harman 1965; see also Blackwell 1969). Additional criteria are required to evaluate the hypothesis yielded by abductive inferences.
Finally, it is worth noting that the schema of abductive reasoning does not explain the very act of conceiving a hypothesis or hypothesis-type. The processes by which a new idea is first articulated remain unanalyzed in the above schema. The schema focuses on the reasoning processes by which an exploratory hypothesis is assessed in terms of its merits and promise (Laudan 1980; Schaffner 1993).
In more recent work on abduction and discovery, two notions of abduction are sometimes distinguished: the common notion of abduction as inference to the best explanation (selective abduction) and creative abduction (Magnani 2000, 2009). Selective abduction—the inference to the best explanation—involves selecting a hypothesis from a set of known hypotheses. Medical diagnosis exemplifies this kind of abduction. Creative abduction, by contrast, involves generating a new, plausible hypothesis. This happens, for instance, in medical research, when the notion of a new disease is articulated. However, it is still an open question whether this distinction can be drawn, or whether there is a more gradual transition from selecting an explanatory hypothesis from a familiar domain (selective abduction) to selecting a hypothesis that is slightly modified from the familiar set and to identifying a more drastically modified or altered assumption.
Another recent suggestion is to broaden Peirce’s original account of abduction and to include not only verbal information but also non-verbal mental representations, such as visual, auditory, or motor representations. In Thagard’s approach, representations are characterized as patterns of activity in mental populations (see also section 9.3 below). The advantage of the neural account of human reasoning is that it covers features such as the surprise that accompanies the generation of new insights or the visual and auditory representations that contribute to it. Surprise, for instance, could be characterized as resulting from rapid changes in activation of the node in a neural network representing the “surprising” element (Thagard and Stewart 2011). If all mental representations can be characterized as patterns of firing in neural populations, abduction can be analyzed as the combination or “convolution” (Thagard) of patterns of neural activity from disjoint or overlapping patterns of activity (Thagard 2010).
The concern with the logic of discovery has also motivated research on artificial intelligence at the intersection of philosophy of science and cognitive science. In this approach, scientific discovery is treated as a form of problem-solving activity (Simon 1973; see also Newell and Simon 1971), whereby the systematic aspects of problem solving are studied within an information-processing framework. The aim is to clarify with the help of computational tools the nature of the methods used to discover scientific hypotheses. These hypotheses are regarded as solutions to problems. Philosophers working in this tradition build computer programs employing methods of heuristic selective search (e.g., Langley et al. 1987). In computational heuristics, search programs can be described as searches for solutions in a so-called “problem space” in a certain domain. The problem space comprises all possible configurations in that domain (e.g., for chess problems, all possible arrangements of pieces on a board of chess). Each configuration is a “state” of the problem space. There are two special states, namely the goal state, i.e., the state to be reached, and the initial state, i.e., the configuration at the starting point from which the search begins. There are operators, which determine the moves that generate new states from the current state. There are path constraints, which limit the permitted moves. Problem solving is the process of searching for a solution of the problem of how to generate the goal state from an initial state. In principle, all states can be generated by applying the operators to the initial state, then to the resulting state, until the goal state is reached (Langley et al. 1987: chapter 9). A problem solution is a sequence of operations leading from the initial to the goal state.
The basic idea behind computational heuristics is that rules can be identified that serve as guidelines for finding a solution to a given problem quickly and efficiently by avoiding undesired states of the problem space. These rules are best described as rules of thumb. The aim of constructing a logic of discovery thus becomes the aim of constructing a heuristics for the efficient search for solutions to problems. The term “heuristic search” indicates that in contrast to algorithms, problem-solving procedures lead to results that are merely provisional and plausible. A solution is not guaranteed, but heuristic searches are advantageous because they are more efficient than exhaustive random trial and error searches. Insofar as it is possible to evaluate whether one set of heuristics is better—more efficacious—than another, the logic of discovery turns into a normative theory of discovery.
Arguably, because it is possible to reconstruct important scientific discovery processes with sets of computational heuristics, the scientific discovery process can be considered as a special case of the general mechanism of information processing. In this context, the term “logic” is not used in the narrow sense of a set of formal, generally applicable rules to draw inferences but again in a broad sense as a label for a set of procedural rules.
The computer programs that embody the principles of heuristic searches in scientific inquiry simulate the paths that scientists followed when they searched for new theoretical hypotheses. Computer programs such as BACON (Simon et al. 1981) and KEKADA (Kulkarni and Simon 1988) utilize sets of problem-solving heuristics to detect regularities in given data sets. The program would note, for instance, that the values of a dependent term are constant or that a set of values for a term x and a set of values for a term y are linearly related. It would thus “infer” that the dependent term always has that value or that a linear relation exists between x and y . These programs can “make discoveries” in the sense that they can simulate successful discoveries such as Kepler’s third law (BACON) or the Krebs cycle (KEKADA).
Computational theories of scientific discoveries have helped identify and clarify a number of problem-solving strategies. An example of such a strategy is heuristic means-ends analysis, which involves identifying specific differences between the present and the goal situation and searches for operators (processes that will change the situation) that are associated with the differences that were detected. Another important heuristic is to divide the problem into sub-problems and to begin solving the one with the smallest number of unknowns to be determined (Simon 1977). Computational approaches have also highlighted the extent to which the generation of new knowledge draws on existing knowledge that constrains the development of new hypotheses.
As accounts of scientific discoveries, the early computational heuristics have some limitations. Compared to the problem spaces given in computational heuristics, the complex problem spaces for scientific problems are often ill defined, and the relevant search space and goal state must be delineated before heuristic assumptions could be formulated (Bechtel and Richardson 1993: chapter 1). Because a computer program requires the data from actual experiments, the simulations cover only certain aspects of scientific discoveries; in particular, it cannot determine by itself which data is relevant, which data to relate and what form of law it should look for (Gillies 1996). However, as a consequence of the rise of so-called “deep learning” methods in data-intensive science, there is renewed philosophical interest in the question of whether machines can make discoveries ( section 10 ).
Many philosophers maintain that discovery is a legitimate topic for philosophy of science while abandoning the notion that there is a logic of discovery. One very influential approach is Thomas Kuhn’s analysis of the emergence of novel facts and theories (Kuhn 1970 [1962]: chapter 6). Kuhn identifies a general pattern of discovery as part of his account of scientific change. A discovery is not a simple act, but an extended, complex process, which culminates in paradigm changes. Paradigms are the symbolic generalizations, metaphysical commitments, values, and exemplars that are shared by a community of scientists and that guide the research of that community. Paradigm-based, normal science does not aim at novelty but instead at the development, extension, and articulation of accepted paradigms. A discovery begins with an anomaly, that is, with the recognition that the expectations induced by an established paradigm are being violated. The process of discovery involves several aspects: observations of an anomalous phenomenon, attempts to conceptualize it, and changes in the paradigm so that the anomaly can be accommodated.
It is the mark of success of normal science that it does not make transformative discoveries, and yet such discoveries come about as a consequence of normal, paradigm-guided science. The more detailed and the better developed a paradigm, the more precise are its predictions. The more precisely the researchers know what to expect, the better they are able to recognize anomalous results and violations of expectations:
novelty ordinarily emerges only for the man who, knowing with precision what he should expect, is able to recognize that something has gone wrong. Anomaly appears only against the background provided by the paradigm. (Kuhn 1970 [1962]: 65)
Drawing on several historical examples, Kuhn argues that it is usually impossible to identify the very moment when something was discovered or even the individual who made the discovery. Kuhn illustrates these points with the discovery of oxygen (see Kuhn 1970 [1962]: 53–56). Oxygen had not been discovered before 1774 and had been discovered by 1777. Even before 1774, Lavoisier had noticed that something was wrong with phlogiston theory, but he was unable to move forward. Two other investigators, C. W. Scheele and Joseph Priestley, independently identified a gas obtained from heating solid substances. But Scheele’s work remained unpublished until after 1777, and Priestley did not identify his substance as a new sort of gas. In 1777, Lavoisier presented the oxygen theory of combustion, which gave rise to fundamental reconceptualization of chemistry. But according to this theory as Lavoisier first presented it, oxygen was not a chemical element. It was an atomic “principle of acidity” and oxygen gas was a combination of that principle with caloric. According to Kuhn, all of these developments are part of the discovery of oxygen, but none of them can be singled out as “the” act of discovery.
In pre-paradigmatic periods or in times of paradigm crisis, theory-induced discoveries may happen. In these periods, scientists speculate and develop tentative theories, which may lead to novel expectations and experiments and observations to test whether these expectations can be confirmed. Even though no precise predictions can be made, phenomena that are thus uncovered are often not quite what had been expected. In these situations, the simultaneous exploration of the new phenomena and articulation of the tentative hypotheses together bring about discovery.
In cases like the discovery of oxygen, by contrast, which took place while a paradigm was already in place, the unexpected becomes apparent only slowly, with difficulty, and against some resistance. Only gradually do the anomalies become visible as such. It takes time for the investigators to recognize “both that something is and what it is” (Kuhn 1970 [1962]: 55). Eventually, a new paradigm becomes established and the anomalous phenomena become the expected phenomena.
Recent studies in cognitive neuroscience of brain activity during periods of conceptual change support Kuhn’s view that conceptual change is hard to achieve. These studies examine the neural processes that are involved in the recognition of anomalies and compare them with the brain activity involved in the processing of information that is consistent with preferred theories. The studies suggest that the two types of data are processed differently (Dunbar et al. 2007).
8. Methodologies of discovery
Advocates of the view that there are methodologies of discovery use the term “logic” in the narrow sense of an algorithmic procedure to generate new ideas. But like the AI-based theories of scientific discovery described in section 6 , methodologies of scientific discovery interpret the concept “discovery” as a label for an extended process of generating and articulating new ideas and often describe the process in terms of problem solving. In these approaches, the distinction between the contexts of discovery and the context of justification is challenged because the methodology of discovery is understood to play a justificatory role. Advocates of a methodology of discovery usually rely on a distinction between different justification procedures, justification involved in the process of generating new knowledge and justification involved in testing it. Consequential or “strong” justifications are methods of testing. The justification involved in discovery, by contrast, is conceived as generative (as opposed to consequential) justification ( section 8.1 ) or as weak (as opposed to strong) justification ( section 8.2 ). Again, some terminological ambiguity exists because according to some philosophers, there are three contexts, not two: Only the initial conception of a new idea (the creative act is the context of discovery proper, and between it and justification there exists a separate context of pursuit (Laudan 1980). But many advocates of methodologies of discovery regard the context of pursuit as an integral part of the process of justification. They retain the notion of two contexts and re-draw the boundaries between the contexts of discovery and justification as they were drawn in the early 20 th century.
The methodology of discovery has sometimes been characterized as a form of justification that is complementary to the methodology of testing (Nickles 1984, 1985, 1989). According to the methodology of testing, empirical support for a theory results from successfully testing the predictive consequences derived from that theory (and appropriate auxiliary assumptions). In light of this methodology, justification for a theory is “consequential justification,” the notion that a hypothesis is established if successful novel predictions are derived from the theory or claim. Generative justification complements consequential justification. Advocates of generative justification hold that there exists an important form of justification in science that involves reasoning to a claim from data or previously established results more generally.
One classic example for a generative methodology is the set of Newton’s rules for the study of natural philosophy. According to these rules, general propositions are established by deducing them from the phenomena. The notion of generative justification seeks to preserve the intuition behind classic conceptions of justification by deduction. Generative justification amounts to the rational reconstruction of the discovery path in order to establish its discoverability had the researchers known what is known now, regardless of how it was first thought of (Nickles 1985, 1989). The reconstruction demonstrates in hindsight that the claim could have been discovered in this manner had the necessary information and techniques been available. In other words, generative justification—justification as “discoverability” or “potential discovery”—justifies a knowledge claim by deriving it from results that are already established. While generative justification does not retrace exactly those steps of the actual discovery path that were actually taken, it is a better representation of scientists’ actual practices than consequential justification because scientists tend to construe new claims from available knowledge. Generative justification is a weaker version of the traditional ideal of justification by deduction from the phenomena. Justification by deduction from the phenomena is complete if a theory or claim is completely determined from what we already know. The demonstration of discoverability results from the successful derivation of a claim or theory from the most basic and most solidly established empirical information.
Discoverability as described in the previous paragraphs is a mode of justification. Like the testing of novel predictions derived from a hypothesis, generative justification begins when the phase of finding and articulating a hypothesis worthy of assessing is drawing to a close. Other approaches to the methodology of discovery are directly concerned with the procedures involved in devising new hypotheses. The argument in favor of this kind of methodology is that the procedures of devising new hypotheses already include elements of appraisal. These preliminary assessments have been termed “weak” evaluation procedures (Schaffner 1993). Weak evaluations are relevant during the process of devising a new hypothesis. They provide reasons for accepting a hypothesis as promising and worthy of further attention. Strong evaluations, by contrast, provide reasons for accepting a hypothesis as (approximately) true or confirmed. Both “generative” and “consequential” testing as discussed in the previous section are strong evaluation procedures. Strong evaluation procedures are rigorous and systematically organized according to the principles of hypothesis derivation or H-D testing. A methodology of preliminary appraisal, by contrast, articulates criteria for the evaluation of a hypothesis prior to rigorous derivation or testing. It aids the decision about whether to take that hypothesis seriously enough to develop it further and test it. For advocates of this version of the methodology of discovery, it is the task of philosophy of science to characterize sets of constraints and methodological rules guiding the complex process of prior-to-test evaluation of hypotheses.
In contrast to the computational approaches discussed above, strategies of preliminary appraisal are not regarded as subject-neutral but as specific to particular fields of study. Philosophers of biology, for instance, have developed a fine-grained framework to account for the generation and preliminary evaluation of biological mechanisms (Darden 2002; Craver 2002; Bechtel and Richardson 1993; Craver and Darden 2013). Some philosophers have suggested that the phase of preliminary appraisal be further divided into two phases, the phase of appraising and the phase of revising. According to Lindley Darden, the phases of generation, appraisal and revision of descriptions of mechanisms can be characterized as reasoning processes governed by reasoning strategies. Different reasoning strategies govern the different phases (Darden 1991, 2002; Craver 2002; Darden 2009). The generation of hypotheses about mechanisms, for instance, is governed by the strategy of “schema instantiation” (see Darden 2002). The discovery of the mechanism of protein synthesis involved the instantiation of an abstract schema for chemical reactions: reactant 1 + reactant 2 = product. The actual mechanism of protein synthesis was found through specification and modification of this schema.
Neither of these strategies is deemed necessary for discovery, and they are not prescriptions for biological research. Rather, these strategies are deemed sufficient for the discovery of mechanisms. The methodology of the discovery of mechanisms is an extrapolation from past episodes of research on mechanisms and the result of a synthesis of rational reconstructions of several of these historical episodes. The methodology of discovery is weakly normative in the sense that the strategies for the discovery of mechanisms that were successful in the past may prove useful in future biological research (Darden 2002).
As philosophers of science have again become more attuned to actual scientific practices, interest in heuristic strategies has also been revived. Many analysts now agree that discovery processes can be regarded as problem solving activities, whereby a discovery is a solution to a problem. Heuristics-based methodologies of discovery are neither purely subjective and intuitive nor algorithmic or formalizable; the point is that reasons can be given for pursuing one or the other problem-solving strategy. These rules are open and do not guarantee a solution to a problem when applied (Ippoliti 2018). On this view, scientific researchers are no longer seen as Kuhnian “puzzle solvers” but as problem solvers and decision makers in complex, variable, and changing environments (Wimsatt 2007).
Philosophers of discovery working in this tradition draw on a growing body of literature in cognitive psychology, management science, operations research, and economy on human reasoning and decision making in contexts with limited information, under time constraints, and with sub-optimal means (Gigerenzer & Sturm 2012). Heuristic strategies characterized in these studies, such as Gigerenzer’s “tools to theory heuristic” are then applied to understand scientific knowledge generation (Gigerenzer 1992, Nickles 2018). Other analysts specify heuristic strategies in a range of scientific fields, including climate science, neurobiology, and clinical medicine (Gramelsberger 2011, Schaffner 2008, Gillies 2018). Finally, in analytic epistemology, formal methods are developed to identify and assess distinct heuristic strategies currently in use, such as Bayesian reverse engineering in cognitive science (Zednik and Jäkel 2016).
As the literature on heuristics continues to grow, it has become clear that the term “heuristics” is itself used in a variety of different ways. (For a valuable taxonomy of meanings of “heuristic,” see Chow 2015, see also Ippoliti 2018.) Moreover, as in the context of earlier debates about computational heuristics, debates continue about the limitations of heuristics. The use of heuristics may come at a cost if heuristics introduce systematic biases (Wimsatt 2007). Some philosophers thus call for general principles for the evaluation of heuristic strategies (Hey 2016).
9. Cognitive perspectives on discovery
The approaches to scientific discovery presented in the previous sections focus on the adoption, articulation, and preliminary evaluation of ideas or hypotheses prior to rigorous testing, not on how a novel hypothesis or idea is first thought up. For a long time, the predominant view among philosophers of discovery was that the initial step of discovery is a mysterious intuitive leap of the human mind that cannot be analyzed further. More recent accounts of discovery informed by evolutionary biology also do not explicate how new ideas are formed. The generation of new ideas is akin to random, blind variations of thought processes, which have to be inspected by the critical mind and assessed as neutral, productive, or useless (Campbell 1960; see also Hull 1988), but the key processes by which new ideas are generated are left unanalyzed.
With the recent rapprochement among philosophy of mind, cognitive science and psychology and the increased integration of empirical research into philosophy of science, these processes have been submitted to closer analysis, and philosophical studies of creativity have seen a surge of interest (e.g. Paul & Kaufman 2014a). The distinctive feature of these studies is that they integrate philosophical analyses with empirical work from cognitive science, psychology, evolutionary biology, and computational neuroscience (Thagard 2012). Analysts have distinguished different kinds and different features of creative thinking and have examined certain features in depth, and from new angles. Recent philosophical research on creativity comprises conceptual analyses and integrated approaches based on the assumption that creativity can be analyzed and that empirical research can contribute to the analysis (Paul & Kaufman 2014b). Two key elements of the cognitive processes involved in creative thinking that have been in the focus of philosophical analysis are analogies ( section 9.2 ) and mental models ( section 9.3 ).
General definitions of creativity highlight novelty or originality and significance or value as distinctive features of a creative act or product (Sternberg & Lubart 1999, Kieran 2014, Paul & Kaufman 2014b, although see Hills & Bird 2019). Different kinds of creativity can be distinguished depending on whether the act or product is novel for a particular individual or entirely novel. Psychologist Margaret Boden distinguishes between psychological creativity (P-creativity) and historical creativity (H-creativity). P-creativity is a development that is new, surprising and important to the particular person who comes up with it. H-creativity, by contrast, is radically novel, surprising, and important—it is generated for the first time (Boden 2004). Further distinctions have been proposed, such as anthropological creativity (construed as a human condition) and metaphysical creativity, a radically new thought or action in the sense that it is unaccounted for by antecedents and available knowledge, and thus constitutes a radical break with the past (Kronfeldner 2009, drawing on Hausman 1984).
Psychological studies analyze the personality traits and creative individuals’ behavioral dispositions that are conducive to creative thinking. They suggest that creative scientists share certain distinct personality traits, including confidence, openness, dominance, independence, introversion, as well as arrogance and hostility. (For overviews of recent studies on personality traits of creative scientists, see Feist 1999, 2006: chapter 5).
Recent work on creativity in philosophy of mind and cognitive science offers substantive analyses of the cognitive and neural mechanisms involved in creative thinking (Abrams 2018, Minai et al 2022) and critical scrutiny of the romantic idea of genius creativity as something deeply mysterious (Blackburn 2014). Some of this research aims to characterize features that are common to all creative processes, such as Thagard and Stewart’s account according to which creativity results from combinations of representations (Thagard & Stewart 2011, but see Pasquale and Poirier 2016). Other research aims to identify the features that are distinctive of scientific creativity as opposed to other forms of creativity, such as artistic creativity or creative technological invention (Simonton 2014).
Many philosophers of science highlight the role of analogy in the development of new knowledge, whereby analogy is understood as a process of bringing ideas that are well understood in one domain to bear on a new domain (Thagard 1984; Holyoak and Thagard 1996). An important source for philosophical thought about analogy is Mary Hesse’s conception of models and analogies in theory construction and development. In this approach, analogies are similarities between different domains. Hesse introduces the distinction between positive, negative, and neutral analogies (Hesse 1966: 8). If we consider the relation between gas molecules and a model for gas, namely a collection of billiard balls in random motion, we will find properties that are common to both domains (positive analogy) as well as properties that can only be ascribed to the model but not to the target domain (negative analogy). There is a positive analogy between gas molecules and a collection of billiard balls because both the balls and the molecules move randomly. There is a negative analogy between the domains because billiard balls are colored, hard, and shiny but gas molecules do not have these properties. The most interesting properties are those properties of the model about which we do not know whether they are positive or negative analogies. This set of properties is the neutral analogy. These properties are the significant properties because they might lead to new insights about the less familiar domain. From our knowledge about the familiar billiard balls, we may be able to derive new predictions about the behavior of gas molecules, which we could then test.
Hesse offers a more detailed analysis of the structure of analogical reasoning through the distinction between horizontal and vertical analogies between domains. Horizontal analogies between two domains concern the sameness or similarity between properties of both domains. If we consider sound and light waves, there are similarities between them: sound echoes, light reflects; sound is loud, light is bright, both sound and light are detectable by our senses. There are also relations among the properties within one domain, such as the causal relation between sound and the loud tone we hear and, analogously, between physical light and the bright light we see. These analogies are vertical analogies. For Hesse, vertical analogies hold the key for the construction of new theories.
Analogies play several roles in science. Not only do they contribute to discovery but they also play a role in the development and evaluation of scientific theories. Current discussions about analogy and discovery have expanded and refined Hesse’s approach in various ways. Some philosophers have developed criteria for evaluating analogy arguments (Bartha 2010). Other work has identified highly significant analogies that were particularly fruitful for the advancement of science (Holyoak and Thagard 1996: 186–188; Thagard 1999: chapter 9). The majority of analysts explore the features of the cognitive mechanisms through which aspects of a familiar domain or source are applied to an unknown target domain in order to understand what is unknown. According to the influential multi-constraint theory of analogical reasoning developed by Holyoak and Thagard, the transfer processes involved in analogical reasoning (scientific and otherwise) are guided or constrained in three main ways: 1) by the direct similarity between the elements involved; 2) by the structural parallels between source and target domain; as well as 3) by the purposes of the investigators, i.e., the reasons why the analogy is considered. Discovery, the formulation of a new hypothesis, is one such purpose.
“In vivo” investigations of scientists reasoning in their laboratories have not only shown that analogical reasoning is a key component of scientific practice, but also that the distance between source and target depends on the purpose for which analogies are sought. Scientists trying to fix experimental problems draw analogies between targets and sources from highly similar domains. In contrast, scientists attempting to formulate new models or concepts draw analogies between less similar domains. Analogies between radically different domains, however, are rare (Dunbar 1997, 2001).
In current cognitive science, human cognition is often explored in terms of model-based reasoning. The starting point of this approach is the notion that much of human reasoning, including probabilistic and causal reasoning as well as problem solving takes place through mental modeling rather than through the application of logic or methodological criteria to a set of propositions (Johnson-Laird 1983; Magnani et al. 1999; Magnani and Nersessian 2002). In model-based reasoning, the mind constructs a structural representation of a real-world or imaginary situation and manipulates this structure. In this perspective, conceptual structures are viewed as models and conceptual innovation as constructing new models through various modeling operations. Analogical reasoning—analogical modeling—is regarded as one of three main forms of model-based reasoning that appear to be relevant for conceptual innovation in science. Besides analogical modeling, visual modeling and simulative modeling or thought experiments also play key roles (Nersessian 1992, 1999, 2009). These modeling practices are constructive in that they aid the development of novel mental models. The key elements of model-based reasoning are the call on knowledge of generative principles and constraints for physical models in a source domain and the use of various forms of abstraction. Conceptual innovation results from the creation of new concepts through processes that abstract and integrate source and target domains into new models (Nersessian 2009).
Some critics have argued that despite the large amount of work on the topic, the notion of mental model is not sufficiently clear. Thagard seeks to clarify the concept by characterizing mental models in terms of neural processes (Thagard 2010). In his approach, mental models are produced through complex patterns of neural firing, whereby the neurons and the interconnections between them are dynamic and changing. A pattern of firing neurons is a representation when there is a stable causal correlation between the pattern or activation and the thing that is represented. In this research, questions about the nature of model-based reasoning are transformed into questions about the brain mechanisms that produce mental representations.
The above sections again show that the study of scientific discovery integrates different approaches, combining conceptual analysis of processes of knowledge generation with empirical work on creativity, drawing heavily and explicitly on current research in psychology and cognitive science, and on in vivo laboratory observations, as well as brain imaging techniques (Kounios & Beeman 2009, Thagard & Stewart 2011).
Earlier critics of AI-based theories of scientific discoveries argued that a computer cannot devise new concepts but is confined to the concepts included in the given computer language (Hempel 1985: 119–120). It cannot design new experiments, instruments, or methods. Subsequent computational research on scientific discovery was driven by the motivation to contribute computational tools to aid scientists in their research (Addis et al. 2016). It appears that computational methods can be used to generate new results leading to refereed scientific publications in astrophysics, cancer research, ecology, and other fields (Langley 2000). However, the philosophical discussion has continued about the question of whether these methods really generate new knowledge or whether they merely speed up data processing. It is also still an open question whether data-intensive science is fundamentally different from traditional research, for instance regarding the status of hypothesis or theory in data-intensive research (Pietsch 2015).
In the wake of recent developments in machine learning, some older discussions about automated discovery have been revived. The availability of vastly improved computational tools and software for data analysis has stimulated new discussions about computer-generated discovery (see Leonelli 2020). It is largely uncontroversial that machine learning tools can aid discovery, for instance in research on antibiotics (Stokes et al, 2020). The notion of “robot scientist” is mostly used metaphorically, and the vision that human scientists may one day be replaced by computers – by successors of the laboratory automation systems “Adam” and “Eve”, allegedly the first “robot scientists” – is evoked in writings for broader audiences (see King et al. 2009, Williams et al. 2015, for popularized descriptions of these systems), although some interesting ethical challenges do arise from “superhuman AI” (see Russell 2021). It also appears that, on the notion that products of creative acts are both novel and valuable, AI systems should be called “creative,” an implication which not all analysts will find plausible (Boden 2014)
Philosophical analyses focus on various questions arising from the processes involving human-machine complexes. One issue relevant to the problem of scientific discovery arises from the opacity of machine learning. If machine learning indeed escapes human understanding, how can we be warranted to say that knowledge or understanding is generated by deep learning tools? Might we have reason to say that humans and machines are “co-developers” of knowledge (Tamaddoni-Nezhad et al. 2021)?
New perspectives on scientific discovery have also opened up in the context of social epistemology (see Goldman & O’Connor 2021). Social epistemology investigates knowledge production as a group process, specifically the epistemic effects of group composition in terms of cognitive diversity and unity and social interactions within groups or institutions such as testimony and trust, peer disagreement and critique, and group justification, among others. On this view, discovery is a collective achievement, and the task is to explore how assorted social-epistemic activities or practices have an impact on the knowledge generated by groups in question. There are obvious implications for debates about scientific discovery of recent research in the different branches of social epistemology. Social epistemologists have examined individual cognitive agents in their roles as group members (as providers of information or as critics) and the interactions among these members (Longino 2001), groups as aggregates of diverse agents, or the entire group as epistemic agent (e.g., Koons 2021, Dragos 2019).
Standpoint theory, for instance, explores the role of outsiders in knowledge generation, considering how the sociocultural structures and practices in which individuals are embedded aid or obstruct the generation of creative ideas. According to standpoint theorists, people with standpoint are politically aware and politically engaged people outside the mainstream. Because people with standpoint have different experiences and access to different domains of expertise than most members of a culture, they can draw on rich conceptual resources for creative thinking (Solomon 2007).
Social epistemologists examining groups as aggregates of agents consider to what extent diversity among group members is conducive to knowledge production and whether and to what extent beliefs and attitudes must be shared among group members to make collective knowledge possible (Bird 2014). This is still an open question. Some formal approaches to model the influence of diversity on knowledge generation suggest that cognitive diversity is beneficial to collective knowledge generation (Weisberg and Muldoon 2009), but others have criticized the model (Alexander et al (2015), see also Thoma (2015) and Poyhönen (2017) for further discussion).
This essay has illustrated that philosophy of discovery has come full circle. Philosophy of discovery has once again become a thriving field of philosophical study, now intersecting with, and drawing on philosophical and empirical studies of creative thinking, problem solving under uncertainty, collective knowledge production, and machine learning. Recent approaches to discovery are typically explicitly interdisciplinary and integrative, cutting across previous distinctions among hypothesis generation and theory building, data collection, assessment, and selection; as well as descriptive-analytic, historical, and normative perspectives (Danks & Ippoliti 2018, Michel 2021). The goal no longer is to provide one overarching account of scientific discovery but to produce multifaceted analyses of past and present activities of knowledge generation in all their complexity and heterogeneity that are illuminating to the non-scientist and the scientific researcher alike.
- Abraham, A. 2019, The Neuroscience of Creativity, Cambridge: Cambridge University Press.
- Addis, M., Sozou, P.D., Gobet, F. and Lane, P. R., 2016, “Computational scientific discovery and cognitive science theories”, in Mueller, V. C. (ed.) Computing and Philosophy , Springer, 83–87.
- Alexander, J., Himmelreich, J., and Thompson, C. 2015, Epistemic Landscapes, Optimal Search, and the Division of Cognitive Labor, Philosophy of Science 82: 424–453.
- Arabatzis, T. 1996, “Rethinking the ‘Discovery’ of the Electron,” Studies in History and Philosophy of Science Part B Studies In History and Philosophy of Modern Physics , 27: 405–435.
- Bartha, P., 2010, By Parallel Reasoning: The Construction and Evaluation of Analogical Arguments , New York: Oxford University Press.
- Bechtel, W. and R. Richardson, 1993, Discovering Complexity , Princeton: Princeton University Press.
- Benjamin, A.C., 1934, “The Mystery of Scientific Discovery ” Philosophy of Science , 1: 224–36.
- Bird, A. 2014, “When is There a Group that Knows? Distributed Cognition, Scientific Knowledge, and the Social Epistemic Subject”, in J. Lackey (ed.), Essays in Collective Epistemology , Oxford: Oxford University Press, 42–63.
- Blackburn, S. 2014, “Creativity and Not-So-Dumb Luck”, in Paul, E. S. and Kaufman, S. B. (eds.), The Philosophy of Creativity: New Essays , New York: Oxford Academic online edn. https://doi.org/10.1093/acprof:oso/9780199836963.003.0008.
- Blackwell, R.J., 1969, Discovery in the Physical Sciences , Notre Dame: University of Notre Dame Press.
- Boden, M.A., 2004, The Creative Mind: Myths and Mechanisms , London: Routledge.
- –––, 2014, “Creativity and Artificial Intelligence: A Contradiction in Terms?”, in Paul, E. S. and Kaufman, S. B. (eds.), The Philosophy of Creativity: New Essays (New York: Oxford Academic online edn., https://doi.org/10.1093/acprof:oso/9780199836963.003.0012 .
- Brannigan, A., 1981, The Social Basis of Scientific Discoveries , Cambridge: Cambridge University Press.
- Brem, S. and L.J. Rips, 2000, “Explanation and Evidence in Informal Argument”, Cognitive Science , 24: 573–604.
- Campbell, D., 1960, “Blind Variation and Selective Retention in Creative Thought as in Other Knowledge Processes”, Psychological Review , 67: 380–400.
- Carmichael, R.D., 1922, “The Logic of Discovery”, The Monist , 32: 569–608.
- –––, 1930, The Logic of Discovery , Chicago: Open Court.
- Chow, S. 2015, “Many Meanings of ‘Heuristic’”, British Journal for the Philosophy of Science , 66: 977–1016
- Craver, C.F., 2002, “Interlevel Experiments, Multilevel Mechanisms in the Neuroscience of Memory”, Philosophy of Science Supplement , 69: 83–97.
- Craver, C.F. and L. Darden, 2013, In Search of Mechanisms: Discoveries across the Life Sciences , Chicago: University of Chicago Press.
- Curd, M., 1980, “The Logic of Discovery: An Analysis of Three Approaches”, in T. Nickles (ed.) Scientific Discovery, Logic, and Rationality , Dordrecht: D. Reidel, 201–19.
- Danks, D. & Ippoliti, E. (eds.) 2018, Building Theories: Heuristics and Hypotheses in Sciences , Cham: Springer.
- Darden, L., 1991, Theory Change in Science: Strategies from Mendelian Genetics , New York: Oxford University Press.
- –––, 2002, “Strategies for Discovering Mechanisms: Schema Instantiation, Modular Subassembly, Forward/Backward Chaining”, Philosophy of Science , 69: S354-S65.
- –––, 2009, “Discovering Mechanisms in Molecular Biology: Finding and Fixing Incompleteness and Incorrectness”, in J. Meheus and T. Nickles (eds.), Models of Discovery and Creativity , Dordrecht: Springer, 43–55.
- Dewey, J. 1910, How We Think . Boston: D.C. Heath
- Dragos, C., 2019, “Groups Can Know How” American Philosophical Quarterly 56: 265–276
- Ducasse, C.J., 1951, “Whewell’s Philosophy of Scientific Discovery II”, The Philosophical Review , 60(2): 213–34.
- Dunbar, K., 1997, “How scientists think: On-line creativity and conceptual change in science”, in T.B. Ward, S.M. Smith, and J. Vaid (eds.), Conceptual Structures and Processes: Emergence, Discovery, and Change , Washington, DC: American Psychological Association Press, 461–493.
- –––, 2001, “The Analogical Paradox: Why Analogy is so Easy in Naturalistic Settings Yet so Difficult in Psychological Laboratories”, in D. Gentner, K.J. Holyoak, and B.N. Kokinov (eds.), The Analogical Mind: Perspectives from Cognitive Science , Cambridge, MA: MIT Press.
- Dunbar, K, J. Fugelsang, and C Stein, 2007, “Do Naïve Theories Ever Go Away? Using Brain and Behavior to Understand Changes in Concepts”, in M. Lovett and P. Shah (eds.), Thinking with Data: 33rd Carnegie Symposium on Cognition , Mahwah: Erlbaum, 193–205.
- Feist, G.J., 1999, “The Influence of Personality on Artistic and Scientific Creativity”, in R.J. Sternberg (ed.), Handbook of Creativity , New York: Cambridge University Press, 273–96.
- –––, 2006, The psychology of science and the origins of the scientific mind , New Haven: Yale University Press.
- Gillies D., 1996, Artificial intelligence and scientific method . Oxford: Oxford University Press.
- –––, 2018 “Discovering Cures in Medicine” in Danks, D. & Ippoliti, E. (eds.), Building Theories: Heuristics and Hypotheses in Sciences , Cham: Springer, 83–100.
- Goldman, Alvin & O’Connor, C., 2021, “Social Epistemology”, The Stanford Encyclopedia of Philosophy (Winter 2021 Edition), Edward N. Zalta (ed.), URL = <https://plato.stanford.edu/archives/win2021/entries/epistemology-social/>.
- Gramelsberger, G. 2011, “What Do Numerical (Climate) Models Really Represent?” Studies in History and Philosophy of Science 42: 296–302.
- Gutting, G., 1980, “Science as Discovery”, Revue internationale de philosophie , 131: 26–48.
- Hanson, N.R., 1958, Patterns of Discovery , Cambridge: Cambridge University Press.
- –––, 1960, “Is there a Logic of Scientific Discovery?”, Australasian Journal of Philosophy , 38: 91–106.
- –––, 1965, “Notes Toward a Logic of Discovery”, in R.J. Bernstein (ed.), Perspectives on Peirce. Critical Essays on Charles Sanders Peirce , New Haven and London: Yale University Press, 42–65.
- Harman, G.H., 1965, “The Inference to the Best Explanation”, Philosophical Review , 74.
- Hausman, C. R. 1984, A Discourse on Novelty and Creation , New York: SUNY Press.
- Hempel, C.G., 1985, “Thoughts in the Limitations of Discovery by Computer”, in K. Schaffner (ed.), Logic of Discovery and Diagnosis in Medicine , Berkeley: University of California Press, 115–22.
- Hesse, M., 1966, Models and Analogies in Science , Notre Dame: University of Notre Dame Press.
- Hey, S. 2016 “Heuristics and Meta-heuristics in Scientific Judgement”, British Journal for the Philosophy of Science , 67: 471–495
- Hills, A., Bird, A. 2019, “Against Creativity”, Philosophy and Phenomenological Research , 99: 694–713.
- Holyoak, K.J. and P. Thagard, 1996, Mental Leaps: Analogy in Creative Thought , Cambridge, MA: MIT Press.
- Howard, D., 2006, “Lost Wanderers in the Forest of Knowledge: Some Thoughts on the Discovery-Justification Distinction”, in J. Schickore and F. Steinle (eds.), Revisiting Discovery and Justification. Historical and Philosophical Perspectives on the Context Distinction , Dordrecht: Springer, 3–22.
- Hoyningen-Huene, P., 1987, “Context of Discovery and Context of Justification”, Studies in History and Philosophy of Science , 18: 501–15.
- Hull, D.L., 1988, Science as Practice: An Evolutionary Account of the Social and Conceptual Development of Science , Chicago: University of Chicago Press.
- Ippoliti, E. 2018, “Heuristic Logic. A Kernel” in Danks, D. & Ippoliti, E. (eds.) Building Theories: Heuristics and Hypotheses in Sciences , Cham: Springer, 191–212
- Jantzen, B.C., 2016, “Discovery without a ‘Logic’ would be a Miracle”, Synthese , 193: 3209–3238.
- Johnson-Laird, P., 1983, Mental Models , Cambridge: Cambridge University Press.
- Kieran, M., 2014, “Creativity as a Virtue of Character,” in E. Paul and S. B. Kaufman (eds.), The Philosophy of Creativity: New Essays . Oxford: Oxford University Press, 125–44
- King, R. D. et al. 2009, “The Automation of Science”, Science 324: 85–89.
- Koehler, D.J., 1991, “Explanation, Imagination, and Confidence in Judgment”, Psychological Bulletin , 110: 499–519.
- Koertge, N. 1980, “Analysis as a Method of Discovery during the Scientific Revolution” in Nickles, T. (ed.) Scientific Discovery, Logic, and Rationality vol. I, Dordrecht: Reidel, 139–157
- Koons, J.R. 2021, “Knowledge as a Collective Status”, Analytic Philosophy , https://doi.org/10.1111/phib.12224
- Kounios, J. and Beeman, M. 2009, “The Aha! Moment : The Cognitive Neuroscience of Insight”, Current Directions in Psychological Science , 18: 210–16.
- Kordig, C., 1978, “Discovery and Justification”, Philosophy of Science , 45: 110–17.
- Kronfeldner, M. 2009, “Creativity Naturalized”, The Philosophical Quarterly 59: 577–592.
- Kuhn, T.S., 1970 [1962], The Structure of Scientific Revolutions , 2 nd edition, Chicago: The University of Chicago Press; first edition, 1962.
- Kulkarni, D. and H.A. Simon, 1988, “The processes of scientific discovery: The strategy of experimentation”, Cognitive Science , 12: 139–76.
- Langley, P., 2000, “The Computational Support of Scientific Discovery”, International Journal of Human-Computer Studies , 53: 393–410.
- Langley, P., H.A. Simon, G.L. Bradshaw, and J.M. Zytkow, 1987, Scientific Discovery: Computational Explorations of the Creative Processes , Cambridge, MA: MIT Press.
- Laudan, L., 1980, “Why Was the Logic of Discovery Abandoned?” in T. Nickles (ed.), Scientific Discovery (Volume I), Dordrecht: D. Reidel, 173–83.
- Leonelli, S. 2020, “Scientific Research and Big Data”, The Stanford Encyclopedia of Philosophy (Summer 2020 Edition), Edward N. Zalta (ed.), URL = <https://plato.stanford.edu/archives/sum2020/entries/science-big-data/>
- Leplin, J., 1987, “The Bearing of Discovery on Justification”, Canadian Journal of Philosophy , 17: 805–14.
- Longino, H. 2001, The Fate of Knowledge , Princeton: Princeton University Press
- Lugg, A., 1985, “The Process of Discovery”, Philosophy of Science , 52: 207–20.
- Magnani, L., 2000, Abduction, Reason, and Science: Processes of Discovery and Explanation , Dordrecht: Kluwer.
- –––, 2009, “Creative Abduction and Hypothesis Withdrawal”, in J. Meheus and T. Nickles (eds.), Models of Discovery and Creativity , Dordrecht: Springer.
- Magnani, L. and N.J. Nersessian, 2002, Model-Based Reasoning: Science, Technology, and Values , Dordrecht: Kluwer.
- Magnani, L., N.J. Nersessian, and P. Thagard, 1999, Model-Based Reasoning in Scientific Discovery , Dordrecht: Kluwer.
- Michel, J. (ed.) 2021, Making Scientific Discoveries. Interdisciplinary Reflections , Brill | mentis.
- Minai, A., Doboli, S., Iyer, L. 2022 “Models of Creativity and Ideation: An Overview” in Ali A. Minai, Jared B. Kenworthy, Paul B. Paulus, Simona Doboli (eds.), Creativity and Innovation. Cognitive, Social, and Computational Approaches , Springer, 21–46.
- Nersessian, N.J., 1992, “How do scientists think? Capturing the dynamics of conceptual change in science”, in R. Giere (ed.), Cognitive Models of Science , Minneapolis: University of Minnesota Press, 3–45.
- –––, 1999, “Model-based reasoning in conceptual change”, in L. Magnani, N.J. Nersessian and P. Thagard (eds.), Model-Based Reasoning in Scientific Discovery , New York: Kluwer, 5–22.
- –––, 2009, “Conceptual Change: Creativity, Cognition, and Culture ” in J. Meheus and T. Nickles (eds.), Models of Discovery and Creativity , Dordrecht: Springer, 127–66.
- Newell, A. and H. A Simon, 1971, “Human Problem Solving: The State of the Theory in 1970”, American Psychologist , 26: 145–59.
- Newton, I. 1718, Opticks; or, A Treatise of the Reflections, Inflections and Colours of Light , London: Printed for W. and J. Innys, Printers to the Royal Society.
- Nickles, T., 1984, “Positive Science and Discoverability”, PSA: Proceedings of the Biennial Meeting of the Philosophy of Science Association , 1984: 13–27.
- –––, 1985, “Beyond Divorce: Current Status of the Discovery Debate”, Philosophy of Science , 52: 177–206.
- –––, 1989, “Truth or Consequences? Generative versus Consequential Justification in Science”, PSA: Proceedings of the Biennial Meeting of the Philosophy of Science Association , 1988, 393–405.
- –––, 2018, “TTT: A Fast Heuristic to New Theories?” in Danks, D. & Ippoliti, E. (eds.) Building Theories: Heuristics and Hypotheses in Sciences , Cham: Springer, 213–244.
- Pasquale, J.-F. de and Poirier, P. 2016, “Convolution and Modal Representations in Thagard and Stewart’s Neural Theory of Creativity: A Critical Analysis ”, Synthese , 193: 1535–1560
- Paul, E. S. and Kaufman, S. B. (eds.), 2014a, The Philosophy of Creativity: New Essays , New York: Oxford Academic online edn., https://doi.org/10.1093/acprof:oso/9780199836963.001.0001.
- –––, 2014b, “Introducing: The Philosophy of Creativity”, in Paul, E. S. and Kaufman, S. B. (eds.), The Philosophy of Creativity: New Essays (New York: Oxford Academic online edn., https://doi.org/10.1093/acprof:oso/9780199836963.003.0001.
- Pietsch, W. 2015, “Aspects of Theory-Ladenness in Data-Intensive Science”, Philosophy of Science 82: 905–916.
- Popper, K., 2002 [1934/1959], The Logic of Scientific Discovery , London and New York: Routledge; original published in German in 1934; first English translation in 1959.
- Pöyhönen, S. 2017, “Value of Cognitive Diversity in Science”, Synthese , 194(11): 4519–4540. doi:10.1007/s11229–016-1147-4
- Pulte, H. 2019, “‘‘Tis Much Better to Do a Little with Certainty’: On the Reception of Newton’s Methodology”, in The Reception of Isaac Newton in Europe , Pulte, H, and Mandelbrote, S. (eds.), Continuum Publishing Corporation, 355–84.
- Reichenbach, H., 1938, Experience and Prediction. An Analysis of the Foundations and the Structure of Knowledge , Chicago: The University of Chicago Press.
- Richardson, A., 2006, “Freedom in a Scientific Society: Reading the Context of Reichenbach’s Contexts”, in J. Schickore and F. Steinle (eds.), Revisiting Discovery and Justification. Historical and Philosophical Perspectives on the Context Distinction , Dordrecht: Springer, 41–54.
- Russell, S. 2021, “Human-Compatible Artificial Intelligence”, in Human Like Machine Intelligence , Muggleton, S. and Charter, N. (eds.), Oxford: Oxford University Press, 4–23
- Schaffer, S., 1986, “Scientific Discoveries and the End of Natural Philosophy”, Social Studies of Science , 16: 387–420.
- –––, 1994, “Making Up Discovery”, in M.A. Boden (ed.), Dimensions of Creativity , Cambridge, MA: MIT Press, 13–51.
- Schaffner, K., 1993, Discovery and Explanation in Biology and Medicine , Chicago: University of Chicago Press.
- –––, 2008 “Theories, Models, and Equations in Biology: The Heuristic Search for Emergent Simplifications in Neurobiology”, Philosophy of Science , 75: 1008–21.
- Schickore, J. and F. Steinle, 2006, Revisiting Discovery and Justification. Historical and Philosophical Perspectives on the Context Distinction , Dordrecht: Springer.
- Schiller, F.C.S., 1917, “Scientific Discovery and Logical Proof”, in C.J. Singer (ed.), Studies in the History and Method of Science (Volume 1), Oxford: Clarendon, 235–89.
- Simon, H.A., 1973, “Does Scientific Discovery Have a Logic?”, Philosophy of Science , 40: 471–80.
- –––, 1977, Models of Discovery and Other Topics in the Methods of Science , Dordrecht: D. Reidel.
- Simon, H.A., P.W. Langley, and G.L. Bradshaw, 1981, “Scientific Discovery as Problem Solving”, Synthese , 47: 1–28.
- Smith, G.E., 2002, “The Methodology of the Principia ”, in G.E. Smith and I.B. Cohen (eds), The Cambridge Companion to Newton , Cambridge: Cambridge University Press, 138–73.
- Simonton, D. K., “Hierarchies of Creative Domains: Disciplinary Constraints on Blind Variation and Selective Retention”, in Paul, E. S. and Kaufman, S. B. (eds), The Philosophy of Creativity: New Essays , New York: Oxford Academic online edn. https://doi.org/10.1093/acprof:oso/9780199836963.003.0013
- Snyder, L.J., 1997, “Discoverers’ Induction”, Philosophy of Science , 64: 580–604.
- Solomon, M., 2009, “Standpoint and Creativity”, Hypatia : 226–37.
- Sternberg, R J. and T. I. Lubart, 1999, “The concept of creativity: Prospects and paradigms,” in R. J. Sternberg (ed.) Handbook of Creativity , Cambridge: Cambridge University Press, 3–15.
- Stokes, D., 2011, “Minimally Creative Thought”, Metaphilosophy , 42: 658–81.
- Tamaddoni-Nezhad, A., Bohan, D., Afroozi Milani, G., Raybould, A., Muggleton, S., 2021, “Human–Machine Scientific Discovery”, in Human Like Machine Intelligence , Muggleton, S. and Charter, N., (eds.), Oxford: Oxford University Press, 297–315
- Thagard, P., 1984, “Conceptual Combination and Scientific Discovery”, PSA: Proceedings of the Biennial Meeting of the Philosophy of Science Association , 1984(1): 3–12.
- –––, 1999, How Scientists Explain Disease , Princeton: Princeton University Press.
- –––, 2010, “How Brains Make Mental Models”, in L. Magnani, N.J. Nersessian and P. Thagard (eds.), Model-Based Reasoning in Science & Technology , Berlin and Heidelberg: Springer, 447–61.
- –––, 2012, The Cognitive Science of Science , Cambridge, MA: MIT Press.
- Thagard, P. and Stewart, T. C., 2011, “The AHA! Experience: Creativity Through Emergent Binding in Neural Networks”, Cognitive Science , 35: 1–33.
- Thoma, Johanna, 2015, “The Epistemic Division of Labor Revisited”, Philosophy of Science , 82: 454–472. doi:10.1086/681768
- Weber, M., 2005, Philosophy of Experimental Biology , Cambridge: Cambridge University Press.
- Whewell, W., 1996 [1840], The Philosophy of the Inductive Sciences (Volume II), London: Routledge/Thoemmes.
- Weisberg, M. and Muldoon, R., 2009, “Epistemic Landscapes and the Division of Cognitive Labor”, Philosophy of Science , 76: 225–252. doi:10.1086/644786
- Williams, K. et al. 2015, “Cheaper Faster Drug Development Validated by the Repositioning of Drugs against Neglected Tropical Diseases”, Journal of the Royal Society Interface 12: 20141289. http://dx.doi.org/10.1098/rsif.2014.1289.
- Zahar, E., 1983, “Logic of Discovery or Psychology of Invention?”, British Journal for the Philosophy of Science , 34: 243–61.
- Zednik, C. and Jäkel, F. 2016 “Bayesian Reverse-Engineering Considered as a Research Strategy for Cognitive Science”, Synthese , 193, 3951–3985.
How to cite this entry . Preview the PDF version of this entry at the Friends of the SEP Society . Look up topics and thinkers related to this entry at the Internet Philosophy Ontology Project (InPhO). Enhanced bibliography for this entry at PhilPapers , with links to its database.
[Please contact the author with suggestions.]
abduction | analogy and analogical reasoning | cognitive science | epistemology: social | knowledge: analysis of | Kuhn, Thomas | models in science | Newton, Isaac: Philosophiae Naturalis Principia Mathematica | Popper, Karl | rationality: historicist theories of | scientific method | scientific research and big data | Whewell, William
Copyright © 2022 by Jutta Schickore < jschicko @ indiana . edu >
- Accessibility
Support SEP
Mirror sites.
View this site from another server:
- Info about mirror sites
The Stanford Encyclopedia of Philosophy is copyright © 2023 by The Metaphysics Research Lab , Department of Philosophy, Stanford University
Library of Congress Catalog Data: ISSN 1095-5054
Featured Topics
Featured series.
A series of random questions answered by Harvard experts.
Explore the Gazette
Read the latest.
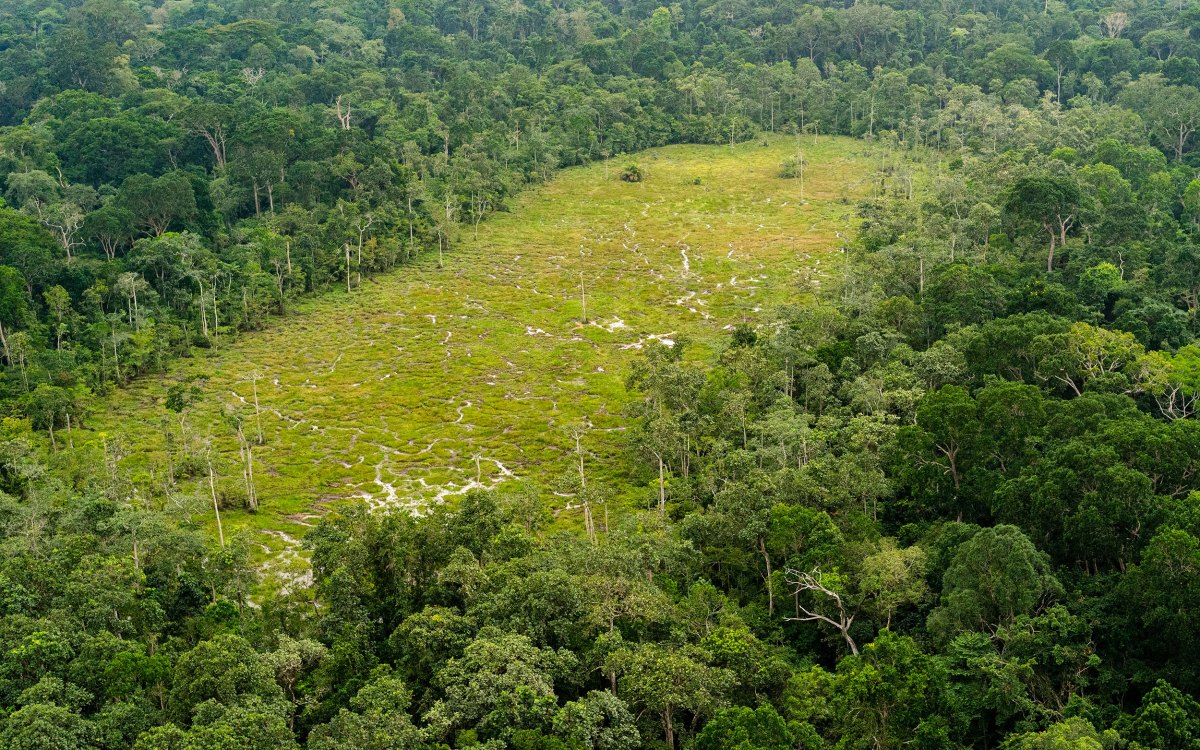
Grappling with how clearings may support rainforest animal life
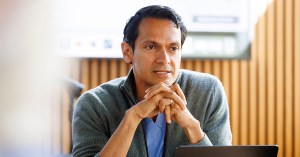
Amid Hurricane Milton’s devastation, a sliver of good news
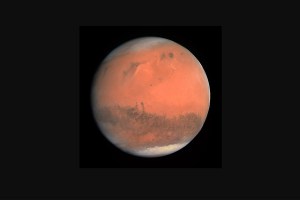
Mars may have been habitable much more recently than thought
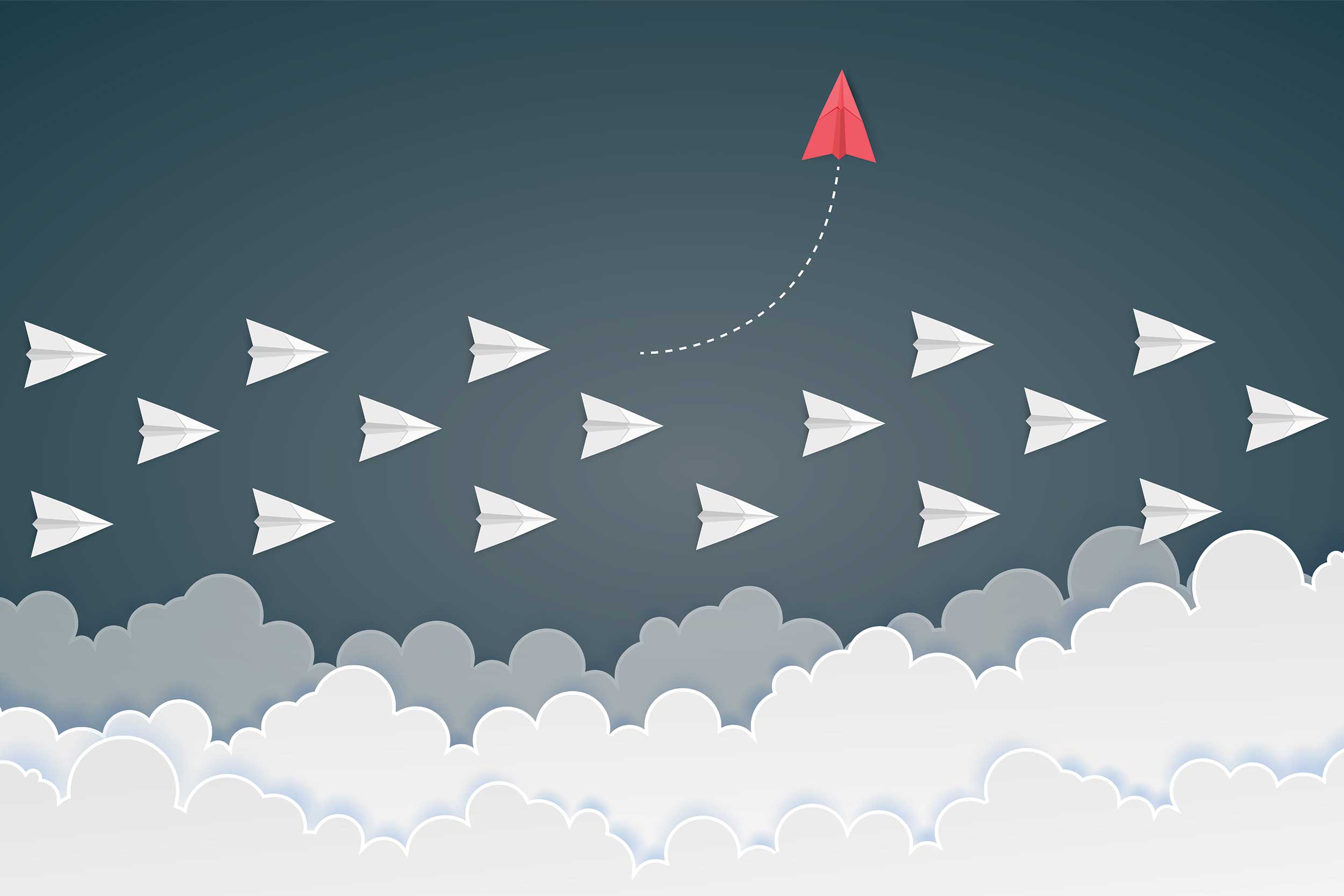
iStock by Getty Images
What it takes to be a scientific breakthrough
Anthony L. Komaroff
Harvard Health Blog Contributor
Medical School professor explains scientific advances vs. world-changing discoveries
In science, advances are a daily occurrence, but true breakthroughs are rare. What does it take to achieve world-changing scientific breakthroughs? Some are the result of a lucky accident, combined with curiosity: scientists traveling down one road suddenly find reason to veer onto another road, one they never planned to travel — a road that may well lead nowhere.
Other major breakthroughs stem from scientists pursuing a very specific dream. One day, usually early in their career, they get an idea that they can’t stop thinking about. It’s crazy, they say to themselves, but is it really impossible? They talk to respected colleagues who often remind them of all the reasons their idea might not work, and how damaging this could be for their career. It’s a sobering message, yet the idea won’t die. So, they scramble to find financial support and seek out colleagues willing to risk traveling that road with them — a road that may well lead nowhere. But sometimes the road leads to major breakthroughs like penicillin and mRNA vaccines.
Breakthroughs due to lucky accidents and curiosity
One day in 1928, Dr. Alexander Fleming at St. Mary’s Hospital in London was growing bacteria in a laboratory dish. Fleming was not pursuing a scientific dream. He was a microbiologist, just doing his job.
Then he noticed something odd: overnight, another kind of microbe, a fungus, had traveled through the air, landed on the laboratory dish, and started to grow and spread on the dish where the bacteria were growing. Fleming soon noticed that the growing fungus seemed to be killing the bacteria. He surmised that it was making some substance that killed the bacteria. Because the name of the fungus was Penicillium rubens, he called the substance the fungus was making “penicillin.”
When Fleming published a paper about his discovery, few were interested. It took another 10 years before other scientists tried to generate large amounts of penicillin to see if it might be able to cure bacterial infections and save lives. We all know how that worked out.
Fleming’s scientific breakthrough, like some others, occurred not because Fleming had a brilliant idea and exclaimed “Eureka!” Instead, it occurred because he noticed something and said, “That’s odd,” and then tried to figure it out.
Breakthroughs due to persistence and resilience in pursuit of a dream
The story of mRNA vaccines, like the Pfizer/BioNTech and Moderna vaccines for COVID-19, is very different from the story of penicillin. For 30 years, a small group of scientists believed that mRNA vaccines would have great advantages over traditional vaccines — if only several obstacles could be overcome. Many of these scientists gave up as they encountered those obstacles, but a few persisted and, ultimately, succeeded. (I described what mRNA vaccines are, how they work, and how obstacles were overcome in a previous blog post .)
One scientist, Dr. Katalin Karikó, joined the faculty of the University of Pennsylvania in the early 1990s with the dream of creating an mRNA vaccine. She applied for grants to support her work, but was repeatedly rejected: the reviewers stated that it was so unlikely that she or anyone could overcome the obstacles that supporting her research would be a wasted investment. Her university only agreed to continue supporting her work if she accepted a demotion and a pay cut. She accepted both, and doggedly pursued her dream.
One major obstacle to mRNA vaccines particularly fascinated her: the violent reaction of the immune system when it encounters mRNA from a virus. Ten years of dogged work helped Karikó and her colleague Drew Weissman figure out how to make a small change in mRNA that prevented that violent immune response — a major step in making all mRNA vaccines possible. Without this, the world wouldn’t have mRNA COVID vaccines today.
Two other scientists who created the Pfizer/BioNTech COVID vaccine, Uğur Şahin and Őzlem Turëci, are Turkish immigrants to Germany who met, fell in love with the idea of creating an mRNA vaccine, and then fell in love with each other. According to The Wall Street Journal, one day in 2002 they took a break for lunch, got married, and then returned in the afternoon to their laboratory to finish an experiment — just one more among many conducted over 30 years. Each experiment was one more possible step toward their ultimate dream until finally, in 2020, they achieved that dream: their mRNA vaccine for COVID-19 proved to be very safe and effective.
Holding hard to their dreams
Whichever path scientists who achieve lifesaving breakthroughs travel, they often endure disinterest, like Fleming, or repeated skepticism, ridicule, and rejection, like Karikó, Weissman, Şahin, and Turëci. Only through sheer persistence did these scientists bring their dreams to life. They have been rewarded with fame and wealth and something even more valuable: the knowledge that because of their work hundreds of millions of people around the world never got sick, and millions never died before their time.
Of course, a relentless obsession with an improbable dream fails to pay off for many scientists. Their ideas, while quite brilliant, in the end are proved wrong: nature doesn’t turn out to operate the way they predicted. In the end, their beautiful theory is murdered by a brutal gang of facts.
Still other scientific dreamers ultimately prove to have been on the right track all along and would have achieved their dream — if only they had done the experiment a little differently, if only they had persisted a little longer, or if only the support for their work had not run out. As a result, neither they nor the rest of us benefited from what would have been — until other scientists rediscovered their work years later.
Ultimately, scientific breakthroughs are possible only if a society is willing to invest in dreamers, recognizing that not all investments will lead to major breakthroughs. However, the investments that do lead to breakthroughs bring an economic return that is far greater than the investment — as well as preventing suffering and death and changing the world.
This story originally appeared in the Harvard Health Blog .
Anthony L. Komaroff is the Steven P. Simcox/Patrick A. Clifford/James H. Higby Professor of Medicine at Harvard Medical School, senior physician at Brigham and Women’s Hospital in Boston, and editor-in-chief of the Harvard Health Letter.
Share this article
You might like.
New research offers detailed overview of layout, makeup of canopy gaps in Congo

Cellphone data suggest evacuation mandates, warning systems worked
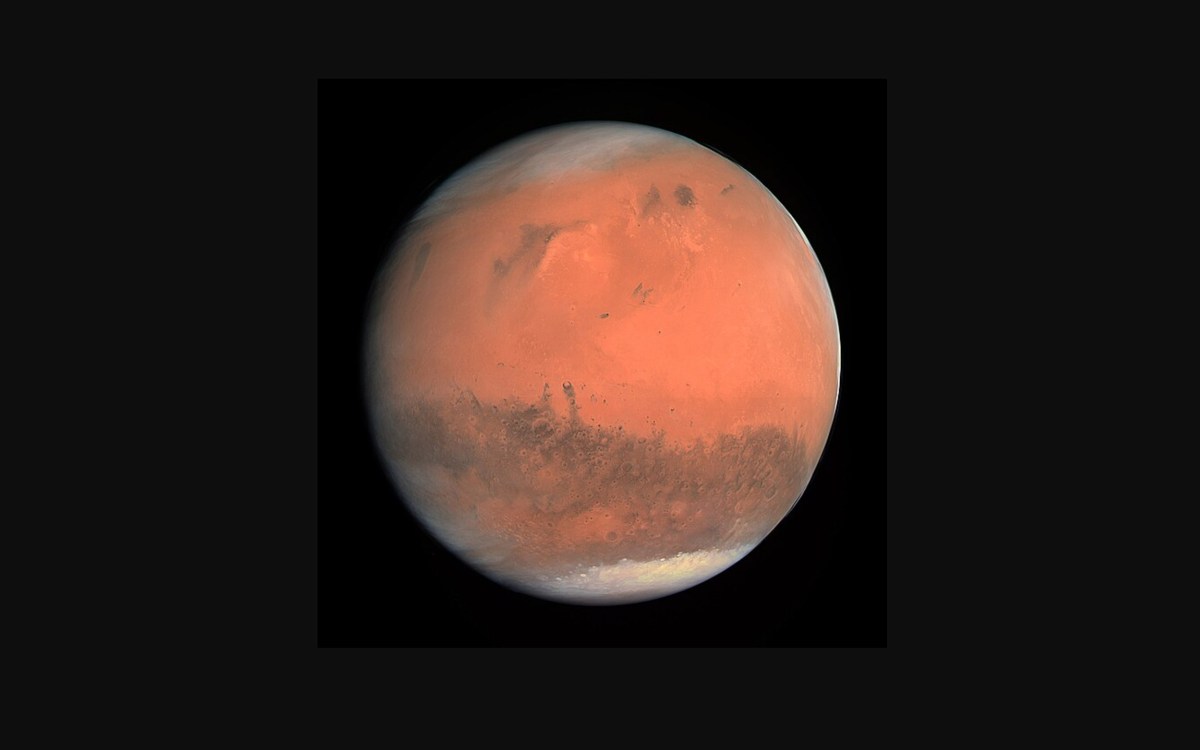
Study bolsters theory that protective magnetic field supporting life-enabling atmosphere remained in place longer than estimates
How to fight depression? Faster.
Hope flags when medications fail, isolating and endangering patients. Backed by a major grant, 2 Harvard scientists are focused on reducing the distance between diagnosis and recovery.
Plastics are everywhere, even in our bodies
We ingest equivalent of credit card per week — how worried should we be? In ‘Harvard Thinking,’ experts discuss how to minimize exposure, possible solutions.
Your side might lose. But you don’t have to lose your mind.
Political engagement is healthy. Doomscrolling? Not so much.
Crucial Scientific Discoveries in Twenty-First Century Essay
Introduction, automobile industry, communication sector, education and schools, works cited.
Scientists are making various inventions especially in the field of medicine and information technology. The need for people to live comfortable and easier lives has led to various inventions which are currently being adapted. America has become the center for such inventions as people adapt more sophisticated lifestyles. Even though the changes seem to impact positively on peoples lives, research has revealed that they have an impact on the health of individuals.
One thing about such inventions is that they never stand the test of time. Most of them excite their users for a particular period of time and there after they are replaced with new ones. Examples of such are the new inventions that are being done on mobile phones. Mobile phone companies are venturing into designs that will suit the needs of modern people. A mobile phone is currently being used to perform more function apart from the original design of calls and text messages.
Man is being transformed from being a social being to a person who mainly operates through machines. In the current world, it is more about how one knows how to operate machines than how they can relate with people. There is little interaction of people as everybody seeks to do their transactions digitally (Macionis 489). These improvements are already having negative impacts on people and there is still much that is expected.
The greatest need in the current society is health care; there is a need for more advanced health care facilities to cater for new complications that are related to changes in lifestyles. The use of automobiles travel even for shorter distances has led to obesity and heart complications. Complications that were previously known to affect the older generations are now being reported in the younger. Technology has also made people to adapt unhealthy eating habits. Fast foods are gaining popularity as people lack the time to make and eat healthy meals. Foods that are considered to be healthy have become tasteless as people look out for sweetness. Below are some of the major scientific innovations and their predicted effects in the 21 st century.
The automobile industry has led to the invention of modern cars that are meant to make transport easier. Compared to previous inventions that caused a lot of pollution on the environment, there has been a need for environmental friendly vehicles. There is also an extinction on fuel resources that has made fuel prices to be exorbitant. Technology is therefore looking for a means of adapting alternative fueling mechanisms that will be economical and also risk free.
Electricity has been considered to be another alternative except that it is very expensive. There are also other alternatives that are already being used only that they require a lot of money to install and maintain. It is hence expected than the living standards will be on the high as people will have no alternative but to adapt to the changes. There are certain engine vehicles that have already been banned in the market and it is expected that such bans will continue.
This is due to the need of conserving the environment which has a direct impact on people’s health. In the near future, there are certain engine cars that will be prohibited in the market and on roads. This will lead to increased expenses as people will be required to change what they are currently driving to something else that is considered better.
With the changes that are taking place in the field of information technology, we are looking forward to more sophisticated means of communication. People have also become too busy to even handle a short conversation. The use of voice mails enables people to listen to calls at their convenient time; it makes it possible for people not to miss out on their calls as they can easily leave a recorded message. The improvements that are being made will ensure that people who are talking over the phone see each other. The ability of a phone to record live pictures that will show where a person is and what they are currently doing are being facilitated.
They will have strong signals that will be able to tell the exact location of an individual. It will hence be easier to trap the location of the caller by simply reading through the signals (Macionis 503). The concept of people using mobile phones to cheat on their callers will be eliminated. There will however be call settings that will also enable a person to hide the identity of their location and hence making them even trickier. We are also looking forward to seeing more transactions being done via the mobile phones. It will even be possible to acquire important documents on line such as marriage certificates.
Internet technology has given rise to online learning where by people are acquiring degrees by studying via the internet. There is a lot of advertisement on how one can acquire a higher degree from a university that is overseas. It is hence predicted that in future, all learning will be done online. There will be an increase in learning institutions that are based online for both senior and junior students. There are some characters that students acquire while in schools which is causing a threat to their morals. Parents who care much about the moral standing of their children will hence opt for online schools where they can study under the supervision of their parents (Macionis 490).
As much as they will be doing it for the sake of protecting their children, it will be done at the expense of their social life which is necessary for their growth. It will also mean that outdoor games will be replaced by indoor and computer games. This will be a serious social issue which will be transferred to the future generation. It will basically mean that people will be so close yet know little about each other. There will be no personal relationship between the teachers and their students. They can only communicate online and acquire the knowledge that they need but will not be able to meet and talk face to face.
The issue of science making life easier or more complicated has been a debate whose clear answer has not been identified. Everything is based on a fifty percent analysis which has made people to live with it. Despite the desirable and undesirable consequences that scientific inventions are having on individuals, change is inevitable. People have had to admit that life is becoming more sophisticated with the improvements that are being made in the field. It is however impossible to do without some of the inventions that are being made as they form part and parcel of our lifestyles. Even the most cultured individuals have found it difficult to resist the changes as they are swept by them. The only thing that has remained for us is to accept the changes and bear the consequences.
Macionis, John. Society: The Basics . New York: Prentice Hall, 2008.
- “Shrinking the World” by John Freeman
- Technology and Type of Powers on the Workplace
- "Foundations for the Twenty-First Century" by Michael J. Anthony Review
- “Twenty-First Century Socialism”
- School Leadership in the Twenty-First Century
- Technology and Lifestyle in the United States
- Mobile Texting and Chatting Negative Effects
- Science and Technology Will Change Our Future
- Advantages of Using Computers at Work
- “Why the Future Doesn’t Need Us” by Bill Joy
- Chicago (A-D)
- Chicago (N-B)
IvyPanda. (2021, December 19). Crucial Scientific Discoveries in Twenty-First Century. https://ivypanda.com/essays/crucial-scientific-discoveries-in-twenty-first-century/
"Crucial Scientific Discoveries in Twenty-First Century." IvyPanda , 19 Dec. 2021, ivypanda.com/essays/crucial-scientific-discoveries-in-twenty-first-century/.
IvyPanda . (2021) 'Crucial Scientific Discoveries in Twenty-First Century'. 19 December.
IvyPanda . 2021. "Crucial Scientific Discoveries in Twenty-First Century." December 19, 2021. https://ivypanda.com/essays/crucial-scientific-discoveries-in-twenty-first-century/.
1. IvyPanda . "Crucial Scientific Discoveries in Twenty-First Century." December 19, 2021. https://ivypanda.com/essays/crucial-scientific-discoveries-in-twenty-first-century/.
Bibliography
IvyPanda . "Crucial Scientific Discoveries in Twenty-First Century." December 19, 2021. https://ivypanda.com/essays/crucial-scientific-discoveries-in-twenty-first-century/.
- To find inspiration for your paper and overcome writer’s block
- As a source of information (ensure proper referencing)
- As a template for you assignment
IvyPanda uses cookies and similar technologies to enhance your experience, enabling functionalities such as:
- Basic site functions
- Ensuring secure, safe transactions
- Secure account login
- Remembering account, browser, and regional preferences
- Remembering privacy and security settings
- Analyzing site traffic and usage
- Personalized search, content, and recommendations
- Displaying relevant, targeted ads on and off IvyPanda
Please refer to IvyPanda's Cookies Policy and Privacy Policy for detailed information.
Certain technologies we use are essential for critical functions such as security and site integrity, account authentication, security and privacy preferences, internal site usage and maintenance data, and ensuring the site operates correctly for browsing and transactions.
Cookies and similar technologies are used to enhance your experience by:
- Remembering general and regional preferences
- Personalizing content, search, recommendations, and offers
Some functions, such as personalized recommendations, account preferences, or localization, may not work correctly without these technologies. For more details, please refer to IvyPanda's Cookies Policy .
To enable personalized advertising (such as interest-based ads), we may share your data with our marketing and advertising partners using cookies and other technologies. These partners may have their own information collected about you. Turning off the personalized advertising setting won't stop you from seeing IvyPanda ads, but it may make the ads you see less relevant or more repetitive.
Personalized advertising may be considered a "sale" or "sharing" of the information under California and other state privacy laws, and you may have the right to opt out. Turning off personalized advertising allows you to exercise your right to opt out. Learn more in IvyPanda's Cookies Policy and Privacy Policy .
Home — Essay Samples — Science — Isaac Newton — Isaac Newton: The Life and Legacy of a Scientific Pioneer

Isaac Newton: The Life and Legacy of a Scientific Pioneer
- Categories: Isaac Newton
About this sample

Words: 514 |
Published: Jan 30, 2024
Words: 514 | Page: 1 | 3 min read
Table of contents
Introduction, early life and education of isaac newton, newton's contributions to mathematics, newton's achievements in science, newton as an inventor and innovator, newton's legacy and impact on the scientific world.
- Encyclopedia Britannica. (n.d.). Isaac Newton. Encyclopedia Britannica. https://www.britannica.com/biography/Isaac-Newton
- Newton, (1729). Mathematical principles of natural philosophy. London.
- University of Cambridge. (n.d.). Sir Isaac Newton. University of Cambridge. https://www.cam.ac.uk/research/news/sir-isaac-newton
- Westfall, R. S. (1995). The life of Isaac Newton. Cambridge University Press.
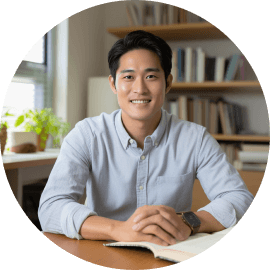
Cite this Essay
To export a reference to this article please select a referencing style below:
Let us write you an essay from scratch
- 450+ experts on 30 subjects ready to help
- Custom essay delivered in as few as 3 hours
Get high-quality help
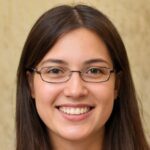
Verified writer
- Expert in: Science
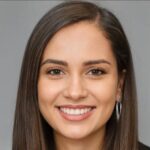
+ 120 experts online
By clicking “Check Writers’ Offers”, you agree to our terms of service and privacy policy . We’ll occasionally send you promo and account related email
No need to pay just yet!
Related Essays
4 pages / 1856 words
2 pages / 1114 words
2 pages / 710 words
1 pages / 441 words
Remember! This is just a sample.
You can get your custom paper by one of our expert writers.
121 writers online
Still can’t find what you need?
Browse our vast selection of original essay samples, each expertly formatted and styled
Related Essays on Isaac Newton
Isaac Newton, a renowned mathematician and physicist, formulated three laws of motion that laid the foundation for classical mechanics. These laws, known as Newton’s Laws of Motion, have provided a framework for understanding [...]
In the vast realm of human knowledge, there are few figures as influential as Sir Isaac Newton. His groundbreaking discoveries in the fields of physics and mathematics revolutionized our understanding of the natural world, but [...]
Sports are most easy and convenient ways for the very good physical and mental exercise. Sports bring a lot of benefits to everyone. Sports provide a person the feeling of well-being and encourage living a healthy life. It keeps [...]
This lab report delves into the fundamental principles of Newton's Second Law of Motion, exploring the interplay between mass, acceleration, and force. The primary objective of this experiment is to ascertain how Newton's second [...]
Sir Isaac Newton, born on January 4, 1643, in Woolsthorpe, England, stands as one of the most illustrious figures in scientific history. His profound contributions to various fields, including the development of calculus, the [...]
Most of the time a student’s achievement in education is measured by grades. Can you achieve more success in life with higher grades? Grades portray what the teacher or professors believe to be the best or worst answer. A [...]
Related Topics
By clicking “Send”, you agree to our Terms of service and Privacy statement . We will occasionally send you account related emails.
Where do you want us to send this sample?
By clicking “Continue”, you agree to our terms of service and privacy policy.
Be careful. This essay is not unique
This essay was donated by a student and is likely to have been used and submitted before
Download this Sample
Free samples may contain mistakes and not unique parts
Sorry, we could not paraphrase this essay. Our professional writers can rewrite it and get you a unique paper.
Please check your inbox.
We can write you a custom essay that will follow your exact instructions and meet the deadlines. Let's fix your grades together!
Get Your Personalized Essay in 3 Hours or Less!
We use cookies to personalyze your web-site experience. By continuing we’ll assume you board with our cookie policy .
- Instructions Followed To The Letter
- Deadlines Met At Every Stage
- Unique And Plagiarism Free

COMMENTS
Essay on Scientific Discoveries: Importance of discoveries and inventions. How to write short essays and speeches. Important topics & ideas.
DNA, gravity, and germ theory are a few of the key findings in history that forever shifted the course of human civilization. Learn how these scientific discoveries changed the world.
The Top Ten Scientific Discoveries of the Decade. Breakthroughs include measuring the true nature of the universe, finding new species of human ancestors, and unlocking new ways to...
Scientific discovery is the process or product of successful scientific inquiry. Objects of discovery can be things, events, processes, causes, and properties as well as theories and hypotheses and their features (their explanatory power, for example).
In an essay for the BBC, Nobel Prize-winner and Royal Society President Sir Venki Ramakrishnan contemplates the nature of scientific discovery - how it has transformed our worldview in a...
Medical School professor explains scientific advances vs. world-changing discoveries. In science, advances are a daily occurrence, but true breakthroughs are rare. What does it take to achieve world-changing scientific breakthroughs?
Impact of Scientific Discoveries on the World Essay. Exclusively available on IvyPanda®. William and Colin (2009) define scientific discoveries as the incoming achievements that are majorly grasped through a thorough exploitation and research on nature and the societal needs. Get a custom essay on Impact of Scientific Discoveries on the World.
This guide is designed to provide help and advice on scientific writing. Although students studying Medical and Life Sciences are most likely to have to write essays for tutorials at Oxford, it is important all scientists learn to write clearly and concisely to present their data and conclusions.
Crucial Scientific Discoveries in Twenty-First Century Essay. Exclusively available on Available only on IvyPanda® Made by Human • No AI. Table of Contents. Introduction. Scientists are making various inventions especially in the field of medicine and information technology.
Born in 1643 in England, Newton's contributions to mathematics, physics, and astronomy revolutionized our understanding of the universe. Through his inventions, discoveries, and innovations, Newton laid the foundation for modern scientific inquiry and transformed the world as we know it.