- Activities for Kids
- Family Life

Under Pressure! 10 At-Home Science Experiments That Harness Air
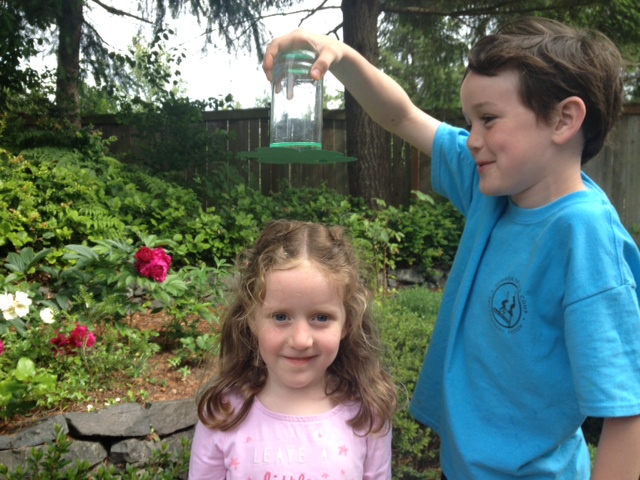
If the at-home orders have you scrambling for indoor activities , we’ve got easy science experiments you can pull out at a moment’s notice from the comfort of your home. Each kids science experiment reveals air’s invisible power, and (usually) uses what you’ve got in the recycling bin to demonstrate it. Read on to learn how to levitate water, submerge tissues without getting them wet and suck an egg into a jug using only a match.
Keep it Simple
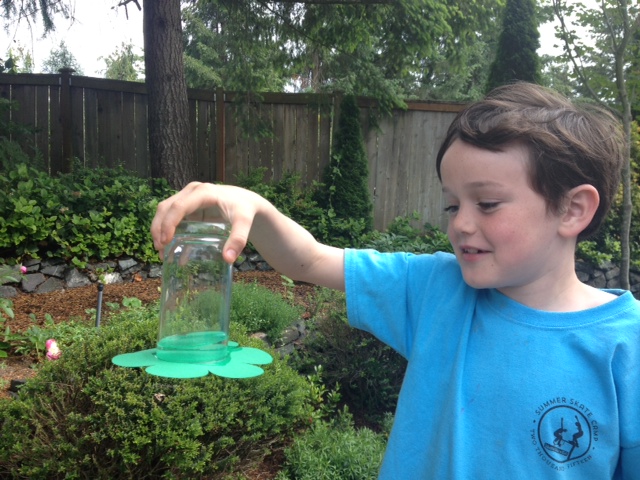
Thankfully, science experiments don't have to be super complex or time consuming. These easy-peasy experiments only require a little prep and leave a big impression on tiny minds. Plus, we’re betting most of what you need to test these theories is already lying around your house.
1. Sink or Swim. Instead of bobbing for apples, your tiny tot will make straws dive and surface with a gentle squeeze. The Kids Activities Blog lays out the important deets for this hands-on experiment that uses a two-liter bottle and play dough to fully certify straws as scuba-ready. Take the dive into serious science with this one!
Why it works: Squeezing the bottle increases the air pressure inside the bottle and forces water up into the straw, which makes it heavy enough to sink.
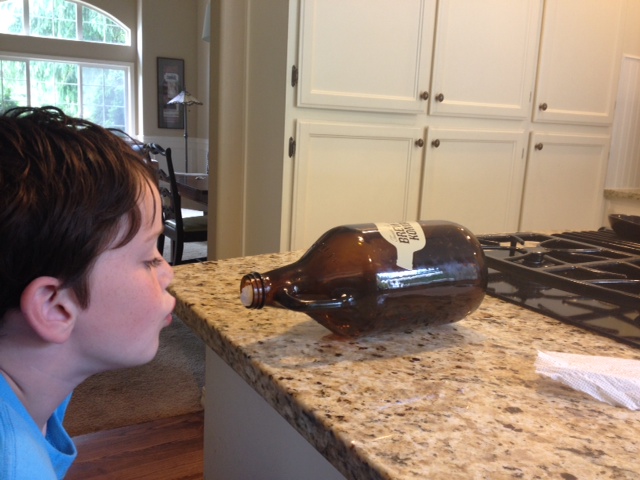
2. Blow Their Minds . Bet your cutie a clean room that she can’t blow a rolled up piece of paper towel into an empty bottle. Sounds like a safe bet, right? But thanks to air pressure, the cards are definitely stacked in your favor. To set up the experiment, place an empty two-liter bottle on its side. Ball up the corner of a paper towel that’s about half the size of the bottle’s top and place it just inside the opening then challenge your little scientist to blow the paper towel into the bottle (Trust us, it can’t be done). No matter how hard she tries, she’s not going to win that bet. Learning plus a clean room? We’ll take it!
Why it works: Even though you can’t see it, that bottle is full of air; when you try to blow something into it, there’s just no room.
3. Be Unpredictable. Two balloons, a yardstick, string, and a hairdryer are all you need for this experiment that will keep your mini me guessing. To get things moving in the right direction, blow up the balloons to the same size and then use the string to attach them, a few inches apart, to the yardstick. Once you’re all set up, ask your kidlet what will happen to the balloons when you aim air from the hair dryer between the two balloons. The obvious answer? They’ll be blown apart. But once your wee one takes aim, she’ll see that the balloons are actually pushed together rather than apart. Who knew?
Why it works: Blowing air between the balloons lowers the air pressure and makes the pressure surrounding them higher, pushing them together.
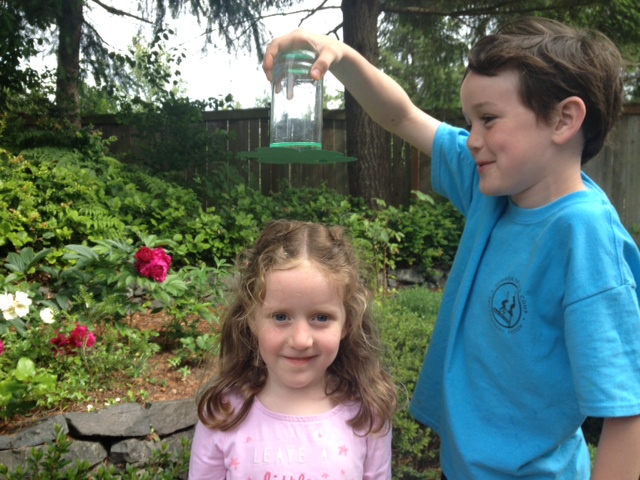
4. Levitate Water . You won’t need to incant Wingardium Leviosa with perfect pronunciation to suspend water during this exciting experiment. Start by filling a glass of water about 1/3 full, then cover it with a piece of cardstock. Tip the glass over, keeping the cardstock in place with your hand, and hold the whole shebang over your unsuspecting kidlet’s head (or a sink if you want to do a test run first!). Then slowly let go of the cardstock while your mini me waits excitedly below. Look ma, no splash! The card stays in place and your little guinea pig stays dry.
Why it works : The outside air pressure working against the cardstock is greater than the weight of the water in the glass.
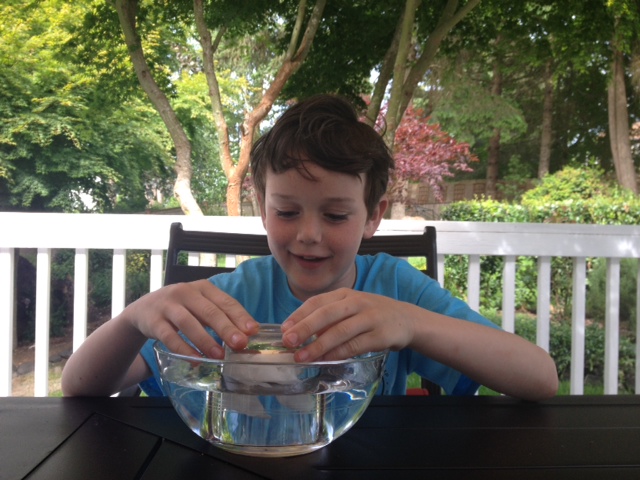
5. Grab a Tissue. To be wet or not to be wet is the question answered in this simple experiment full of drama. To set the scene, loosely crumple a tissue so that when you stick it in a small glass and turn it over the tissue doesn’t fall out. Then, have your little lab assistant fill a bowl with water, turn the glass over and submerge it completely (psst… keep the glass parallel to the water to make the experiment work). Ta da! The tissue stays dry even when it’s below the water line.
Why it works: The air pressure inside the glass is strong enough to keep the water out and the tissue dry.
Complicate Matters
Get mom or dad in on the action with these experiments that take a little more time and some helping hands to demonstrate just how powerful air pressure can be.
6. Blast Off. Nothing makes air pressure more tangible than a classic bottle rocket launched on a sunny summer afternoon. You and your sidekick can spend time fashioning a plastic bottle into a space-worthy vessel with a cone top and flamboyant fins on the side. Then, hook it up to the air pump and let her rip! Up, up and away! Science Sparks has simple instructions you can use (and even a cool video!) to make one with your budding scientist.
Why it works: Pumping air into the bottle builds up pressure until you just can’t add any more and all that force sends the rocket flying.
7. Make Eggs Magical. This “look ma, no hands, wires or mirrors” trick will get them every time; an egg being sucked into a jar while your little scientist delightedly looks on is always a hit. To perform this illusory feat, you’ll need a glass jar with an opening just smaller than an egg (think: old school milk jug) and a peeled, boiled egg. When you and your Little have checked these items off your list, it’s time to start the show. Mom or dad should toss a lit match into the glass jar, followed by your mini lab assistant, who’ll quickly set the egg over the opening. Abracadabra! Alakazam! The match dies out; the egg gets (seemingly) inexplicably sucked into the bottle. And just like that you’ve performed another bit of parent magic without breaking a sweat.
Why it works: The match uses up the air inside the bottle. Once that happens the pressure outside the bottle is greater and pushes the egg down into the bottle.
8. Build a Barometer. The invisible air pressure around us is always changing, but try explaining that to the tot lot. We've found a seeing-is-believing DIY barometer experiment to turn the tides for your tiny skeptic. Not only will you reveal ever-changing air pressure, but you can also predict any summer storms heading your way. Get all you need to know about making your own version using a screw-top jar, rubber bands and a straw at Wonderful Engineering .
Why it works: When the air pressure is high, it pushes down on the straw tilting it up, and when it’s low, pressure inside the jar pushes up against the straw pointing it down.
9. Inflate Marshmallows. Put those marshmallows you’re stockpiling for summer s’mores to good use in this DIY vacuum experiment. To make the vacuum, use a hammer and nail to pierce a hole (big enough to fit a straw) into the lid of a screw-top glass jar. Next, stick a straw ever-so-slightly into the hole and seal the edges with play dough or molding clay so there’s no way for the air to get out other than through that straw. Now you’re ready to see what happens to a marshmallow when it’s trapped inside; place the marshmallow in the jar, screw the top back on, and have your mini me take the air out gulp by gulp through the straw (just be sure to cover the straw hole between breaths so no air makes it back in). As the air is removed, the marshmallow expands, like a nightmare vision straight out of Ghostbusters . Who you gonna call?
Why it works : When you use a straw to remove all the air from the jar, there’s no air left working against the marshmallow. Instead, the air trapped inside the marshmallow is able to expand.
10. Pit Balloons Against Bottles. Is your future scientist ready for another challenge? Just like blowing a paper towel into a jug, this science experiment from Steve Spangler Science is oh-so-much harder than it looks. To entice your little experimenter, place an un-inflated balloon into an empty plastic bottle and ask him if he thinks he can blow it up. Easy right? But no matter how hard he tries, that balloon just won’t fill with air! The trick to inflating the balloon is a simple one that takes mom or dad’s helping hand and just like that, what was once impossible becomes possible!
Why it works: At first, the bottle is full of air so there’s no room for the balloon to expand when you try to blow it up. But when you try this experiment after the trick, there’s an escape route for the air inside the bottle, leaving room for the balloon to inflate.
Need some fresh ideas?
Subscribe to our weekly newsletter for expert parenting tips and simple solutions that make life instantly better.
By subscribing you agree to Tinybeans Terms and Privacy Policy
Related reads
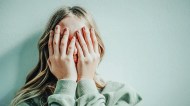
Why Are Gen Z Kids Covering Their Noses in Family Photos?
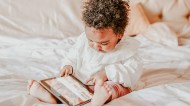
Screen Time for Babies Linked to Sensory Differences in Toddlerhood, Study Shows
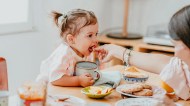
Kids Shouldn’t Have to Finish Dinner to Get Dessert, Dietitian Explains
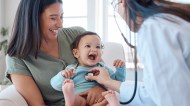
The Questions Parents Should Be Asking Their Pediatrician—but Aren’t
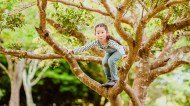
6 Better Phrases to Say Instead of ‘Be Careful’ When Kids Are Taking Risks
- your daily dose
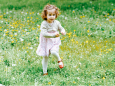
- and connection
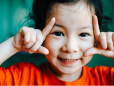
- Your daily dose
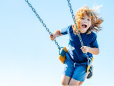

- Nov 18, 2019
10 Simple Experiments for Density and Buoyancy and Air Pressure
Updated: Jun 24, 2020

Develop an understanding of air pressure, buoyancy, and density using a series of hands-on labs.
When I’m teaching a science concepts like air pressure and density my goal is to help kids build mental models of what’s going on. Whenever possible I try to start with something they can touch and feel and experience. Here’s a simple sequence we did in my classroom. I hope you can see how students’ understanding builds.
1. Air is Stuff: Air Pressure Experiment with Water
This activity is a good place to start. When you try to pour water into the jug, it won’t go in. This is a concrete way to show that air is stuff. This always surprises and puzzles kids and encourages them to play. And when they’re intrigued, kids engage with difficult material more easily.

This is where we begin our study of buoyancy. Can you see where this will lead?
If you don’t get the idea that air is stuff, you won’t believe that it has weight. And if you don’t believe that air has weight, you won’t see how it can produce pressure. And if you don’t understand how air produces pressure, you won’t be able to see how it creates buoyancy. And if you don’t understand how buoyancy works, then it’s tough to grasp the concept of density. Sure, you can memorize the formula for density, but what does that tell you about density? BTW what IS the formula for density? And will it be on the test?
2. Matter Presses: Understanding Pressure
Once we proved to ourselves that air is stuff, we’ll play with the concepts of weight and pressure. This activity is free on my website. If you’re interested in a copy, you can sign up here.

This is a super simple activity to show kids how the weight of an object (our body) doesn’t change as you change your position (squatting, sitting, standing on tiptoe), yet its pressure does. It’s a concrete way for kids to feel the connection between the concepts of weight and pressure.
We’re just getting started on our investigation into density, buoyancy, and air pressure. These three concepts are related, and it’s helpful to study them together. In this activity, kids see how pressure comes from weight. We’ll continue that line of thought in the next couple of activities.
3. Streamlines: Water Pressure Experiments with a Water Bottle
Have you tried this experiment? It’s easy, a little messy, and super fun. Plus, kids find it intriguing, so that’s a huge point in its favor.
How many observations can you make? Note how the lower streams are shooting farther than the upper ones. What could you conclude from that?
Click here (or on a pic) for middle school labs on this topic.
This is a visual example showing how pressure comes from weight. The greater push comes from the taller column of water. Kids can prove this to themselves by comparing bottles of different diameters and heights. It’s easy to conclude that it’s only the height of the water that changes the shape of the squirt.
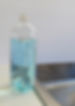
This activity gives good evidence that the water sitting above the hole produces the pressure. This is a direct correlation to air pressure, which comes from the weight of Earth’s air sitting on top of you.
The difficulty with understanding air pressure is that we ignore the surrounding air. We rarely think of air as sitting on us. It’s invisible so we forget it’s there. Time to roll the tape from activity #1 . Air is stuff. It’s always there and we need to remember this to understand air pressure.
If you climb a mountain to a place where there’s less air above you, there’s less pressure. And vice versa, the lower you go, the higher the pressure. We call sea-level standard pressure, but if you go below sea level (into a cave for instance) air pressure increases.
[Students may know that air high in the atmosphere is thinner than that near sea level. While that’s important, it’s a separate issue and we don’t deal with it yet.]
This is part 3 of our conceptual journey—we’ve determined that air is stuff and we’ve connected weight to pressure. The definition of stuff is that it has weight and takes up space. And if air has weight, it must be able to produce pressure by sitting on stuff.
And what keeps air sitting on Earth? The same force that keeps every other substance sitting on Earth… gravity! Just because it’s light and thin and invisible doesn’t make it immune to gravity. Gravity gives air its weight and air’s weight produces pressure. It’s that simple. The complicated part is that we haven’t trained our brains to think in those terms. We forget that air is there and we forget that air is stuff. So it’s helpful to refer to experiments that kids have completed—like trying to pour water into a sealed bottle (experiment #1 ). The water won’t go in because the bottle is already full… of air.
And this is our job as teachers—to help kids think like scientists.
4. Nature Abhors a Vacuum: Playing with Suction Cups
Now that we’re beginning to get an idea of where air pressure comes from, what if we could change it? What if we could change the pressure around an object? How would that affect it? In this activity, we play with suction cups. Their shape allows them to trap some air and then change their volume.

If their volume increases but the amount of air inside stays the same, the pressure will drop. Now the inside pressure is less than the outside pressure. It’s this small difference that makes suction cups stick. The higher outside air pressure is pushing them against the surface, keeping them attached.
This is a good activity to delve into the idea that pressure can come from two different sources. We’ve already looked at what causes the outside, or atmospheric, pressure (air’s weight).
And now we’re looking at the pressure which comes from the air pushing against the sides of the container. All gasses exhibit this pushiness. This is a more common understanding of air pressure and one that confuses kids when they’re learning about atmospheric pressure.
5. Nature Abhors a Vacuum: Playing in the Tub
Who hasn’t tried this? Umm, a lot of kids apparently. Part of our job as science teachers is to help kids play with materials so they can discover concepts on their own. Play builds a library of phenomena and experiences that kids can refer to when unpacking their understandings. Here they see how they can lift a full, upside-down cup and it doesn’t empty. It remains full until the rim of the cup breaks the surface of the water. They can use a bottle of any shape or size and see the same results.
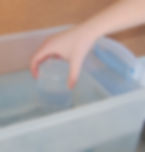
What keeps the water in the cup?
Water seeks its level by falling to the lowest point. But for water to leave this cup, a vacuum would have to form in the space since there’s no way for air to enter. The surrounding air pressure pushes on the surface of the water and holds the water in the cup.
What if the cup were very tall, wouldn’t the pressure from the water in the cup overwhelm the atmospheric pressure? Yup!

Normal air pressure is about 15 pounds per square inch. For a one inch column of water to weigh 15 pounds, it would need to be about 32 feet high.
Above 32 feet a vacuum would form and the water would not stay higher than that. This is the basis for early barometers. These were made with mercury because it’s super dense and therefore short enough to fit inside a room. Making a water barometer is a cool experiment if you have the time and space for it.
Do you see the barometer here? The sealed tube of mercury is inverted into an open dish of mercury, just like the experiment we did with the cup and water. As the room’s air pressure rises and falls because of changing weather, the height of the mercury will rise and fall.
(Click the image to go to the full painting)
6. Determining Density: An Experiment for Kids

This is the classic way to find the density of an object. While you can use anything that sinks, I prefer polymer clay. It’s sold under brand names Fimo and Sculpey, but there are off-brands too. The beauty of this clay is that it doesn’t dry out, doesn’t leave a residue, and you can use it in water.
But why clay? By using clay, you can show that density is a quality of a substance. It doesn’t change if you have more or less of the substance. Kids can calculate the density for two or three different-sized lumps to prove this to themselves.
Click the image to go to the lab directions.
7. How do Boats Float? A Buoyancy Lab
You can understand floating and sinking in two ways:
First, you can look at the way pressure changes with the depth or height of a fluid. As we saw in Activity #3 above, the pressure in a fluid depends on how deep the fluid is. The deeper you are, the higher the pressure is. So, if you’re standing in water, the pressure at your feet is higher than near your head. This difference in pressure causes a force that pushes you upward.

Do you float? It depends. You also have a downward force (your weight) so these two forces work against each other and the larger one wins.
Another way to look at sinking and floating is to realize that water holds up the water above it. If you could remove a chunk of water and replace it with another object of identical size, will that object float or sink? It depends. If the object weighs more than the same volume of water, then it will sink. If it weighs less, it will float. And if it weighs exactly the same, it will neither float nor sink but stay where you put it.
It’s this second idea that we’re exploring here. We’re determining how much water an object displaces and whether that amount of water weighs more or less than the object. The cool thing about this procedure is that you can use it with floating objects. Here the boat displaces an amount of water. If we collect and weigh this water, we see that it weighs more than the entire boat. Here we're using polymer clay which is cool because it won't float if it's a solid ball, but it does float if its shaped like a boat. You could also use a square of foil to shape an aluminum foil boat but it's a little less forgiving when trying to reshape it multiple times..
So the weight of the boat (a downward force) is less than what the water can support (the upward force) and the boat floats. If we loaded the boat with weights, it would still displace the same amount of water. When would it sink? At the point when its weight increased beyond the weight of the displaced water.
I like this setup because it’s simple and cheap to make and is easy to store.
8. Air Is Compressible: How to Deflate a Marshmallow
This activity uses two different pumps—one that pumps air into a bottle and one that pumps air out of a bottle. Can you think what beverage you might use each for?

I love using marshmallows for this since they’re soooo visual. This always draws a WOW from kids and they want to do it over and over. When you pump air in, the marshmallows contract and when you pump the air out, they expand. The marshmallows fatigue over time, but you can use them a few times for sure.
Here we’re back to exploring the idea that air pressure is a function of how much gas is inside a confined space. If you add more molecules to the space, the pressure goes up and if you take some out, the pressure drops. This doesn’t explain surrounding (ambient) air pressure or why that rises and falls, but it’s an important part of understanding.
9. Out with a Bang: Heat Causes Expansion

This is another not-to-be-missed activity that your students will want to try over and over. It’s simple and quick. I let them do it themselves, though I supervised closely.
Add a centimeter or two of water to an empty can. Place it on a hot plate until the water is at or near boiling. Using tongs, remove the can and invert it into a bowl of water. BANG! The can collapses instantly.
What’s going on? As you heat the water, it turns to gas and drives out much of the air that was filling the can. Since the water vapor is hot, it doesn’t take much to fill the can. When you place the can into the water, it cools and the water vapor condenses. The pressure in the can drops dramatically (since it’s sealed and no air can get in) and the higher outside air crushes the can.

Sometimes the can doesn’t get crushed, but fills with water. Can you see why? Here, the air pressure pushes water into the can until the air pressure inside and outside are equal. It’s the same explanation but with a different outcome. And if this happens, you can reuse the can for another try!
10. Local Pressure: Heat Causes Expansion
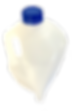
This is the last in our lineup. Here we add some very hot water to a milk jug and swirl it around to heat the plastic. Next we dump out the water and cap the jug and wait. Before long the jug implodes. It’s not as dramatic as the previous demo but it gets the point across. I appreciate doing different setups that focus on the same concepts. It helps solidify ideas.
Plus, we’re scientists, we repeat stuff.
As much as possible, we begin with concrete experiences that kids use to construct their understanding based on what they’re seeing. A sequence like this forms the basis of our comprehension and gives us something to discuss and return to again and again.
- Experiments & Activities
Recent Posts
Mitosis and Meiosis
Teaching Static Electricity Using VIPs
Dollar Store Optics
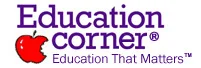
Top 10 Air Pressure Experiments: Fun & Easy

Are you ready to be blown away by some exciting air pressure experiments?
Air pressure experiments can be a great way to spark students’ interest in science and encourage them to explore the world around them.
These hands-on experiments help students better understand the properties of air and how it behaves under different conditions, such as changes in pressure or temperature.
1. Balloon-Powered DIY Drink Dispenser
Get ready to impress your guests with your very own balloon-powered drink dispenser and discover the amazing potential of air pressure!
This experiment showcases the principles of air pressure and fluid dynamics, making it an excellent opportunity for students and science enthusiasts to learn about these fundamental concepts in a fun and engaging way.
2. Make A Bottle Rocket
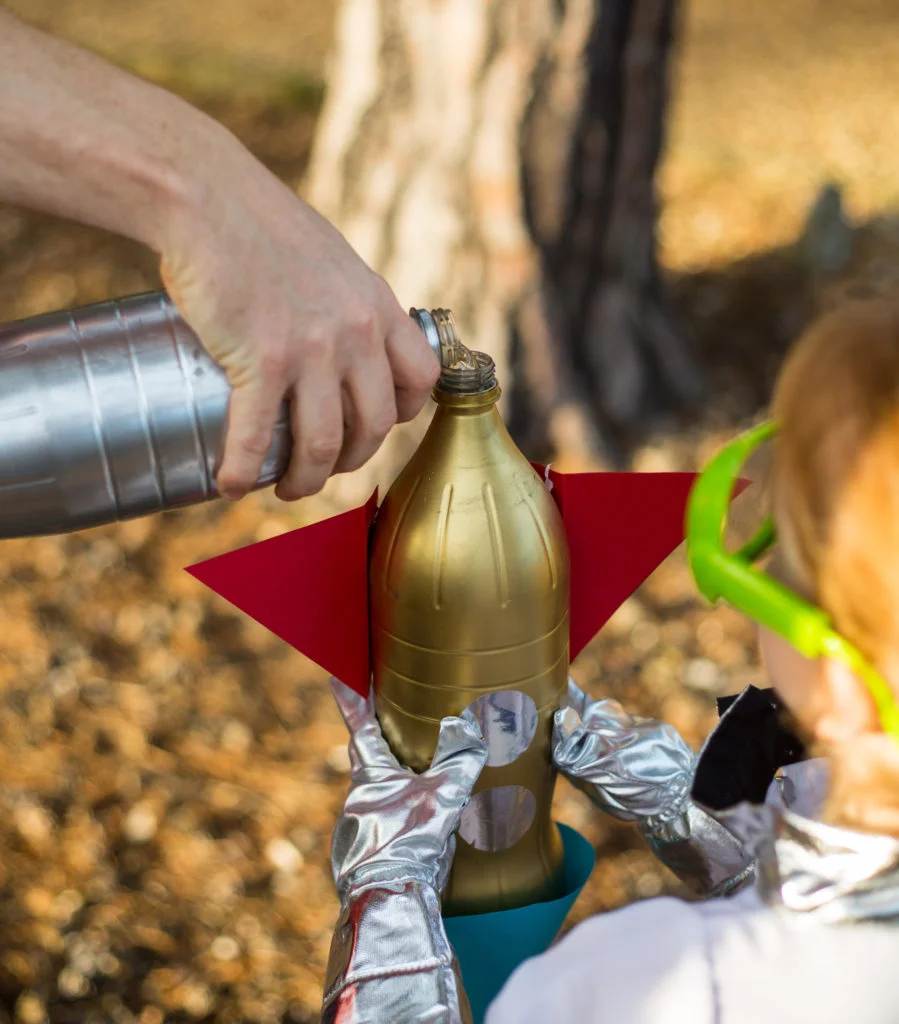
Get ready for lift-off with this exciting experiment that will have you launching your very own bottle rocket! By harnessing the power of air pressure, you can create a simple yet thrilling rocket that flies high into the sky.
Learn more: Make a Bottle Rocket
3. Flying Ping-Pong
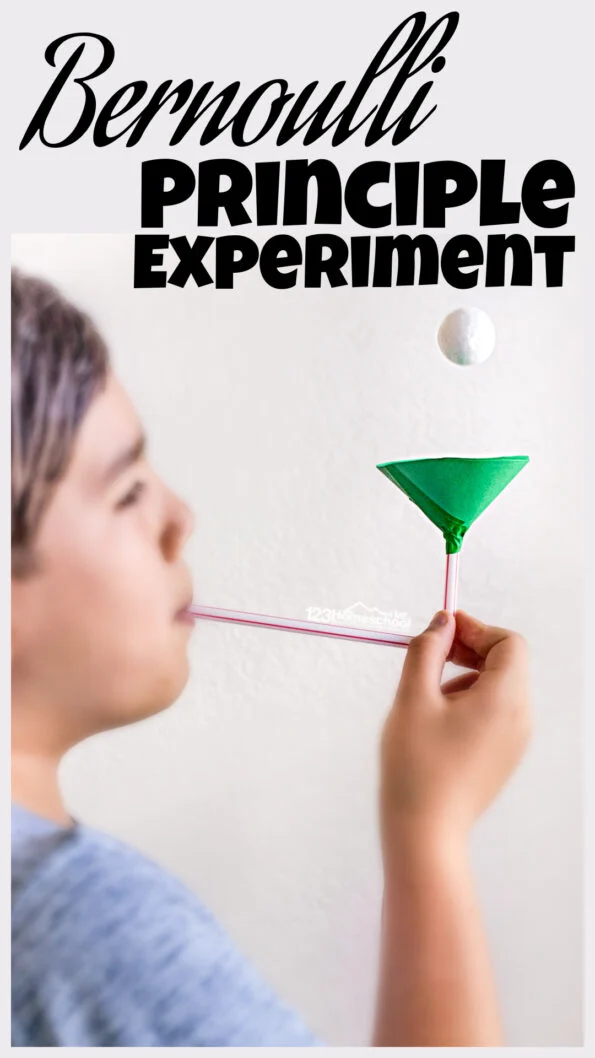
With one hand, place the ping-pong ball over the paper cone you’ve made, and with the other, blow a steady stream of air to cause the ball to levitate.
By gaining an understanding of Bernoulli’s principle, students can unlock the potential to design and create innovative solutions to real-world problems in a variety of fields.
Learn more: Bernoulli Principle for Kids
4. Air Pressure and Bottle
Get ready to witness a mind-blowing experiment that showcases the power of air pressure! By simply making a small hole in a plastic bottle and filling it with water, you can witness the incredible effects of air pressure at work.
5. Air-Powered Lift
Get ready to amaze your friends with this exciting experiment! With just a glass, a candle, and a plate, you can lift the plate using nothing but the power of air pressure.
6. Egg in a Bottle
With this exciting experiment using just a bottle, learn about the strength of air pressure! You may produce a variety of fascinating and unexpected effects by adjusting the air pressure inside the bottle.
7. Balloon Air Pressure Experiments
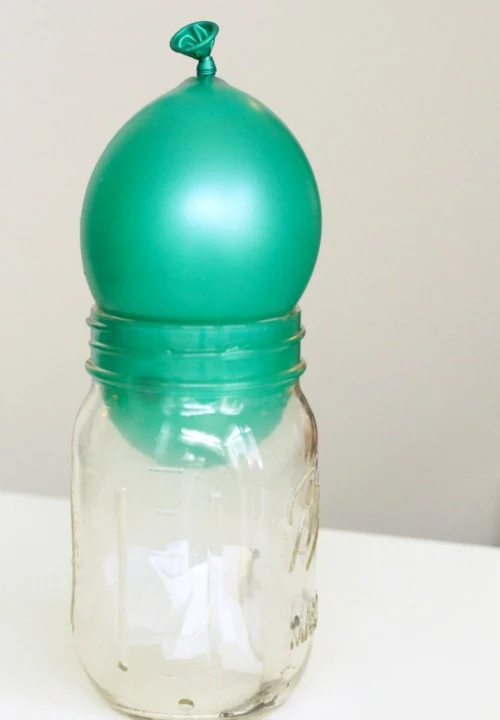
With this exciting experiment using just a bottle, learn about the strength of air pressure! You may produce a variety of fascinating and bizarre outcomes by regulating the air pressure inside the bottle.
Learn more: Balloon in a Bottle
8. Weather: Measuring Air Pressure
Get ready to become a meteorologist with this fascinating experiment that allows you to measure air pressure and predict changes in the weather!
By using a simple barometer made from a glass jar, a balloon, and a straw, you can measure changes in air pressure and use them to predict changes in the weather.\
9. Can Crush
The Can Crush experiment is a great demonstration of the effects of air pressure and it can be a fun and engaging activity for students.
10. DIY Model Lungs-Air Pressure Experiment
The balloon lung experiment is a fascinating demonstration that combines the principles of air pressure and the mechanics of the respiratory system.
Similar Posts:
- 68 Best Chemistry Experiments: Learn About Chemical Reactions
- 37 Water Science Experiments: Fun & Easy
- Top 50 Fun Food Science Experiments
Leave a Comment Cancel reply
Save my name and email in this browser for the next time I comment.
Your browser is not supported
Sorry but it looks as if your browser is out of date. To get the best experience using our site we recommend that you upgrade or switch browsers.
Find a solution
- Skip to main content
- Skip to navigation
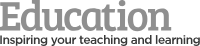
- Back to parent navigation item
- Find resources
- Experiments and investigations
- Cross-curricular activities
- Meet the scientists
- Boost your knowledge
- Beyond the classroom
- Get funding
- About the RSC
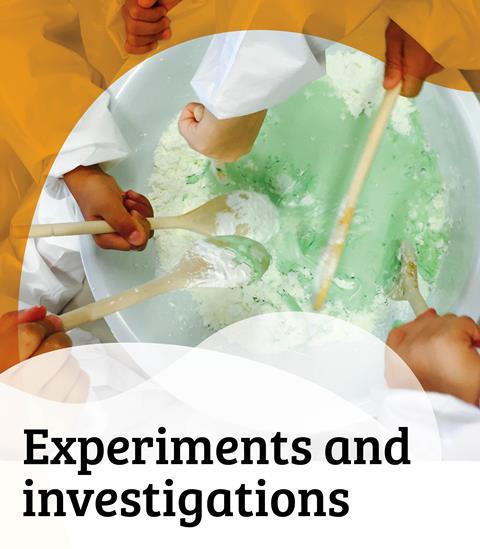
- More navigation items
Primary science investigations
- 2 Air pressure and the antigravity bottle
- 3 Air pressure, gases and the leaky bottle
- 4 Dissolving, density and ‘heavy’ sugar
- 5 Fizzy irreversible changes and bath bombs
- 6 Irreversible changes and the ‘fire extinguisher’
- 7 Irreversible changes and the ‘freaky hand’
- 8 Properties of gases, air pressure and ‘sticky’ cups
- 9 Properties of solids and ‘biscuit bashing’
- 10 Viscosity and ‘racing’ liquids
- 11 Freezing and the ‘intriguing ice’ experiment
- 12 Liquids, gases and the ‘lava lamp’
Air pressure, gases and the leaky bottle
- No comments
Try this simple investigation to explore the effects of air pressure
This resource is also available in Welsh and Irish

Get the Welsh language version .

Get the Irish language version .
This experiment focuses on air pressure, and can help develop learners’ understanding of forces, gravity and the properties of air. Watch the video of the ‘leaky bottle’ demonstration below, and then find out how your learners can explore air pressure themselves using rulers and newspaper.
Learning objectives
- To develop a simple definition of pressure in terms of force.
- To develop an awareness that the air around us exerts pressure on the objects it comes into contact with.
- To appreciate, through practical experimentation, that although air pressure is not often felt, its actions can be seen and explained.
Watch the video
The video below shows how to carry out the ‘leaky bottle’ demonstration.
Source: Royal Society of Chemistry
Investigate gases and atmospheric pressure with the Leaky Bottle experiment.
Download the supporting materials
Set up and run the investigation with your class using the teacher notes and classroom slides, featuring a full equipment list, method, key words and definitions, questions for learners, FAQs and more.
- Teacher notes
PDF | Editable Word document
Classroom slides
PDF | Editable PowerPoint document
DOWNLOAD ALL
What do learners need to know first?
Learners should already know that force is a push or a pull and that area is the space occupied by a flat shape or an object’s surface.
Equipment list
Leaky bottle demonstration (or per group if desired):.
- Plastic water bottle with screw-top lid
- Map/push pin
- Plastic tray to catch excess water
- Water to fill bottle
Main investigation (each group will need):
- 30 cm ruler
- Two identical sheets of newspaper
- Clear table top with a straight edge
Additional resources
- Investigate the affects of air pressure further in our anti-gravity bottle investigation or sticky cups investigation .
- Read up on solids, liquids and gases in this That’s Chemistry! textbook chapter .
- Introduce your learners to solids, liquids and gases with our primary science podcast .
Leaky bottle: teacher notes
Leaky bottle: classroom slides, additional information.
Primary science investigations were developed in collaboration with the Primary Science Teaching Trust
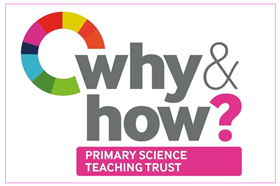
Air pressure and the antigravity bottle
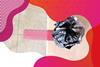
Dissolving, density and ‘heavy’ sugar
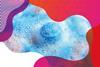
Fizzy irreversible changes and bath bombs
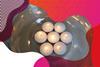
Irreversible changes and the ‘fire extinguisher’
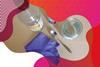
Irreversible changes and the ‘freaky hand’
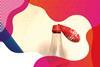
Properties of gases, air pressure and ‘sticky’ cups
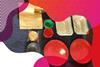
Properties of solids and ‘biscuit bashing’
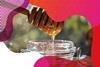
Viscosity and ‘racing’ liquids
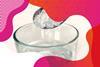
Freezing and the ‘intriguing ice’ experiment
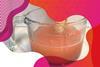
Liquids, gases and the ‘lava lamp’
- Practical experiments
- Properties of matter
Related articles
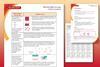
Particle diagrams | Structure strip | 14–16
By Kristy Turner
Support learners to describe and evaluate the particle model for solids, liquids and gases with this writing activity
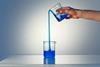
Illustrate polymer properties with a self-siphoning solution
2024-04-22T05:38:00Z By Declan Fleming
Demonstrate the tubeless siphon with poly(ethylene glycol) and highlight the polymer’s viscoelasticity to your 11–16 learners
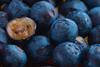
Revealing blueberries’ nanostructure
2024-03-22T11:00:00Z By Nina Notman
Find out how microscopic, self-assembling particles give blueberries their characteristic blue hue
No comments yet
Only registered users can comment on this article., more primary science.
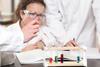
Catherine’s chemistry practical skills sessions
Find out how Catherine organised her chemistry practical skills sessions and get tips for applying to the RSC Primary Science Teaching Empowerment Fund
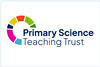
CPD support from the Primary Science Teaching Trust
Explore professional development resources, webinars and support from the Primary Science Teaching Trust.
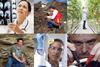
STEM careers and skills activities
Five out of five
Introduce primary learners to STEM careers and encourage them to explore their own skills. Includes a game, colouring poster, fact files and teaching notes.
- Newsletters
- Find your local education coordinator
Site powered by Webvision Cloud
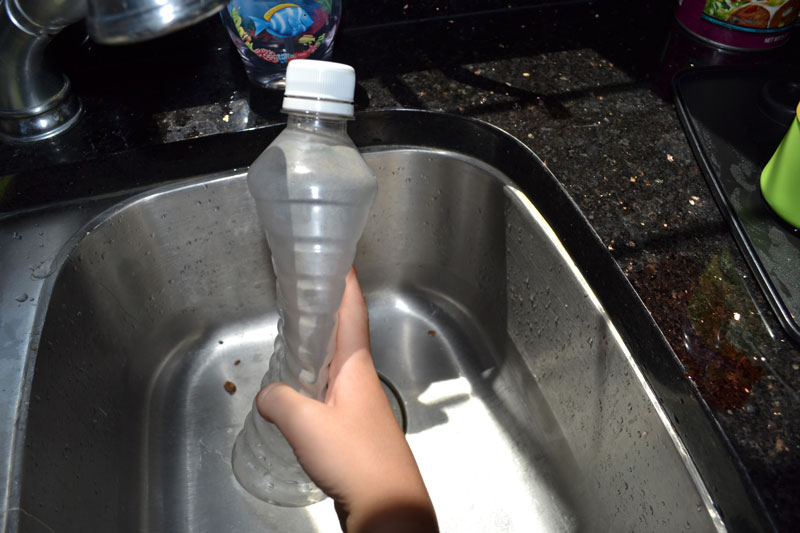
Air Pressure Experiment
A visual experiment to demonstrate air pressure.
Posted by Admin / in Matter Experiments
This experiment helps to show kids the power of air pressure by using temperature change to change the density of air. This is an very easy air pressure experiment and only requires a few common supplies to get started.
Materials Needed
- 2 clear water bottles with caps
- Refrigerator
EXPERIMENT STEPS
Step 1: Peel the labels off each water bottle so kids can see what is happening inside the bottles during the experiment.
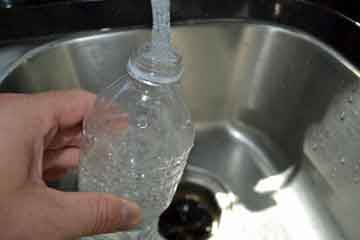
Step 2: Fill one water bottle with cool water.
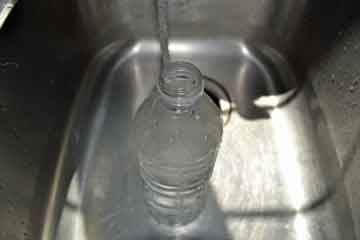
Step 3: Fill the second water bottle with hot water. Use hot pads, if needed, to avoid burning hands. Hot tap water works fine.
Step 4: Put the lids on the bottles and shake until the temperature of the plastic is consistent with the water inside the bottle.
Step 5: Remove the bottle caps and pour the water down the drain.
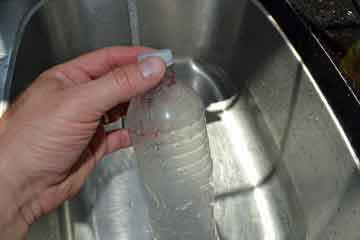
Step 6: Quickly screw the bottle caps back on the water bottles.
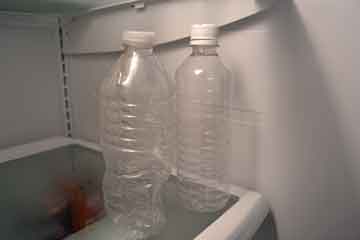
Step 7: Place both bottles inside of the refrigerator.
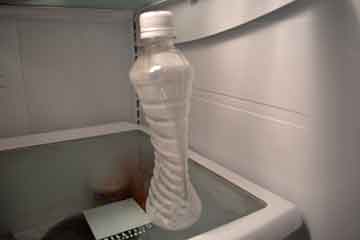
Step 8 : Wait about 5 minutes and open the refrigerator door and remove the bottles. Look at the shape of the bottles now.
SCIENCE LEARNED
The two water bottles behave completely differently after being placed inside the cold environment of a refrigerator. Nothing happens to the water bottle that was rinsed with cold water before being placed inside the refrigerator. The bottle that was heated by hot water, however, was crushed after being placed inside the cold refrigerator. Why?
The air inside the bottle which was heated with hot water expanded from the higher temperature. The expanded air was then sealed inside the bottle when the cap was tightened on the bottle. As soon as the air inside the hot bottle began to cool, negative air pressure was created as the air inside the bottle began to cool and contract. Placing the bottle inside the refrigerator amplified this result even greater. The air pressure difference between the air outside the bottle and inside the bottle was great enough to pull in the sides of the plastic bottle, crushing the bottle.
You can try to perform this experiment in reverse, by pouring hot water over the bottle to try to get the bottle to expand back to its original shape. This works to some degree, but is difficult because a lot of hot water is needed to heat the air inside the bottle enough. The plastic bottle acts like an insulator, but it will work if enough heat energy is added to bring the temperature of the air back up to the pre-refrigerated level.
Please subscribe to our YouTube channel to help us so we can make more videos for you!
Please click here to go to our channel and subscribe . thank you.
- About the author
- Back to Experiment
We like you too :)
Thanks for taking time to give us feedback!
- Matter science experiments
- science experiments for kids
- air pressure experiment
- demonstrate air pressure
- hot and cold air pressure
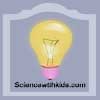
posted by Allen Douglas
- previous experiment
- next experiment
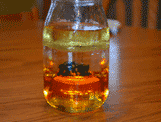
Liquid Density Experiment
in Matter Experiments
Experiment with the density of different types of liquids.
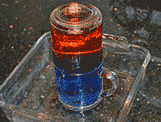
Hot and Cold Water Density
Use this simple experiment to demonstrate hot and cold water density..
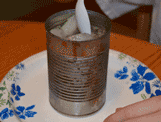
Water Cycle Experiment
Experiment to show all the phases of the water cycle.
- Skip to primary navigation
- Skip to main content
- Skip to primary sidebar
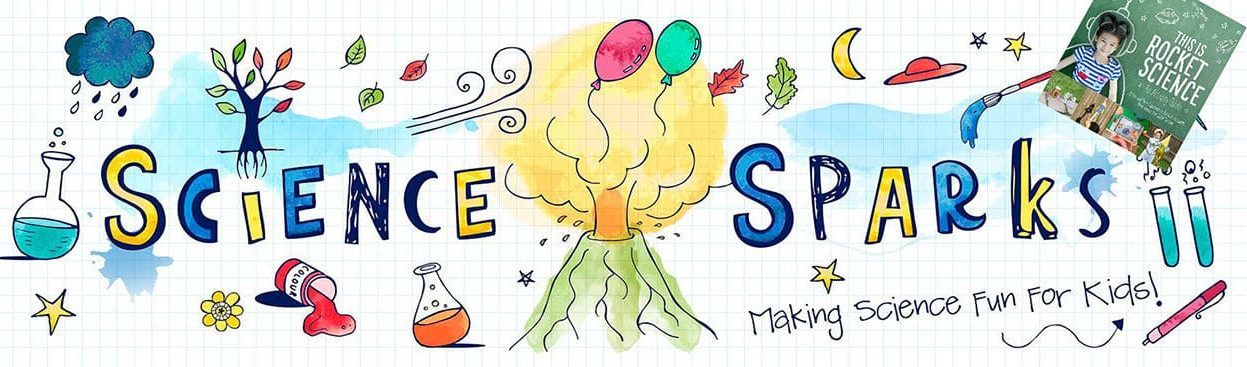
- FREE Experiments
- Kitchen Science
- Climate Change
- Egg Experiments
- Fairy Tale Science
- Edible Science
- Human Health
- Inspirational Women
- Forces and Motion
- Science Fair Projects
- STEM Challenges
- Science Sparks Books
- Contact Science Sparks
- Science Resources for Home and School
6 Air Pressure Experiments for Kids
May 26, 2014 By Emma Vanstone 2 Comments
These air pressure experiments are great fun and fantastic demonstrations of a fascinating force.
What is air pressure?
Air pressure is the weight of the atmosphere pressing down on Earth. We measure air pressure using a barometer . Have you ever climbed a mountain or visited an area at a high altitude and found you get out of breath more easily? This is because air pressure decreases at higher altitudes. Have you been on a plane and felt discomfort in your ears? This is due to changes in air pressure. Changes in air pressure allow for lots of cool science experiments. Here are some of our favourite air pressure experiments .
Air Pressure Experiments
Make a drinks dispenser.
This easy drinks dispenser is a brilliant science project and useful too! Party guests will wonder how this fun science trick works!
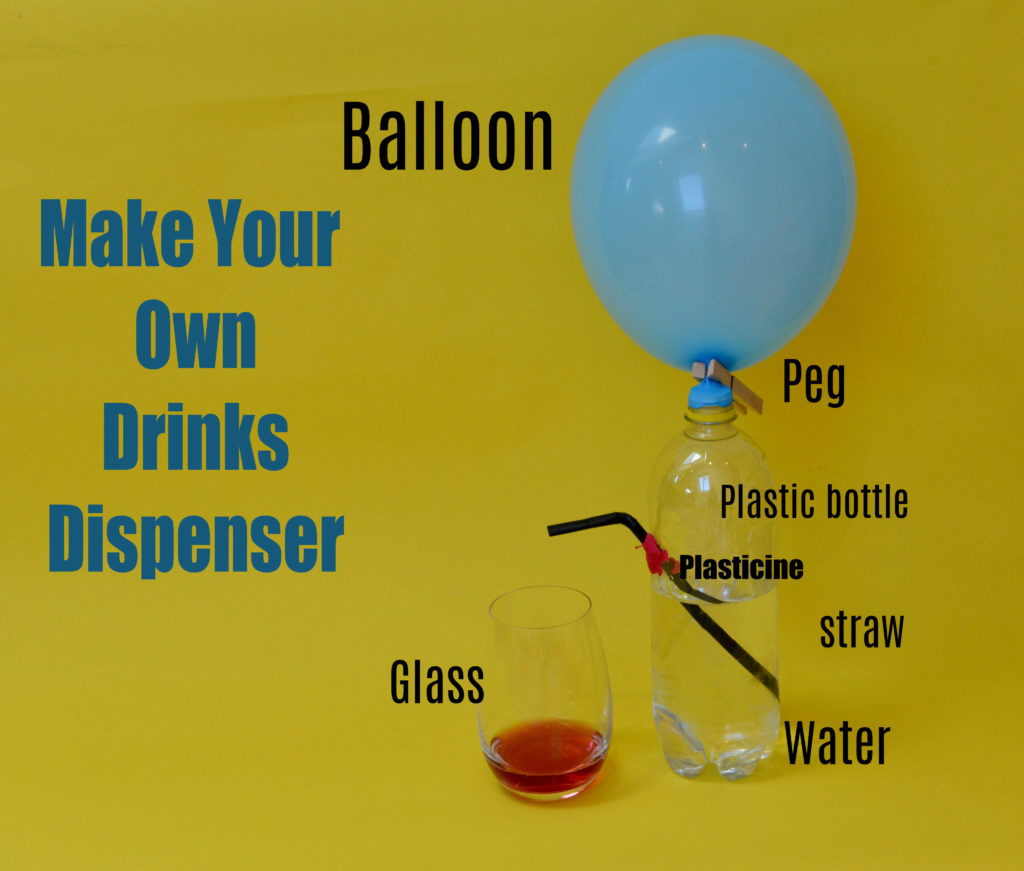
Make a Bottle Rocket
My favourite and the most impressive of these activities is this fantastic bottle rocket! Make sure you have a lot of space to launch the rocket, as ours flew with a lot more force than we expected.
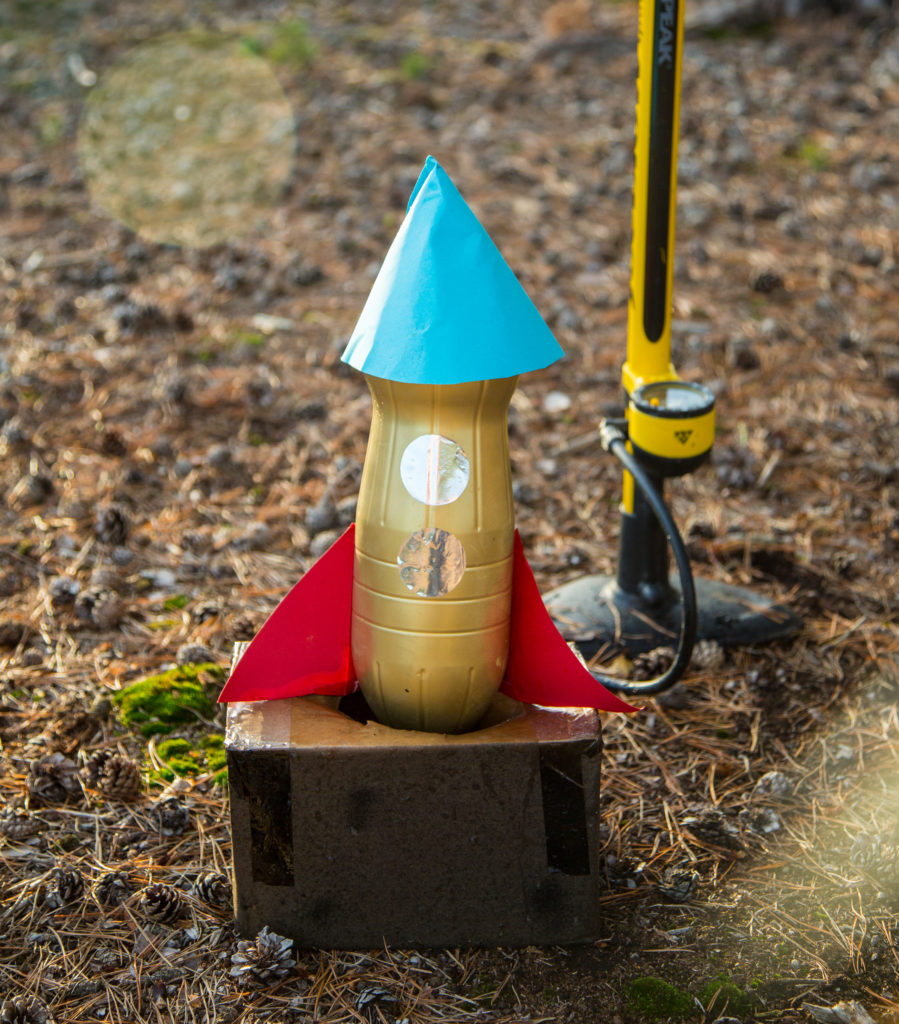
Blow Paper into a Bottle
Try and blow a ball of paper into a squash bottle and watch it fly back out. This one had my 6-year-old in hysterics and is a super simple demonstration of air pressure.
Make a Barometer and Measure Air Pressure
Record air pressure over a period of time with this easy to make barometer . You should find that air pressure varies with the weather and can be used to predict the weather.
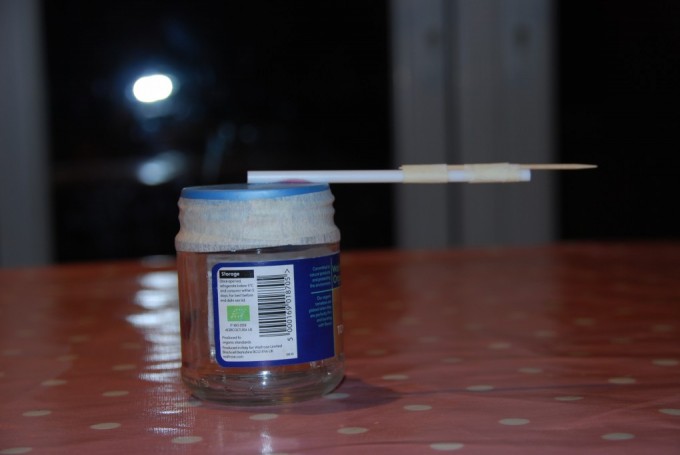
Egg into a Jar
Watch an egg magically drop into a jar . This happens because the air inside the jar is heated by the lit matches. After heating, the air starts to escape past the egg and as the air cools, the now higher pressure air on the outside forces the egg into the jar.
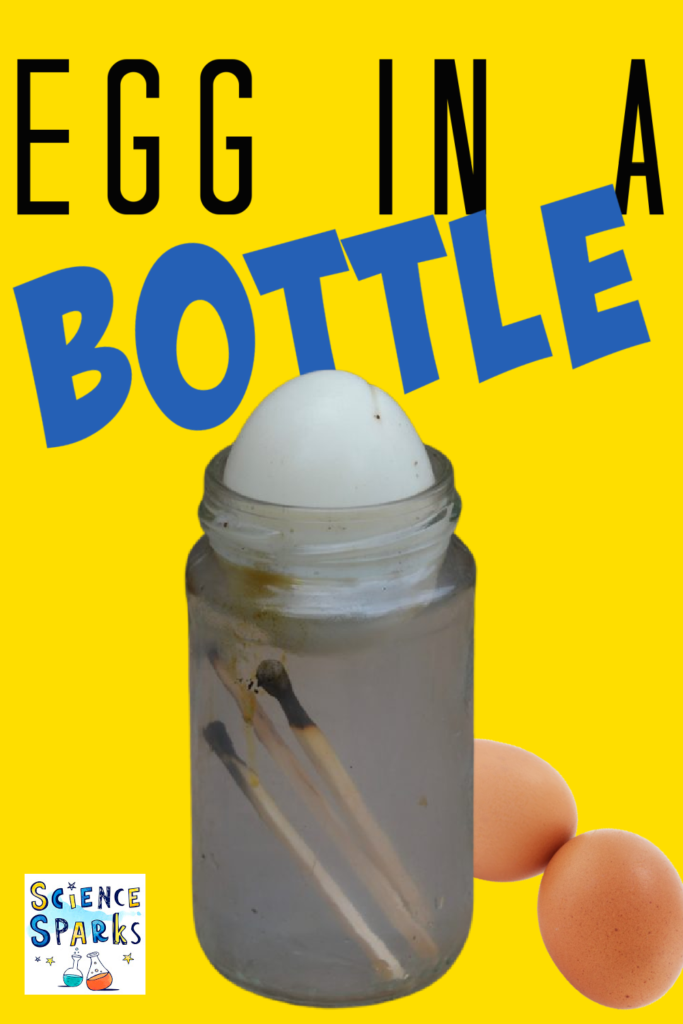
Make a Fake Lung
Find out how your lungs work with this fun model lung activity showing how changes in air pressure move air in and our of the lungs.
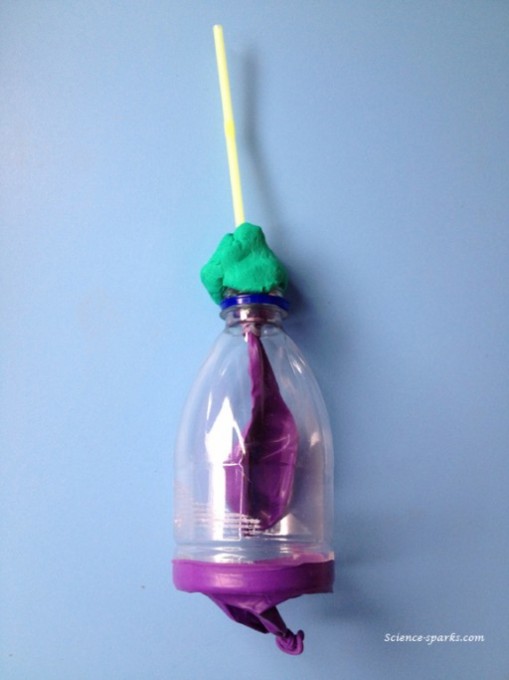
Rising Water Air Pressure Demonstration
Watch water rise up into a glass with this simple air pressure demonstration using a candle, water and glass.
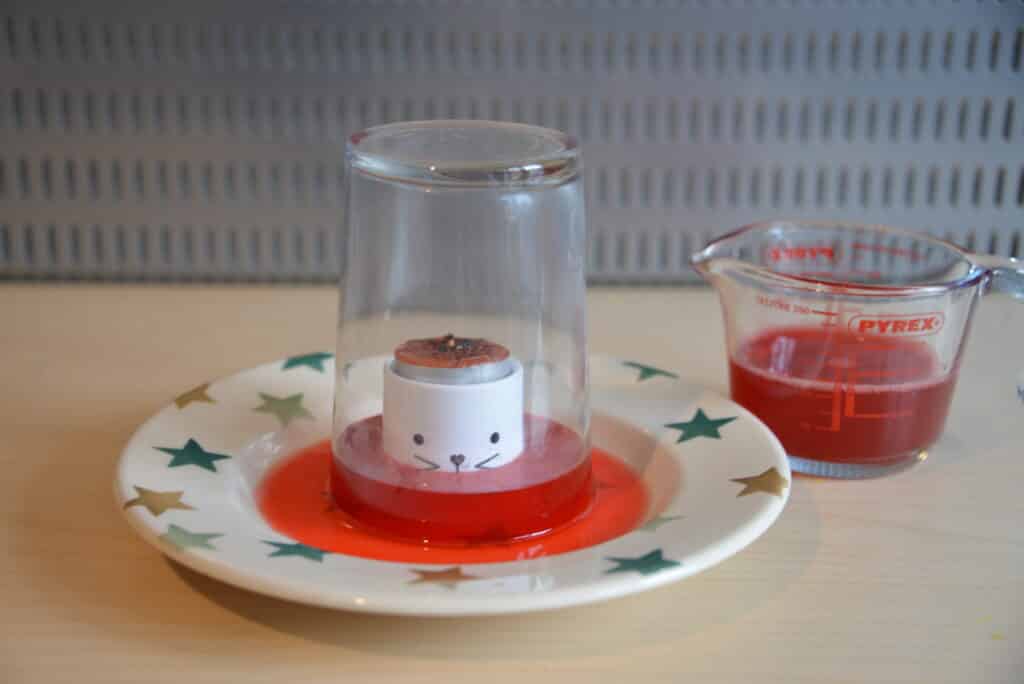
We also love this fun air pressure game on Kids Activities Blog .
Can you think of any more air pressure experiments or activity ideas to demonstrate air pressure for us?
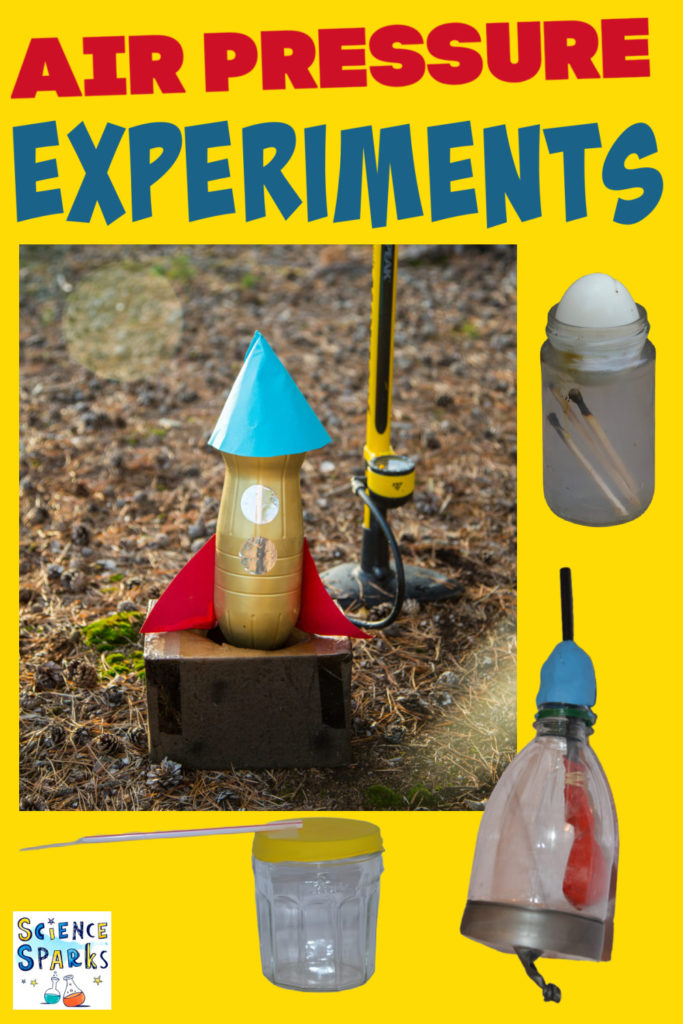
Last Updated on March 22, 2023 by Emma Vanstone
Safety Notice
Science Sparks ( Wild Sparks Enterprises Ltd ) are not liable for the actions of activity of any person who uses the information in this resource or in any of the suggested further resources. Science Sparks assume no liability with regard to injuries or damage to property that may occur as a result of using the information and carrying out the practical activities contained in this resource or in any of the suggested further resources.
These activities are designed to be carried out by children working with a parent, guardian or other appropriate adult. The adult involved is fully responsible for ensuring that the activities are carried out safely.
Reader Interactions
June 16, 2014 at 5:44 am
I am definitely going to try this experiments because kids love all these activities and there are generally very eager to experiment new things.
Leave a Reply Cancel reply
Your email address will not be published. Required fields are marked *
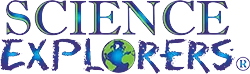
Balloon and Jar Air Pressure Experiment
by Science Explorers | Mar 29, 2021 | Blog | 0 comments
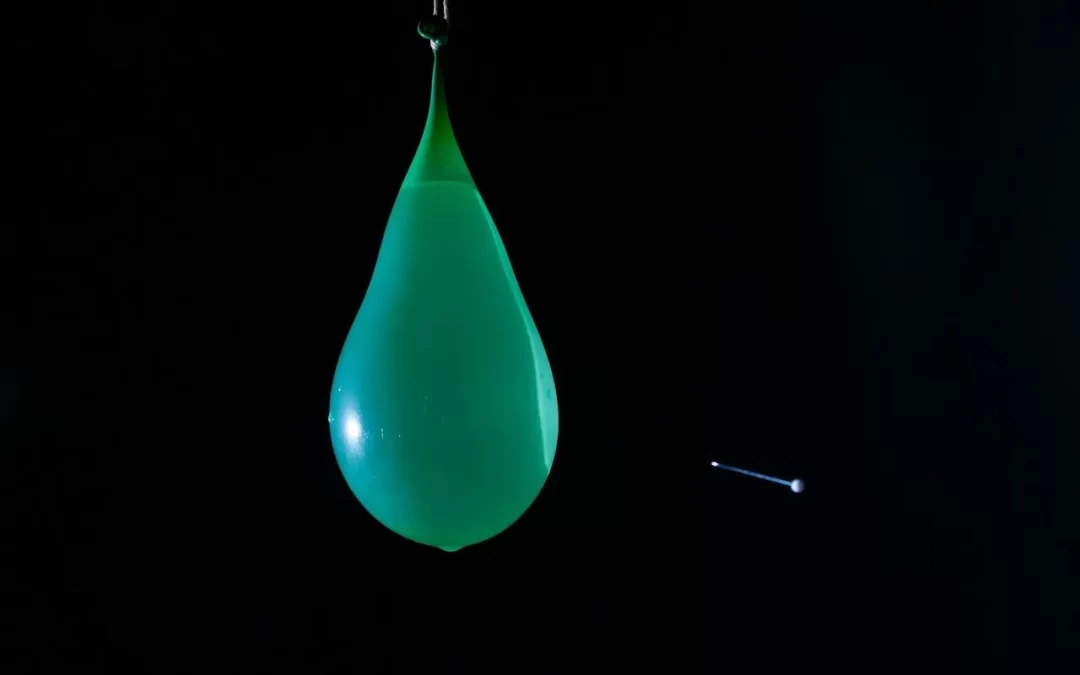
Air pressure experiments for children are a fun way to introduce kids to a new scientific concept. Kids and adults alike have a blast with this balloon and jar air pressure experiment. The experiment shows children what happens when the air pressure inside a jar changes by using just a few materials. It’s the perfect lesson for elementary school-age children with adult supervision.
Learn more about our virtual and in-person science camps!
What You’ll Need
To perform the experiment, you’ll need:
- Water balloon.
- Piece of paper.
Safety Note
This experiment uses fire. Children must be supervised and should not perform the experiment on their own.
How to Conduct the Experiment
Follow these instructions to suck a water balloon into a jar using air pressure:
- Fill the balloon: Fill the water balloon until it’s slightly wider than the neck of the jar and tie the balloon.
- Place the balloon on the jar: Place the jar on a flat surface and rest the balloon on top of the open jar.
- Demonstrate with the water balloon: Help the kids push down slightly on the balloon to show them it won’t fit inside the jar.
- Remove balloon: After demonstrating, remove the balloon from the container.
- Get your matches: Light a piece of paper with a match and drop it in the jar.
- Place the balloon again: When the fire starts to grow, place the balloon back over the mouth of the jar.
- Watch the reactions: Observe what happens to the balloon and the fire. The balloon will begin to shake, and the fire will be extinguished as the balloon is sucked into the jar. The balloon will be sucked about halfway into the container.
- Let the kids try: Once the fire has died and the jar has cooled, have the children try to remove the balloon. It will be a little challenging!
- Safely remove the balloon: To remove the water balloon from the jar, start by turning the jar sideways. Place your finger between the container and the balloon to release the suction. The balloon should come out easily after that.
Children will love doing this experiment over and over. To make this air pressure experiment even more fun for kids, let each child pick a balloon to decorate before you fill it with water. This allows children to observe any differences between how the balloons behave, such as which balloon was most difficult to remove and which one worked best.
The Science Behind the Experiment
This experiment is all about air pressure. When you first place the filled balloon atop the jar, air pressure prevents you from pushing it inside. The air trapped inside the jar has nowhere to go, since the balloon covers the opening. At this point, the air pressure within the jar is the same as the air pressure outside it, making it impossible to fit the balloon in.
But when you add the lit piece of paper to the jar, things change. The burning paper causes the air inside the jar to heat up and expand. As the fire grows, the air in the jar will start escaping around the sides of the balloon. When the balloon begins shaking that’s how you know the air is escaping.
The balloon acts as a one-way valve, allowing air within the jar to escape but preventing new air from entering. With less air in the jar, the air pressure drops. At this point, the air pressure within the jar is lower than outside it, which causes the balloon to get sucked in.
Learn About Science Summer Camps at Science Explorers
If you’re looking for more fun science activities for your child, we have the perfect programs at Science Explorers. Learn more about our virtual and in-person summer science camps and contact us for additional information.
Recent Posts
- 5 Earth Science Experiments: Safe, Exciting and Educational
- Beach STEM Activities
- Guide to Sports Science for Children
- Tips to Encourage a School STEM Program
- Activities to Teach Children About Hurricanes
Recent Comments
Choose an Account to Log In

Notifications
Science project, air is everywhere.

Grade Level: 3rd - 5th; Type: Physical Science
Objective:
To provide evidence that air is everywhere and takes up space.
Research Questions:
- What properties does air have?
- How can you prove that air takes up space?
- How can you prove that air has mass?
Air is everywhere! You might not be able to touch it or see it but it is all around us. Though invisible, you can easily see that air takes up space when you blow up a balloon. You can see the movement that it creates when a breeze blows through the leaves on a tree.
- Straight pin
- Balloons (at least 2)
- Piece of cardboard
- Short, wide-mouth jar
Experimental Procedure:
(Two-part experiment)
- Inflate two balloons and tie them. Use string to tie a balloon on each end of the ruler. Tie a short string to the middle of the ruler so that you can hold the string to where the ruler is suspended.
- Move the balloons until the ruler is balanced.
- Make a prediction of what will happen if one balloon is popped. Record your prediction.
- Pop one of the balloons using a straight pin. What happened to the ruler? Record your observation.
- Trace the mouth of the jar onto the cardboard. Cut the cardboard larger than the diameter of the jar.
- Fill the jar half full with water. Place the piece of cardboard over the top of the jar.
- Make a prediction about what will happen when you turn the jar upside down. Record your prediction.
- Turn the jar upside down while you hold the cardboard tightly in place. Take your hand off the cardboard. What happened to the water? Record your observation.
Terms/Concepts: Air; Matter; Mass; Properties of matter
References:
Science Turns Minds On . (1995) New York: McGraw-Hill
Related learning resources
Add to collection, create new collection, new collection, new collection>, sign up to start collecting.
Bookmark this to easily find it later. Then send your curated collection to your children, or put together your own custom lesson plan.

Advertisement
Science Projects for Kids: Air Pressure
- Share Content on Facebook
- Share Content on LinkedIn
- Share Content on Flipboard
- Share Content on Reddit
- Share Content via Email
There's air all around you -- but how much do you really know about it? Learn more with science projects for kids: air pressure.
When you think of air, you might think of emptiness, but air is actually exerting a force -- or pushing -- on everything, all the time.
This invisible force is called air pressure, and you can demonstrate it with a simple science projects for kids.
Follow the links below to learn how to perform your own science projects for kids involving air pressure:
Book Blast Experiment
Your books will blast off from the force of your breath. Learn more here.
Indoor Tornado Experiment
Capture a tornado in a bottle with this science project.
Unspillable Water Experiment
Turn this glass upside down, and the water stays put! Find out why.
Caved-in Can Experiment
Call on an invisible force to crush a can with this project.
Keep reading for a science project that will show you the power of a little compressed air.
For more fun science projects, check out:
- Fun Science Projects for Kids
- Science Projects for Kids: Weather and Seasons
- Science Projects for Kids: Light and Heat
Compressed air has great strength. The book blast experiment is a science project that demonstrates just how powerful it can be.
What You'll Need:
- Large airtight plastic bag
Step 1: Challenge a friend to move 3 books stacked on top of one another on a table by simply blowing at them. Of course, your friend won't be able to do it!
Step 2: Place a large plastic bag on the table, and put the 3 books on top of the bag. Leave the open end of the bag sticking out over the edge of the table.
Step 3: Hold the opening together, leaving a hole as small as possible. Blow into the bag. Take your time; stop to rest if you need to.
If you blow long and hard enough, the books will rise off the table. They will be supported by the compressed air in the plastic bag.
The next science project simulates a storm inside your own home. Keep reading science projects for kids: air pressure.
Do tornadoes make you dizzy? Do their spinning, twirling winds make you wonder how they work? Well, shake up a mini-tornado of your own with this wild indoor tornado activity and study its spiraling vortex of currents without fear.
- Clear mayonnaise jar
- Food coloring
- Liquid dish soap
Step 1: Fill an ordinary glass mayonnaise jar about two-thirds of the way with water. Add a few drops of food coloring (any color) to the water.
Step 2: Add a teaspoon of liquid dish soap and a teaspoon of vinegar. Screw the lid on good and tight to prevent leaks and extreme messes.
Step 3: Give the jar a good, hard shake, then give it a twist to set the liquid inside spinning.
Step 4: What you'll see is a tiny bottled vortex that looks just like a miniature tornado. Watch the spinning mini-tornado closely and you may even gain insight into the real thing.
Keep reading science projects for kids: air pressure for a science experiment that uses air pressure to make a wall of water.
Air pressure can be stronger than gravity. This unspillable water experiment demonstrates its strength as it keeps the contents of a water glass in place, even upside down.
- Juice glass
- 4x6-inch index card
Step 1: Fill a juice glass full of water. Let the water run over so that the lip of the glass is wet. Be sure that you fill the glass right up to the top.
Step 2: Place a 4x6-inch index card on top of the full glass of water. Be sure to press the card down securely with your hand so that it makes a good seal all around the wet lip of the glass.
Step 3: Working over a sink, hold the card in place with one hand as you turn over the glass. Carefully let go of the index card. The card will stay in place, and the water will stay in the glass.
What happened? The force of air pressure against the card is stronger than the force of gravity on the water. The air pressure holds the card in place.
In fact, the force of air pressure is so great that you can even use it to crush a can. Find out how with the next science project in science projects for kids: air pressure.
Air pressure is strong enough to bend a can. Find out how you can amaze your friends with the caved-in can experiment.
- Large container
- Empty soda can
- Measuring cup
- Tongs or pot holders
Step 1: Fill a large container with water and ice cubes. Set it aside to use later.
Step 2: Pour 1/2 cup of water into an empty soda can.
Step 3: With adult supervision, put the can on a burner on the stove.
Step 4: When the water in the can starts to boil, you will see steam coming from the hole in the top of the can. Turn off the stove, and use tongs or pot holders to remove the can from the heat.
Step 5: Quickly put the can in the container of ice water, turning it upside down to rest on its top. Now, watch the can collapse as it cools.
What Happened? When you heated the water in the can, it produced steam that forced the air out of the can.
When you put the can in the ice water, its temperature lowered, and the steam condensed back into water.
The pressure of the air outside the can was greater than the air pressure inside the can. The weight of the outside air crushed the can.
ABOUT THE ACTIVITY DESIGNERS
Indoor Tornado Experiment by Maria Birmingham, Karen E. Bledsoe, and Kelly Milner Halls
Please copy/paste the following text to properly cite this HowStuffWorks.com article:
- ASME Foundation
- Sections & Divisions
- Sign In/Create Account
5 Ways to Demonstrate Air Pressure to Children
- Topics & Resources
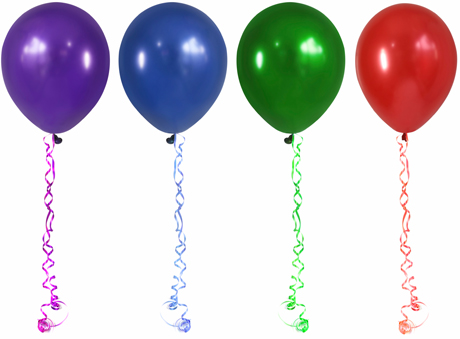
Date Published:
Nov 22, 2010
Aurora Lipper
This story was updated on 10/11/2022.
Note: when conducting at-home science experiments with children, an adult should always be present. Even the simplest experiments have the potential to go wrong.
The ordinary pressure of the air surrounding us is 14.7 pounds per square inch—but this can change based on a few factors, such as when the wind blows or a car or airplane accelerates. Wherever the air pressure is higher, there will be a stronger force or push against an object. Similarly, when an air particle speeds up, it actually “pushes” less.
Imagine that fast-moving air particles are in so much of a hurry that they don’t have time to apply force—this is the principle used to make airplanes fly. When a plane moves along the runway, the air above the wing speeds up, lowering the pressure so that the air below the wing can push the plane upward.
Interested in testing out these principles in a more tangible way? Try one or more of the following experiments:
Water Glass Trick
Step 1: Fill a cup one-third with water.
Step 2: Cover the entire mouth of the cup with an index card.
Step 3: Holding the card in place, take the cup to the sink and turn it upside down.
Step 4: Remove your hand from underneath.
Voilà! Because the water inside the cup is lighter than the air outside, the card is held in place. This is due to about 15 pounds of force from the air pushing up, while the force of the water pushing down is only about one pound of force.
Fountain Bottle
Step 1: Fill a 2-liter soda bottle half full of water.
Step 2: Take a long straw and insert it into the mouth of the bottle.
Step 3: Wrap a lump of clay around the straw to form a seal.
Step 4: Blow hard into the straw—then stand back.
When you blow into the straw, you’re increasing the air pressure inside the sealed bottle. This higher pressure pushes on the water, forcing it up and out of the straw.
Ping-Pong Funnel
Step 1: Put a ping-pong ball inside the wide part of a funnel.
Step 2: Blow hard into the narrow end of the funnel.
Step 3: You’ll notice that the ball doesn’t pop out of the funnel—but why?
This is because as you blow into the funnel, the air moves faster and lowers the air pressure underneath the ball. Because the air pressure is higher above the ball than below it, it’s pushed down into the funnel—no matter how hard you blow or in which direction you point the funnel.
The Million Dollar Bet
Step 1: Place an empty water or soda bottle down horizontally on a table.
Step 2: Roll a piece of paper towel into a small ball about half the size of the bottle opening.
Step 3: Tell a friend you’ll pay them one million dollars if they can blow the ball into the bottle.
Don’t worry about losing money—because this is impossible. No matter how hard someone tries to force more air into the bottle, there's no room for it. The air will flow right out, pushing away the paper ball.
Kissing Balloons
Step 1: Blow up two balloons and attach a piece of string to each.
Step 2: Place one balloon in each hand, holding them by the string.
Step 3: Position the two balloons so they are at your nose level and six inches apart.
Step 4: Blow hard into the space between the balloons.
As you lower the air pressure in that space between the balloons, the pressure of the surrounding air becomes higher. This automatically pushes the balloons together, causing them to “kiss.”
[Adapted from “Top Ten Air Pressure Experiments to Mystify Your Kids-Using Stuff From Around the House,” by Aurora Lipper, for Mechanical Engineering , January 2008.] Read More: How to Mentor Young Engineers Experiential Learning and Cooperative Education Pay Off Engineering Education, Family Style
Related Content
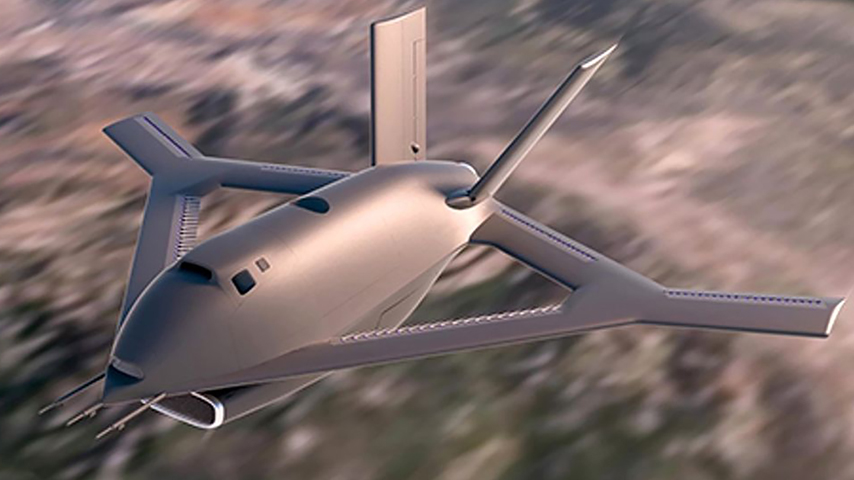
ASME Membership (1 year) has been added to your cart.
The price of yearly membership depends on a number of factors, so final price will be calculated during checkout.
You are now leaving ASME.org
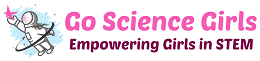
How to do an Air Pressure on Water Experiment for Kids
- September 26, 2021
- 5-6 Year Olds , 7-9 Year Olds , Physics
Have you ever wondered how water can be pulled up into a straw? Or what happens to the air pressure when you go up in an airplane?
Now, you can explore these questions with this awesome experiment. The science behind this phenomenon is air pressure.
If you have ever had a bike pump, then you know that the higher the pressure inside, the more forcefully it can push air out . This same principle also applies to water being sucked up through your water dispenser.
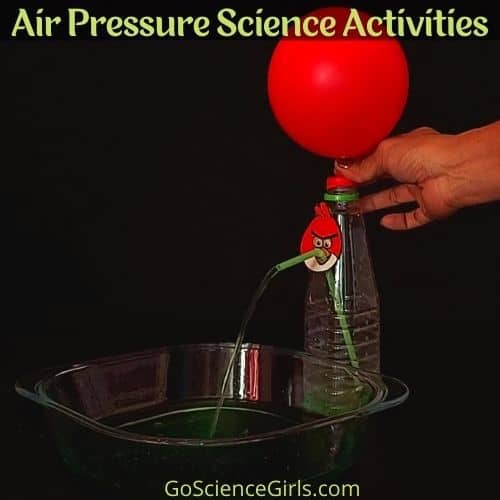
Air Pressure and Water Experiment Supplies
Air Pressure on Water Experiment is an awesome physical science experiment as it can be done using simple and easily available supplies. Here is the list of items you need to collect before you start the experiment.
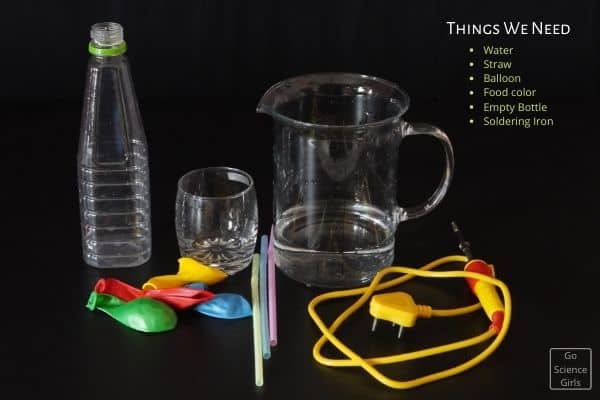
1) A Balloon
2) A Plastic straw
4) A small container (glass one is better to get a good visual experience) or a Peg
5) Putty or Plasticine
6) A Plastic water bottle (Make sure it is clean and clear no matter the shape and size.)
7) Food Color
8) Some other miscellaneous things like kids-friendly knife, glue, metal scale, etc.
Directions to do Air Pressure on Water Experiment
Step-1: Select a place where you feel free to do experiments with water like sinks or outdoors as this activity messy up things with water.
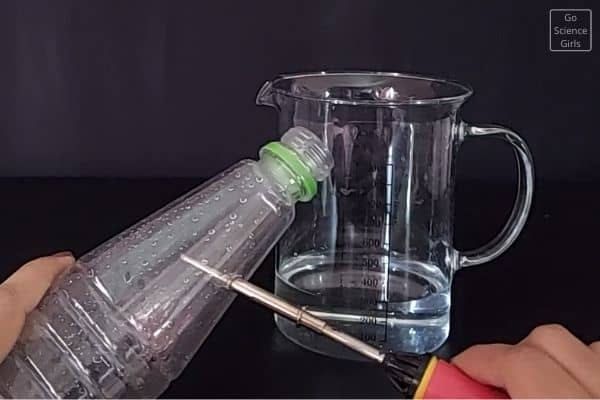
Step-2: Now, pick an old and clear plastic bottle and make a hole of 10cm at the middle of the bottle. You can use kid-friendly knife or scissors to make hole. Whatever the tool you use, make sure you are moving it clock and anti-clock wise direction to achieve a good round shape. You can also use a soldering rod to make exact size hole as of straw.
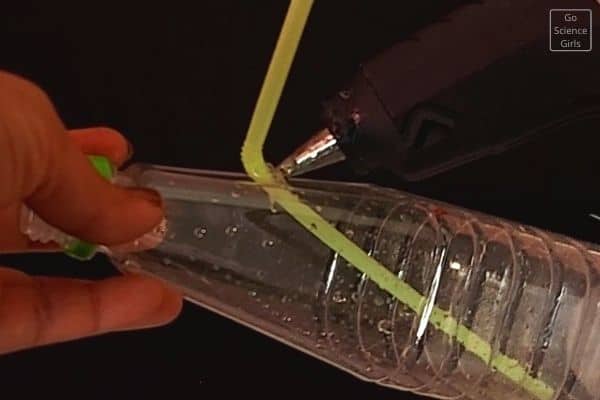
Step-3: Once the hole is ready, insert the plastic straw into it and seal any leakages around the hole using hot glue. You can also use putty or plasticine to seal it.
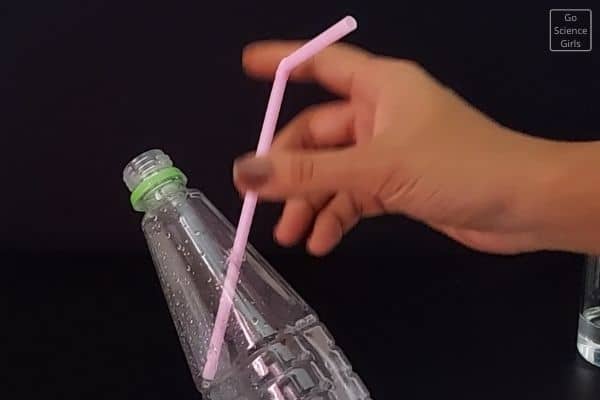
Step-4: Then, take a small container and add some amount of water. Also add a few drops of food color and into the water to make it colourful. This colourful water is good to experiment with as it gives good visual experience of the experiment.
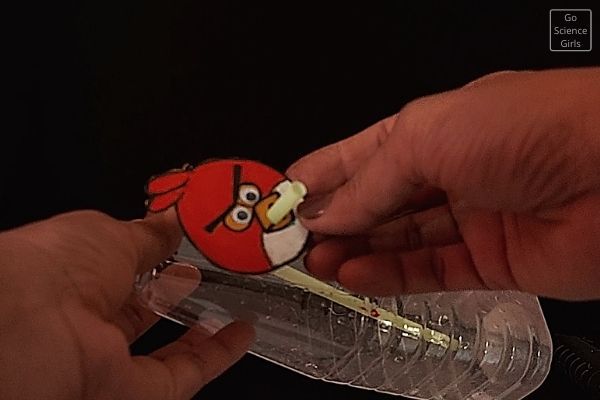
Step-5: As a next step, pour the color water into the plastic bottle fixed with straw up to half way. And keep a peg or small glass or transparent container under the other end of the straw which is hanging outside of the bottle.
Step-6: In this step, take a medium sized balloon and inflate it using your mouth or any balloon blower machine. Seal the mouth of the inflated balloon and fit it around the mouth of the bottle carefully. At this point, our school science fair project set up to show Air Pressure is ready for demo.
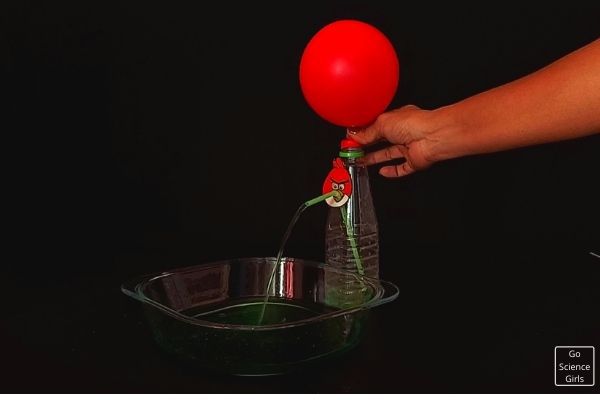
Step-7: Now, release the secured mouth part of inflated balloon such that the air inside it goes straight down into the plastic bottle.
Step-8: You will notice the color water inside the plastic bottle moving out of the straw towards the small container placed beside the plastic bottle under the open end of straw.
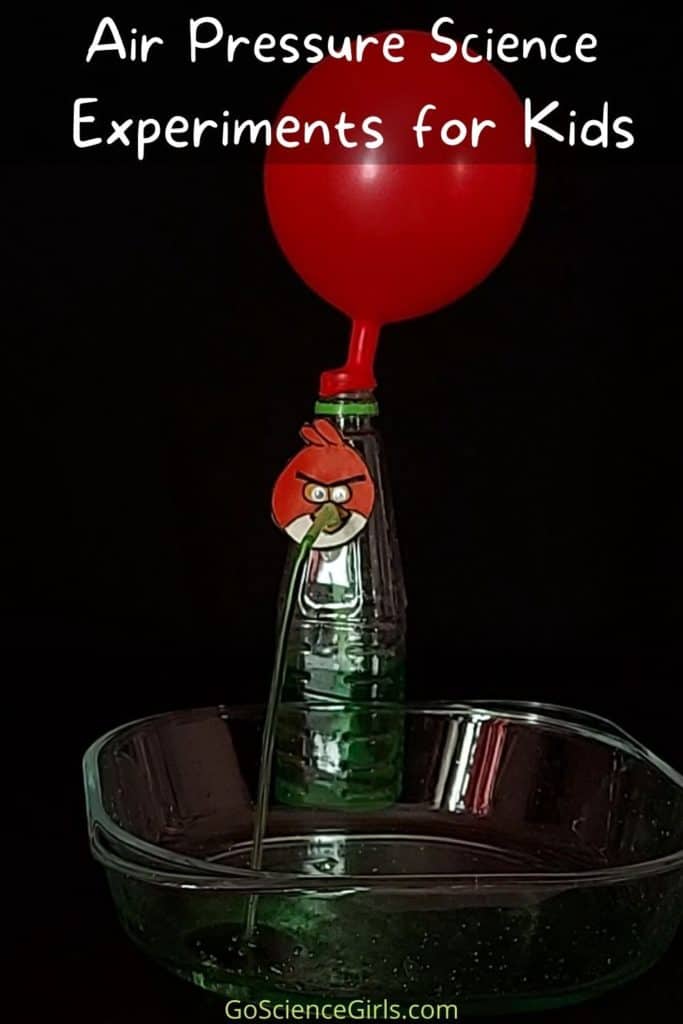
What is the science behind the water movement in this experiment?
In this physical science project, the air gives pressure equally on the water and inside straw when there is no balloon around the mouth of the plastic bottle.
But when the inflated balloon is set up on top of the plastic bottle i.e. around its mouth part, the air inside the balloon forces down into it.
Thus, creates increased pressure on the surface of the water and presses the water molecules down due to force and gravity. This increased pressure inside the water pushes water into the straw. And hence the water moves towards the straw and stores in the small container placed outside.
The science concepts learned through this classic Air Pressure Experiment on Water include:
1) Atmospheric Pressure: The pressure applied by the molecules (that has weight) present in the atmosphere or air towards the surface of the Earth due to the force and gravity is known as Atmospheric Pressure. Atmospheric Pressure is also known as Barometric Pressure.
2) Pressure: The amount of force exerted per unit area byt the surrounding surfaces and particles is known as pressure. The pressure unit is pascal. The formula of pressure is P=F/A. Absolute pressure, atmospheric pressure are the types of pressure.
3) Potential Energy: The energy developed due to the pressure or force with in itself is known as potential energy. Potential energy can also use electric charges to build its energy.
In this experiment, the air inside the inflated balloon develops potential energy and tries to come out of it. And hence, the water feels pressure when the mouth of the inflated balloon releases.
This pressure makes the water flow out of the plastic bottle and into the small container placed beside it through the straw.
Other Air Pressure Experiments You Can Try at Home
Balloon and Pin Experiment
Egg in a Bottle – Air Pressure Experiment
Balloon in a Bottle : Air Pressure Experime nt
Drip Drop Bottle-Water Bottle Pressure Experiment
What we learn from Air Pressure on Water Experiment
- Students learn about Air and Atmospheric pressure
- Explore different types of forces, pressures, and potential energy
- Get knowledge on various science terms such as atmospheric pressure, force, pressure, stress, etc.
- Can be a great science fair idea
- Encourages children to actively participate in science work-shops and events
How do you define Air Pressure?
Air pressure is the pressure created by the weight of the particles in the air that are forcing to move down to Earth because of gravity.
In simple words, air around us encompasses of a lot of air molecules (that has weight), which exerts pressure whenever they get in touch of any objects. This is the pressure we call it as Air Pressure.
Air pressure is also known as Atmospheric Pressure. As we use Barometer to generally measure atmospheric pressure, we also call it as Barometric Pressure.
The standard unit of atmospheric pressure is equivalent to 101,325 Pa or 29.9212 inches Hg or 760 mm Hg or 14.696 psi.
P_h= P_0 e^{\frac {-mgh}{kT}}
P_0= sea level pressure
P_h= pressure at height h
g= Acceleration due to gravity
K= Boltzmann’s Constant (Ideal gas constant divided by Avogadro’s number)
T= Absolute Temperature
M= Mass of one air molecule
Safety Measures
1) It is highly recommended to wear safety glasses to protect your eyes.
2) Handle the hot glue or hot melting glue carefully otherwise children may burn their hands
3) Use kid-friendly knife
4) Always there must be an adult supervision while conducting this experiment
When you inflate the balloon, the air inside it creates potential energy and faces pressure against the rubber surface of balloon. And when the inflated balloon placed over the neck of the plastic bottle, the air pressure of balloon flows into it. This creates higher pressure on the top of the plastic bottle and thus creates even more pressure inside water in the bottle. This pressure moves water from the plastic bottle to the container placed beside it through the straw.
There are many ways to demonstrate pressure through our daily activities. Here is the easy one to explore or demonstrate pressure: 1) Take a plastic water bottle and fill it with water to its half way. 2) Place a straw into its neck part and seal the leaky edges using putty or clay. 3) Blow heavily into the straw which creates increased air pressure inside the bottle. 4) This increased pressure inside the bottle pushes the water out of straw like a fountain.
Water dispenser plays important role in restaurants, hotels, offices, etc. And it is the perfect example to demonstrate Air Pressure. Water dispenser is useful to supply normal to moderate to hot water whenever we press button. Water dispenser is a set-up of upside down 4-5 gallon water bottle at the top of the machine. Mostly, water dispensers work by pressing button, through which you are increasing the pressure inside by allowing the air inside the bottle. And that’s the way, you can dispense water from the machine when air allowed inside the bottle.
Air has mass as it contains a lot of tiny particles that possess weight. These particles when touched against any solid object, exerts pressure. The pressure exerted by air molecules in all directions around us, known as air pressure. However, because of the air particles weight, we experience more air pressure when we stay closer to the surface of Earth.
Take one litre plastic bottle and make a straw size hole at the middle of it. Insert a straw through the hole and seal the leakages using clay or putty. Outside the bottle and under the other open end of the straw, place a small container. Now, fill half of the bottle with water and cover its mouth using inflated balloon. When you release the air inside the balloon, there creates high pressure inside the bottle and water. Thus, the water is let outside the bottle through the straw and into the container placed beside the bottle.
Here are some of the situations where we use air pressure in everyday life: 1) When we drink through straw, the air pressure inside it decreases while outside pressure increases and forces the drink to suck inside the straw. 2) Consider a vacuum cleaner, it has a fan inside it which creates low pressure environment inside the machine. Whereas the outside atmospheric pressure forced inside and takes the dirt and air molecules to suck inside the machine. 3) Syringes creates pressure by plunging the nob of it while taking the blood from human body. This pressure sucks the blood from human body into the syringe easily.
1) The pressure of air inside car tires holds the car weight 2) The flight movement up in the sky because of the air pressure on its wings. 3) Bullet firing from the gun using gas pressure. 4) Inflation of balloon because of air pressure developed inside. 5) Sucking a drink through straw using pressure created inside it.
Leave a Reply Cancel Reply
Your email address will not be published. Required fields are marked *
Name *
Email *
Add Comment *
Save my name, email, and website in this browser for the next time I comment.
Post Comment
Get Your ALL ACCESS Shop Pass here →

Air Resistance STEM Activity
Whoa! STEM in under 10 mins; all you need to do is grab some paper! Let’s explore air resistance with a few sheets of paper! Let’s answer the question, what is air resistance? Then, find even more fun and easy activities to demonstrate air resistance for kids’ physics. Look for free printable science worksheets. We love easy STEM activities for kids!
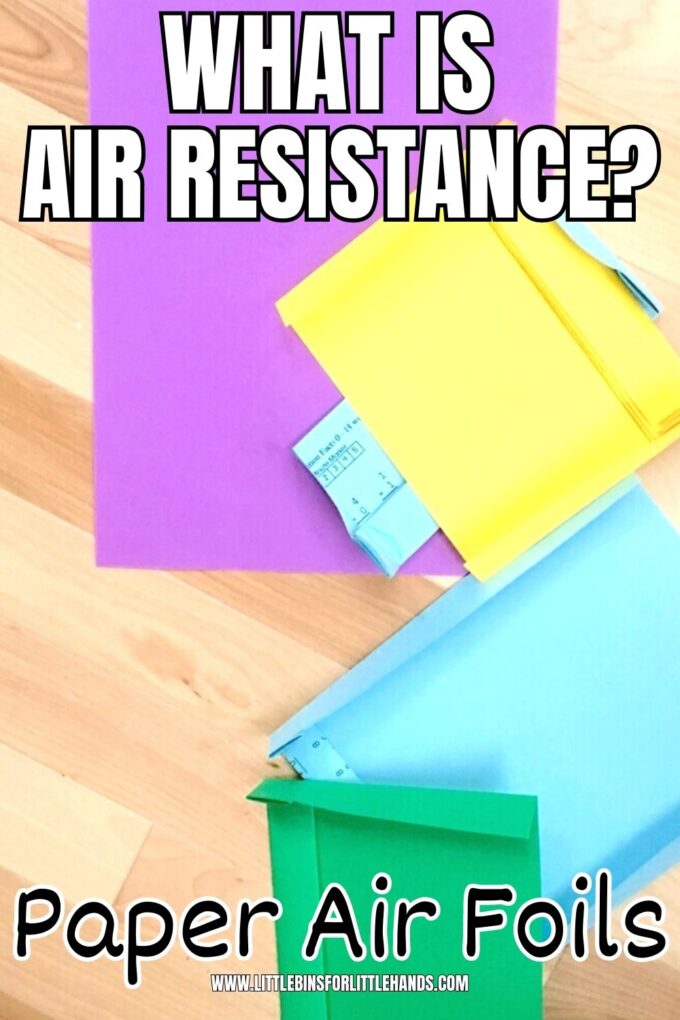
What is STEM?
STEM stands for science, technology, engineering, and math and i so essential to incorporate it into lesson plans.
We have put together a handy STEM resource here.
This fantastic air resistance STEM activity below requires so little setup and uses simple-to-grab supplies. We happened to have a bunch of colored computer paper, but ordinary white paper will do too!
Check out more fun physics for kids here.
We checked out a cool book from the library called Making Origami Science Experiments Step by Step by Michael LaFosse. We found this little gem of a STEM activity, building paper airfoils using simple origami folds.
I hadn’t considered combining origami and STEM, but it is the perfect project, especially if you have a few minutes. Learn more about air resistance below.
Kids of all ages can participate in this activity! The younger kids will happily enjoy this playful STEM activity and can talk about what they see. While older kids can take notes, record observations, draw conclusions, and develop more experiments (see the science process below)!
There are many ways to extend this activity into a longer lesson, and I will share more air resistance activities below. Plus, we have a handy free printable you can download at the end of this post.
ALSO CHECK OUT: Easy STEM Activities and Science Experiments With Paper
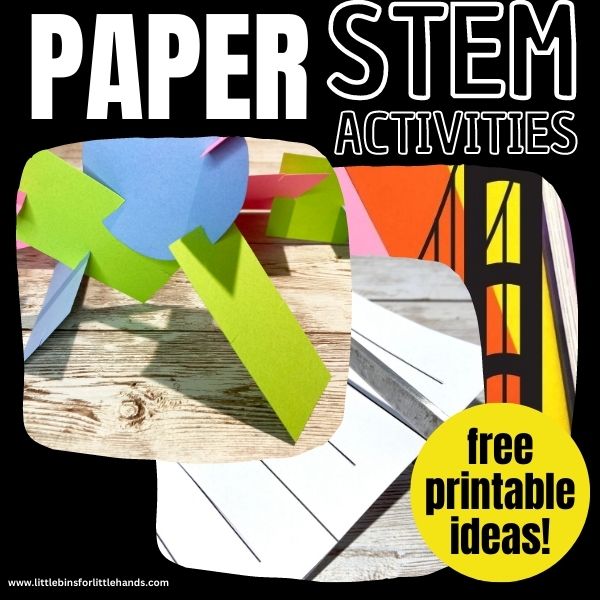
What is Air Resistance for Kids?
Of course, you want to add some of the science behind this air resistance STEM activity! How does air resistance affect the speed of a falling object like a paper air foil? I bet you have already figured that one out!
Air resistance is a force that pushes against things as they move through the air. When you throw a ball or ride a bike, the air around you pushes back, trying to slow you down. This force is called air resistance. Have you ever stuck your hand out of a car window while it’s moving? You can feel the air pushing against your hand, right? Or how about on a windy day, you try to run into the wind or away from the wind; what do you notice? That’s air resistance at work!
An object’s size, shape, and speed affect how much air resistance it experiences . Compare a feather and a rock! If you drop a feather and a rock from the same height, the feather takes longer to reach the ground. Why? A feather experiences more air resistance because it is lighter and has a greater surface area.
You might also like to try some of these fun gravity activities !
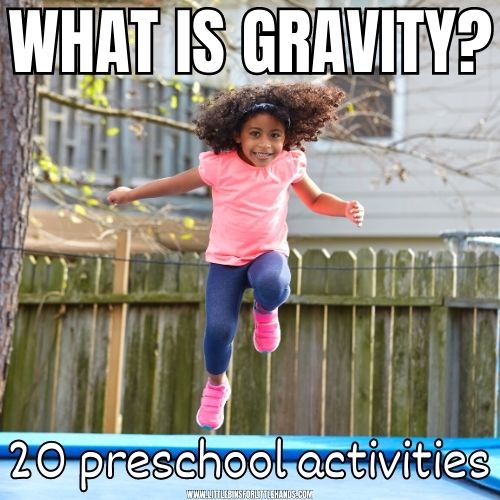
What else does air resistance help with? What about helping birds fly or slowing down a parachute’s fall? Sometimes air resistance can be a problem! Think about a time you wanted to ride your bike faster but felt like the wind pushing against you. Air resistance is all around us.
Scientists and engineers study air resistance to design better cars, planes, and sports equipment. They try to make things more streamlined to reduce air resistance and make them move faster and more efficiently. What about a bike helmet? Think about the car racing you see on tv! Have you ever entered a Pine Wood Derby as a Cub Scout or Boy Scout?
Add This Free Science Process Pack to Your Experiment

Air Resistance Experiment
- Printer/Computer Paper
- Origami Science Book (optional for this activity)
- Other objects to explore air resistance (optional)
All you need is a few sheets of paper, an open area, and our handy STEM activity printable sheet if you want to extend the lesson. This is a great place to experiment. As a result, you will want to have some trial runs with different air foils. Learn more about the scientific method for kids .
INSTRUCTIONS:
PART 1: To start, you want a control test which will just be your unfolded piece of paper.
REMEMBER TO ASK QUESTIONS TO ENCOURAGE OBSERVATION AND CRITICAL THINKING!
Hold the paper out at arm’s length and release!
- What happens?
- What do you notice about the paper moving through the air?
- Does it drop quickly or slowly?
- Does it float around a bit or drop straight down?
These are all good points to record in your journal if you are extending the learning portion of this air resistance STEM activity.
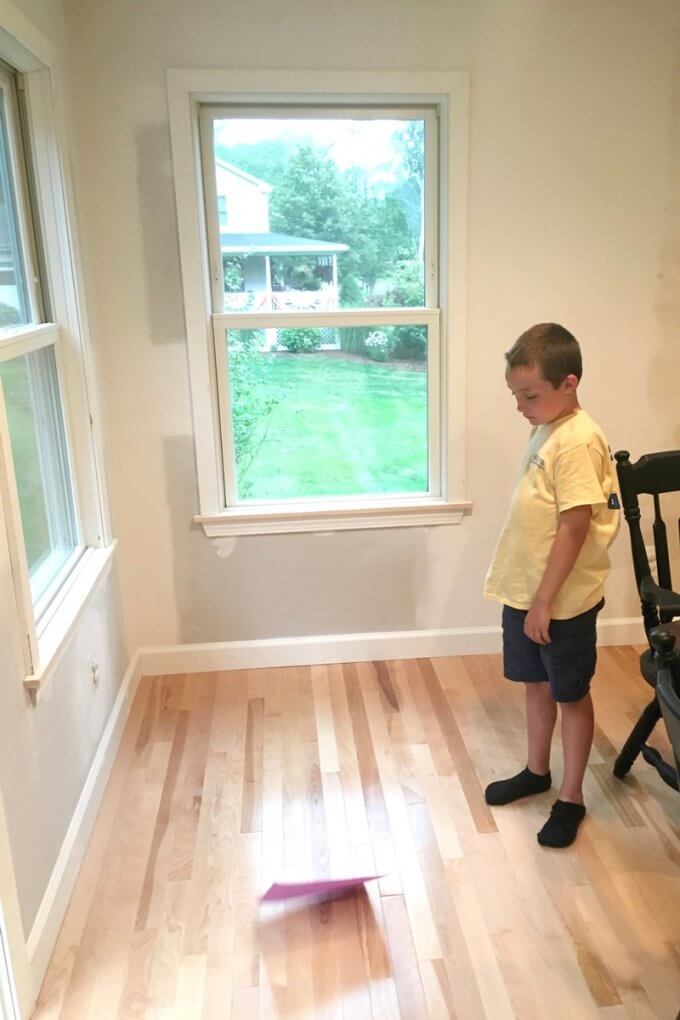
PART 2: Let’s test and compare the air resistance of different paper types. Cardboard, poster board, card stock… big and small pieces.
How to Make Air Foils
Luckily this is so simple as I remember some of the crazy origami folds I used to try and make from instructions!
We made three paper air foils, all with varying amounts of folds. 1/4 way up the paper, 1/2 way up the paper, and 3/4 way up the paper. Why do we need to test different amounts of folds? Doing so is called changing a variable in an experiment .
FOLD IT: Check out the 1/2 way up air foil below. While it might look like a traditional paper fan fold, a valley fold is not how you would fold a paper fan.
TIP: You are not flipping back and forth but instead folding the paper over itself until you get to the 1/2 way point or whatever point you choose to test.
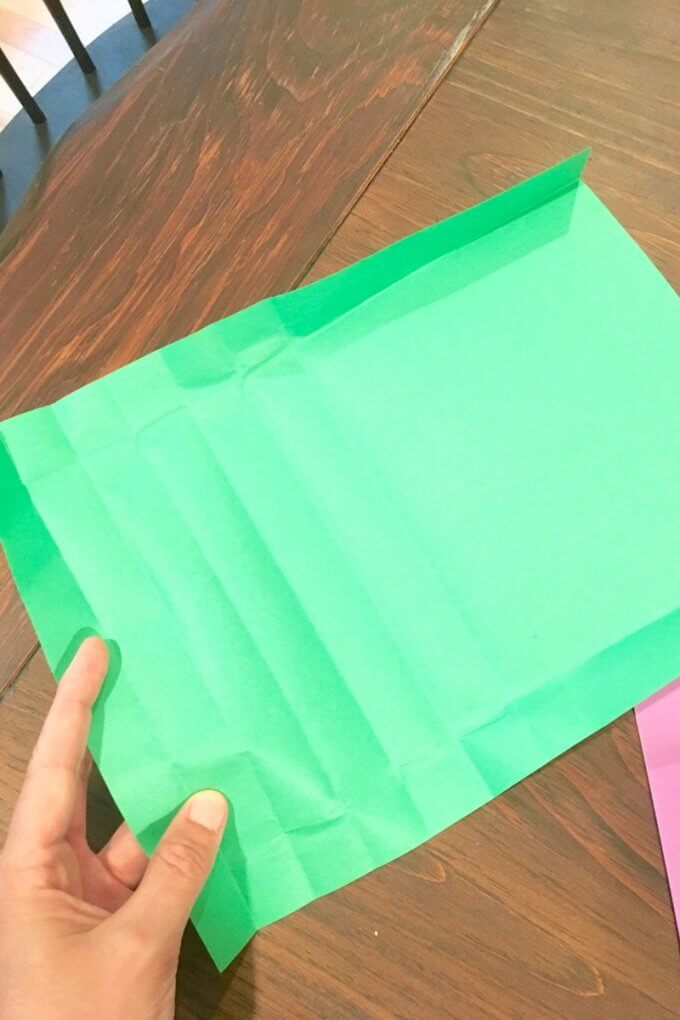
Finally, The last step for creating your paper air foil is to fold the edges over once on each side, as seen below. Nothing fancy. Just a quick and straightforward air foil with computer paper!
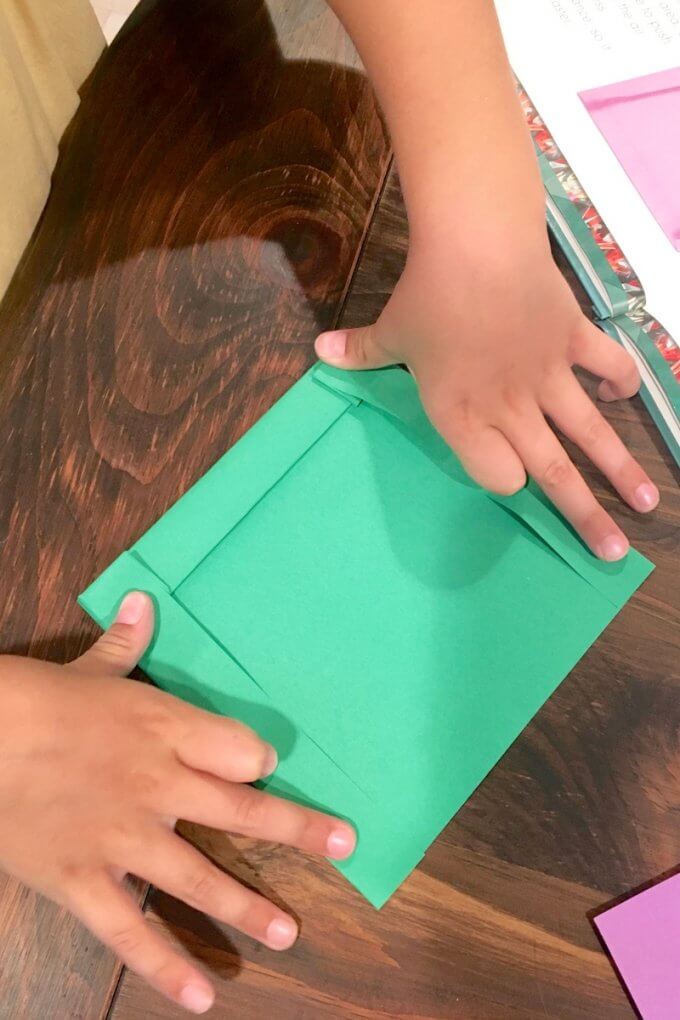
To test our thoughts on air resistance, we need to change the shape of the paper, and we will do it with an origami fold called the valley fold .
By now, you may have developed your hypothesis , which might be: Do different shapes of paper have different air resistance?
TEST IT: Now it’s time to test out what you know about air resistance. Take your control air foil (the unfolded paper) and test it with the newly folded air foil. Hold both your air foil models out at arm’s length and release.
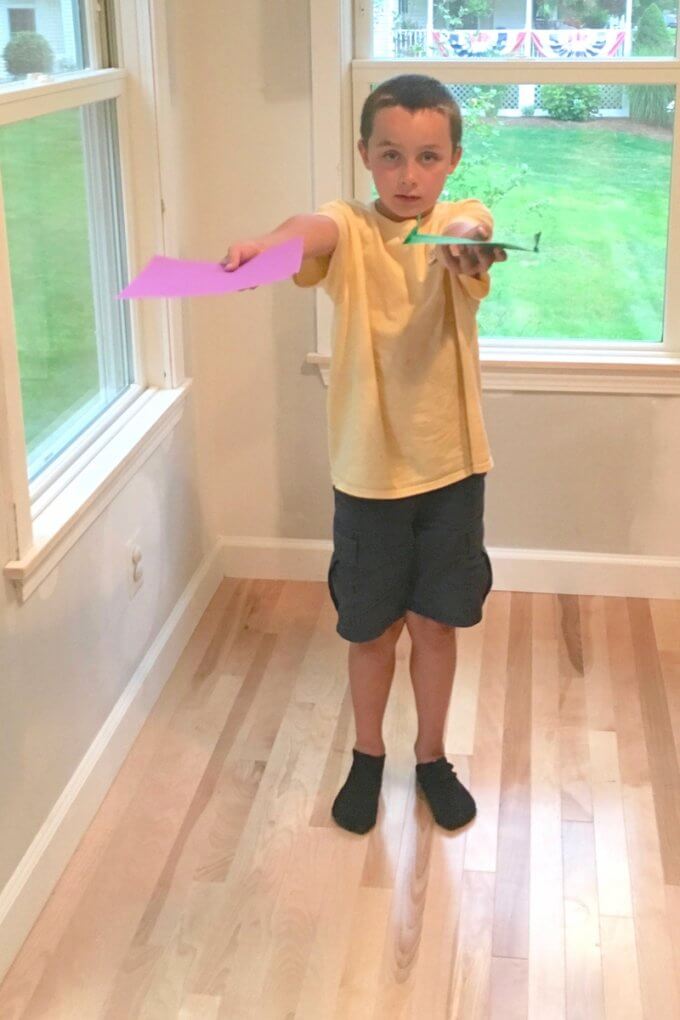
It’s time to take a look at what happens! What observations can you make, and are there any conclusions you can draw?
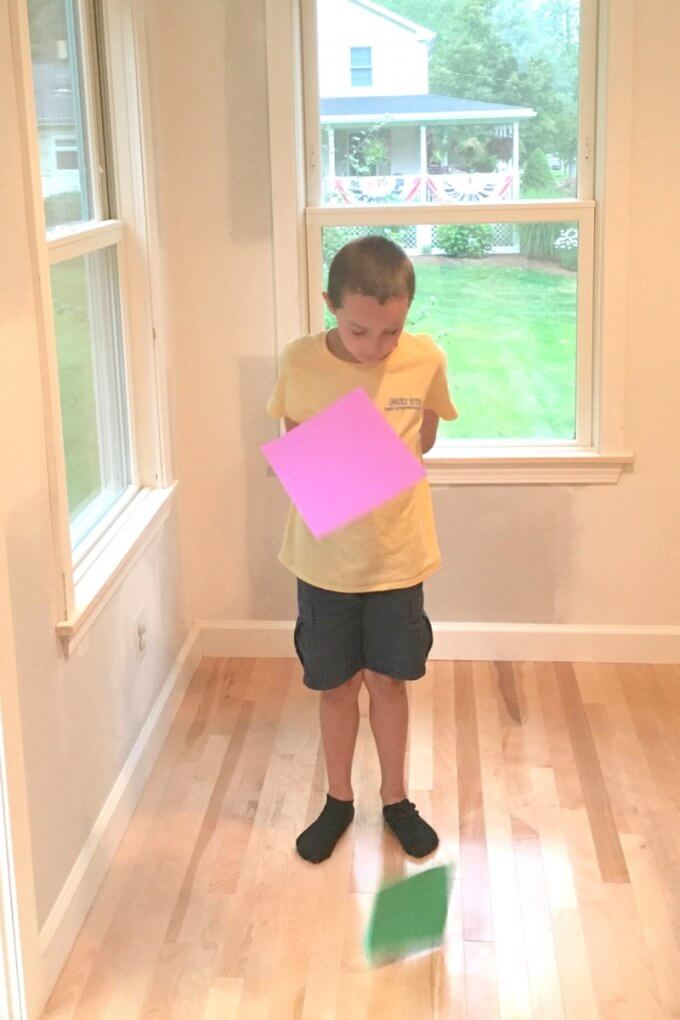
Then, we made an even smaller air foil by valley-folding the paper more! The difference is less noticeable. However, the more compact an air foil is, it does hit the ground faster. What other shapes of airfoils can you come up with?
In addition, you can test out different paper airplanes , scrunched up paper balls, or make a helicopter .
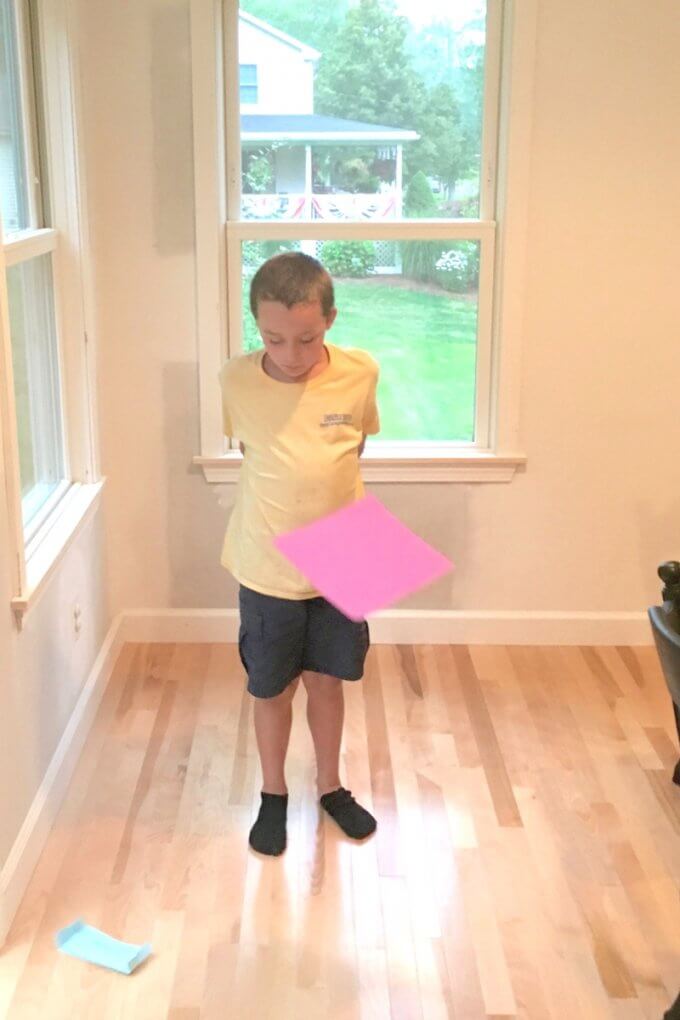
Observation skills, critical thinking skills, as well as the ability to persist through failure are all great lessons learned from simple STEM activities .
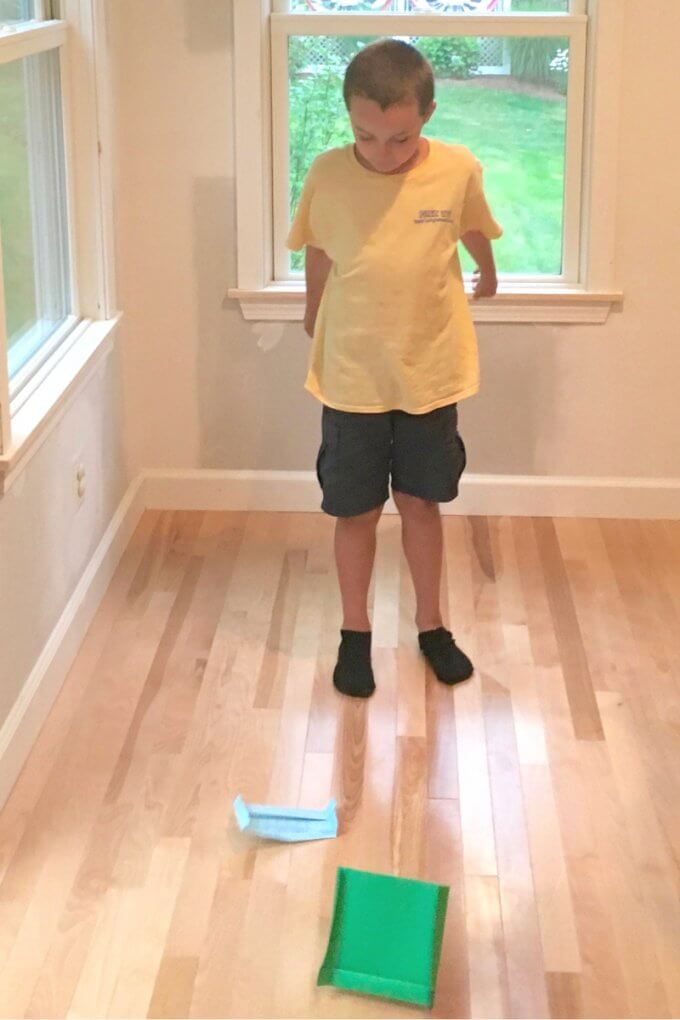
More Are Resistance Activity Ideas
Straw Rockets: Create simple rockets using paper and attach them to plastic straws. Kids can blow into the straw to launch the rockets and observe how air resistance affects their flight. Use the video to see how to create your own. Grab the templates for all the activities and many more in our Paper Projects Pack .
Watch the Video Here:
Parachute Drop: Design and make small parachutes from lightweight materials like tissue paper or plastic bags. Drop them from a height and observe how air resistance slows their descent.
Paper Airplane Race : Have a paper airplane competition to see whose plane flies the farthest or stays in the air the longest. Kids can experiment with different designs to understand how air resistance affects flight.
Wind Tube Experiments: Build a wind tube using cardboard boxes or PVC pipes, and let kids experiment with various objects to see how air resistance affects their movement in the tube.
Sailboat Racing: Make DIY sailboats out of everyday materials you have around. Craft paper sails with straws. Fill a shallow pool or tub with water and observe how the boats move using their sails.
Feather and Tissue Paper Races: Set up a race between a feather and a piece of tissue paper by blowing on them and observing how air resistance affects their motion. A turkey or meat baster is fun to use too!
Balloon Cars: Create simple balloon-powered cars using straws, bottle caps, and balloons. Kids can experiment with different balloon sizes and shapes to see how air resistance affects the car’s speed. In addition, you can set up balloon rockets!
Flying Kites: Make a DIY kite! Fly kites on a windy day and discuss with kids how the shape and design of the kite help it overcome air resistance.
Windmill STEM Project : Build a windmill and test it outside. Learn about how useful wind power can be and how it is generated.
Anemometer : Build an anemometer and take it outside to explore wind speed.
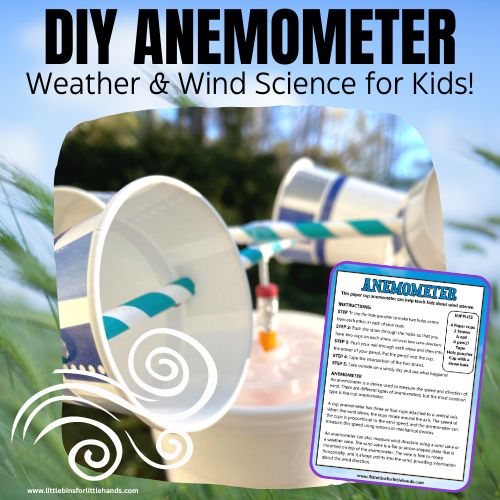
More Engineering Resources
Below you’ll find various engineering resources to supplement the many engineering projects on the website. From the design process to fun books to key vocabulary terms…you can feel confident providing these valuable skills. Each one of the resources below has a free printable!
ENGINEERING DESIGN PROCESS
Engineers often follow a design process. Although there are many different design processes that all engineers use, each one includes the same basic steps to identify and solve problems.
An example of the process is “ask, imagine, plan, create, and improve.” This process is flexible and may be completed in any order. Learn more about the Engineering Design Process .
WHAT IS AN ENGINEER?
Is a scientist an engineer? Is an engineer a scientist? It might not be very clear! Often scientists and engineers work together to solve a problem. You may find it hard to understand how they are similar yet different. Learn more about what an engineer is .
ENGINEERING BOOKS FOR KIDS
Sometimes the best way to introduce STEM is through a colorfully illustrated book with characters your kids can relate to! Check out this fantastic list of teacher-approved engineering books , and get ready to spark curiosity and exploration!
ENGINEERING VOCAB
Think like an engineer! Talk like an engineer! Act like an engineer! Get kids started with a vocabulary list that introduces some awesome engineering terms . Make sure to include them in your next engineering challenge or project.
QUESTIONS FOR REFLECTION
Use these reflection questions below with your kids after they have completed a STEM challenge. These questions will encourage discussion of the results and increase critical thinking skills. These questions or prompts will help to promote meaningful discussions individually and in groups. Read the questions for reflection here.
Printable STEM Pack for Kids
80+ Doable Engineering Projects in one convenient pack!
- Full instructions with sample images
- Activity-specific instruction sheets
- Data Collection Sheets
- Questions for Reflection
- Architecture Building Cards: Try the tallest tower challenge
- Bridge Building Cards: Explore different types of bridges to build your own.
- Paper Chain STEM Challenge: Who can make the longest chain? Great icebreaker or quick challenge!
- 3 Little Pigs Architectural Pack: Design a house that won’t blow away!
- Great marshmallow challenge: A classic challenge kids love!
- Want to try a Real-world STEM challenge lesson but don’t know where to start? Our easy-to-follow template shows the steps!
- What’s the difference between a scientist and an engineer?
- Crossword and word search with engineering vocabulary.
- Engineering vocabulary cards
- Design a one-of-a-kind invention and write about it with this 5-page activity!
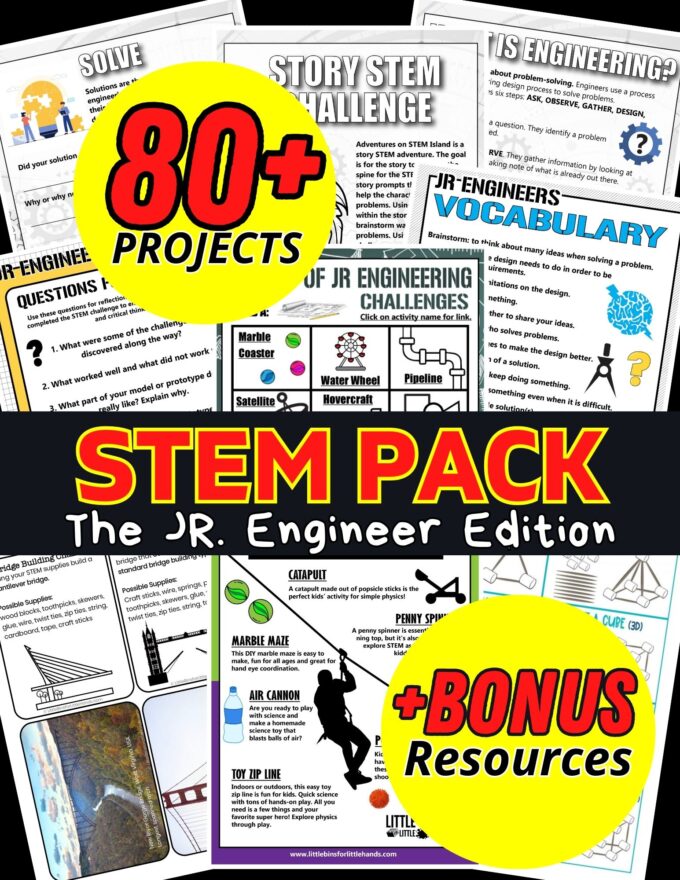
- Pingback: 21 Quick STEM Activities for When You're In a Rush
- Pingback: Simple Physics Activities Science Experiments STEM Ideas for Kids
Comments are closed.

Subscribe to receive a free 5-Day STEM Challenge Guide
~ projects to try now ~.


Fun and easy play-based STEAM activity for toddlers, preschool & kindergarten
5 fun play-based science activity ideas for kids – air and more.
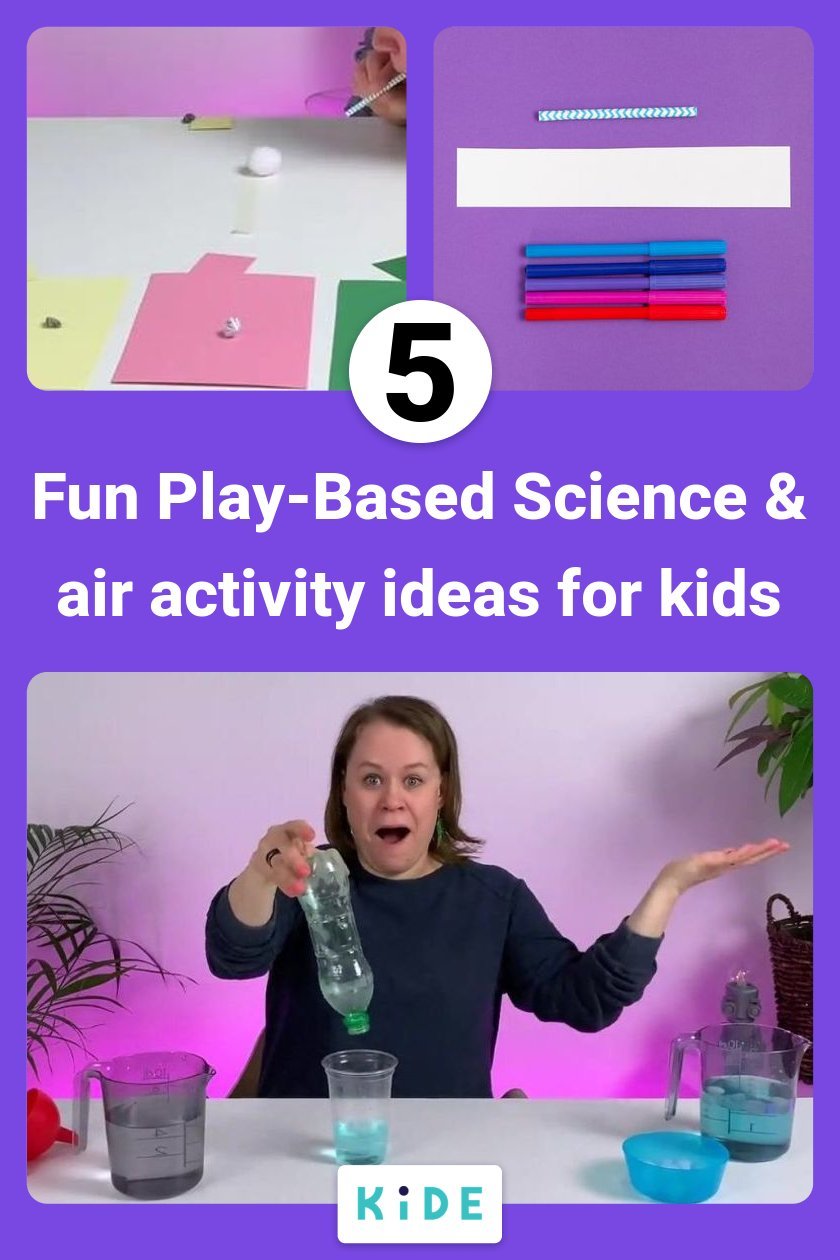
These hands-on activities only need everyday supplies and minimal preparation.
They're perfect for toddlers, preschoolers, and kindergarteners and can be easily adapted to meet popular learning standards and curriculums.
Make learning fun by introducing research problems through play and a story. This will motivate children to solve problems for their new imaginary friends!
According to academic research, stories lead to better focus, increased engagement, and improved learning outcomes.
5 best air science activity ideas
1. power of the wind, 2. the windworms, 3. shrinking air, 4. wind in a bottle, 5. hot and cold bottles, how can air move things around.
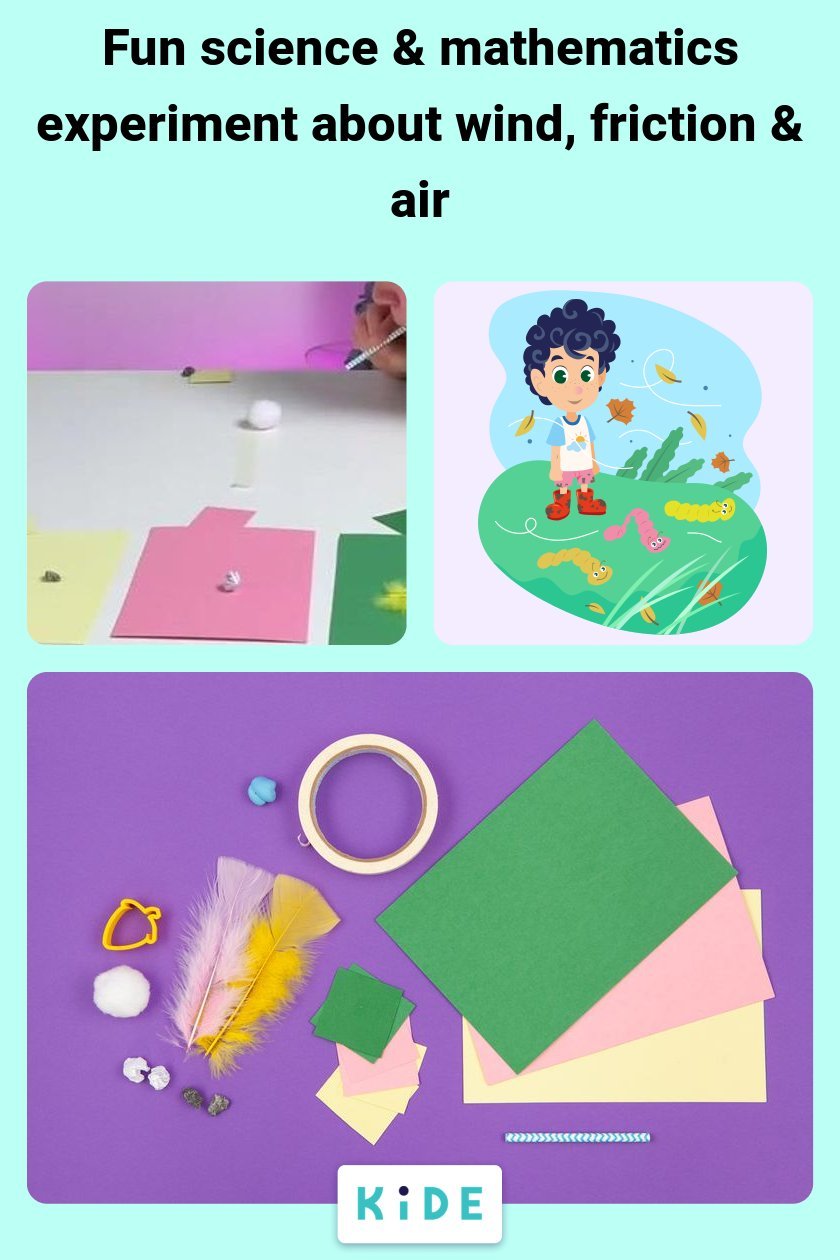
Measure how far the blown air can move different objects.
Practise making a prediction by pondering how far the air will be able to move the objects.
masking tape
different-coloured cardstock to use as classification bases
small pieces of coloured paper to mark your measurements
different small objects
You can also use
poster putty
See the full activity Or, subscribe for free weekly activities
Free weekly activities of your choice. No hidden fees, no credit card needed.
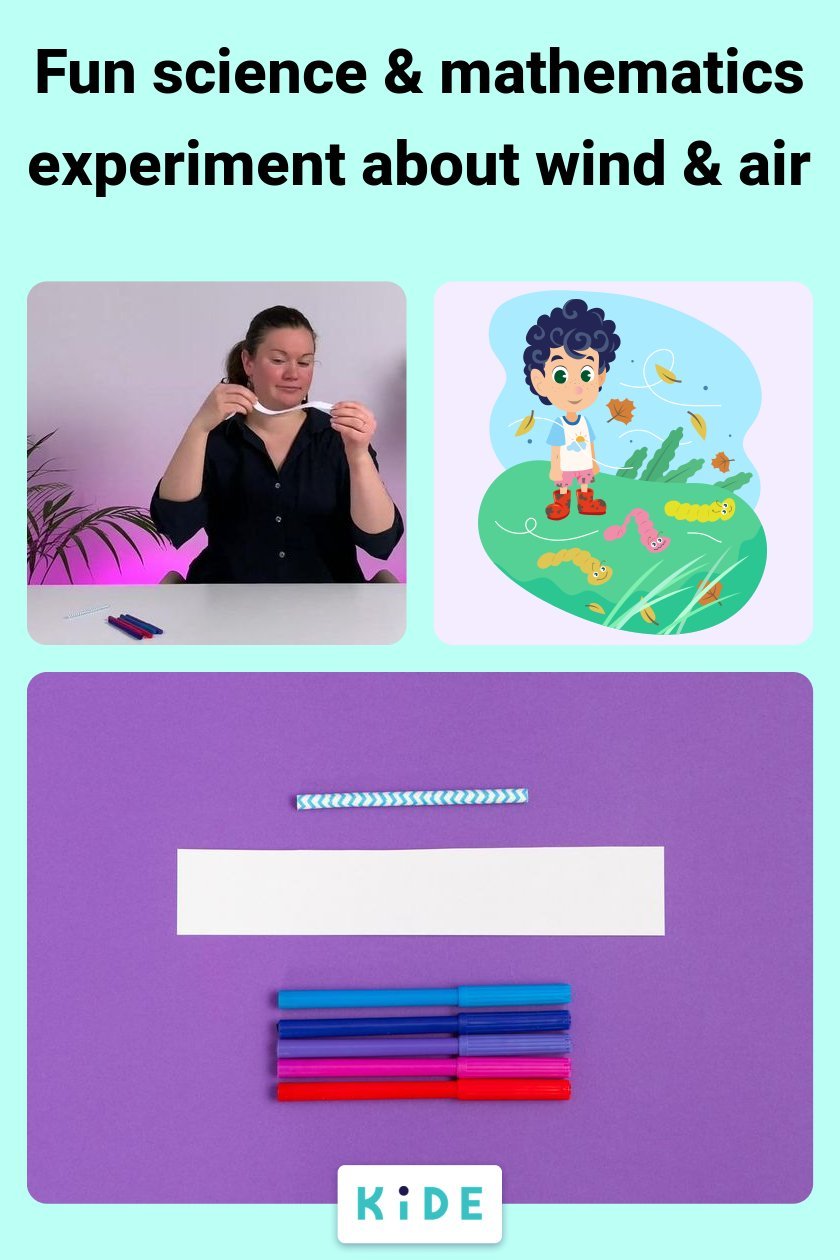
Continue making observations about how the wind moves objects.
Craft your own windworms and have a playful race with them
strips of paper
coloured pencils
instructions
Can air get hot and cold?
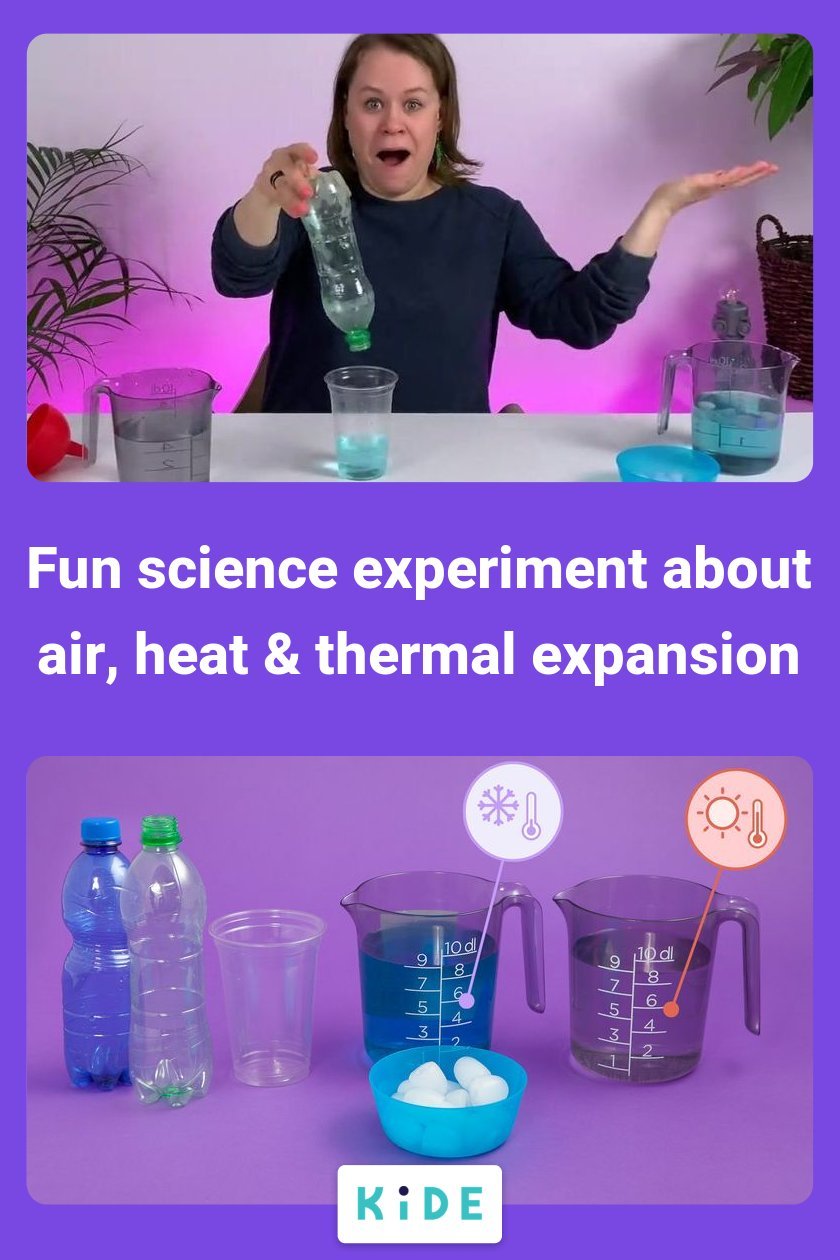
Observe how air takes up space
warm tap water in a jug/thermos bottle
cold tap water in jugs
tall, transparent cups
plastic bottles
food colouring
Supplies for Demonstration
cold water in a dish/jug
hot water in a jug/thermos bottle
plastic bottle with a cap
You Can Also Use
thermometers
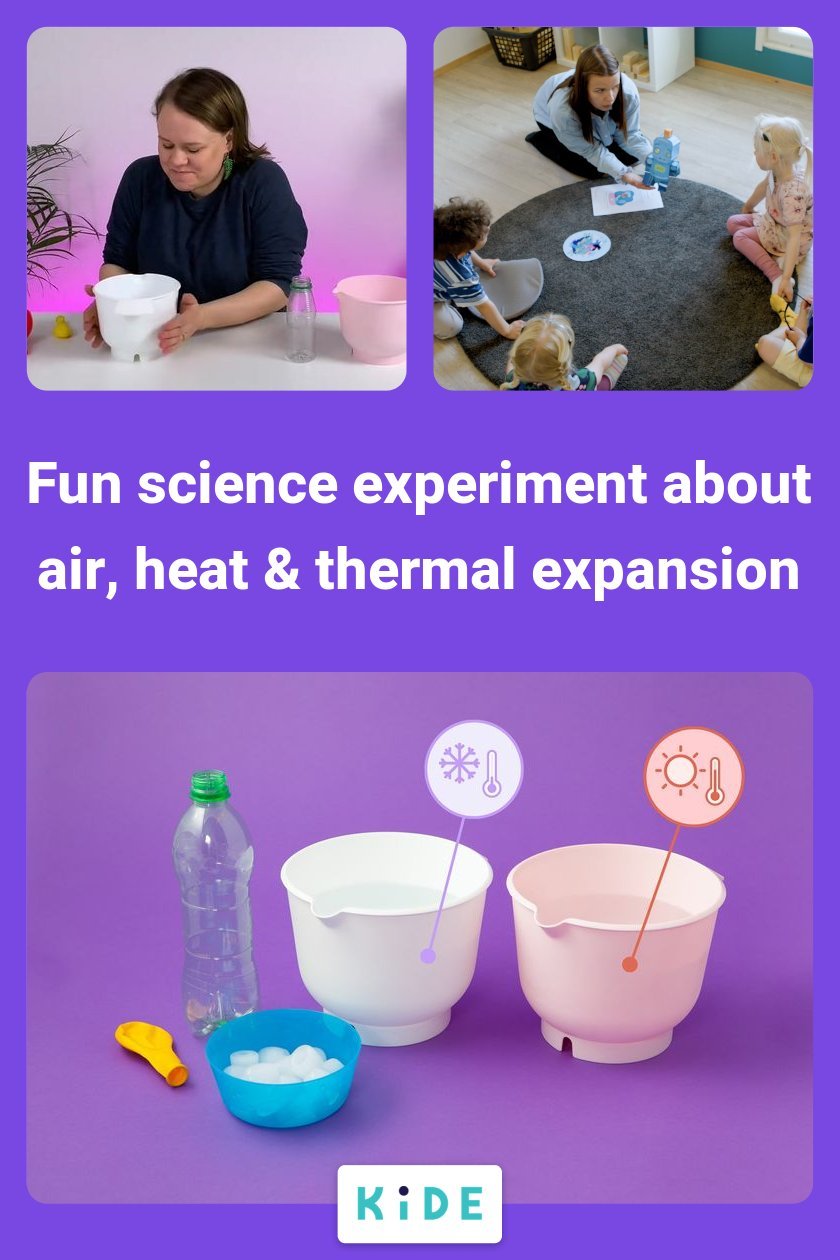
Observe the thermal expansion in a bottle
Make interpretations: heated air takes up more space than cold air.
cold water in dishes
warm water in dishe
hot water in a thermos bottle

What happens to a plastic bottle when the temperature changes?
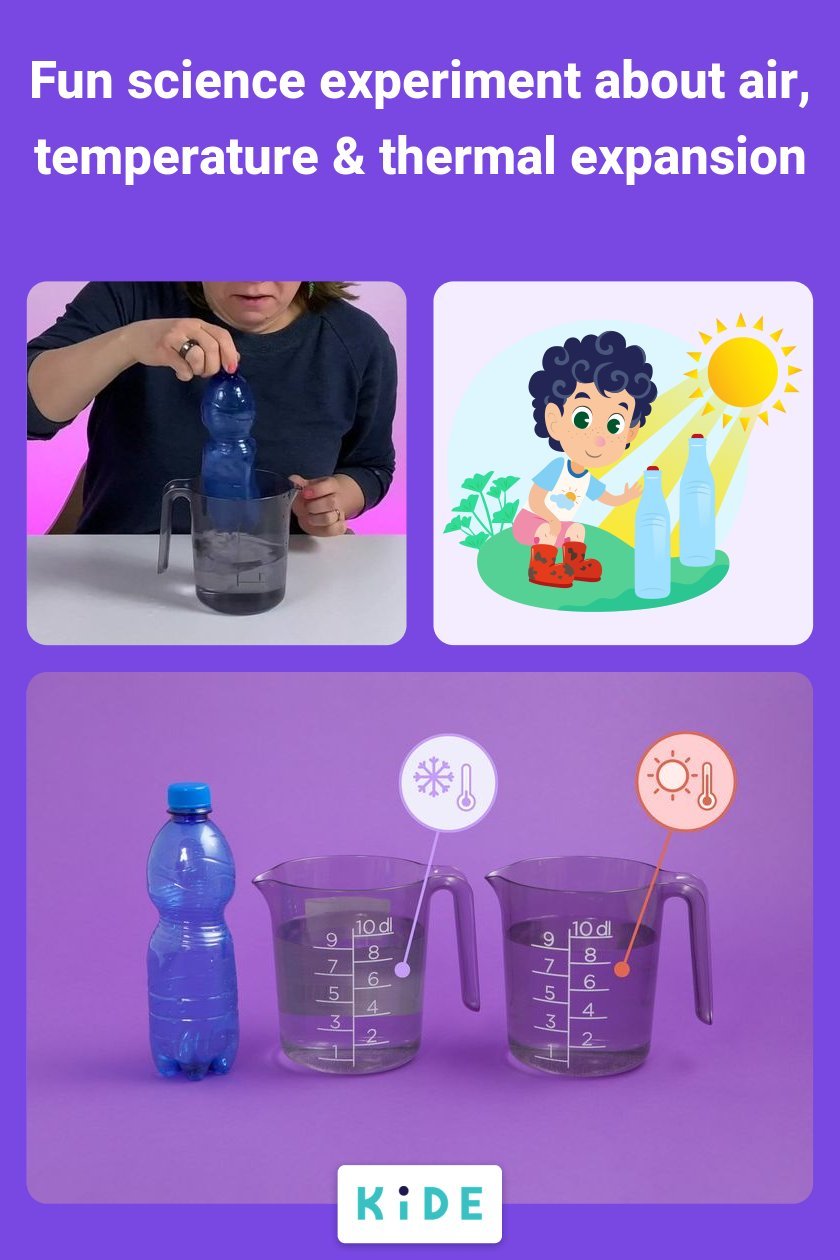
Observe how the bottle changes with thermal expansion
Interpret how air takes up space
jugs / bowls of cold water
jugs / bowls of hot water
plastic bottles with caps
cooler bag for the ice
What is Kide Science – and is it for free?
Kide Science is a free digital treasure trove of ready-to-go, play-based lesson plans and training materials for teachers of 3-8 year olds.
With our free subscription, you get free weekly lesson plans like these directly to your inbox.
Subscribe here for free weekly activities!
What others love about Kide’s activities
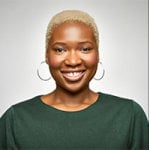
Preschool Teacher
This program is incredible. The characters, the stories, the experiments are so much fun. I do not need to spend any time planning. Everything I need is given to me be Kide Science.
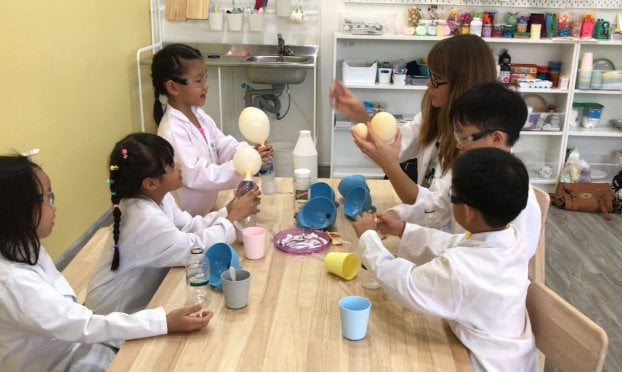
Kindergarten Teacher
Super easy to plan, and the items are usually things that we already have. Planning is made very easy & the children are very motivated!
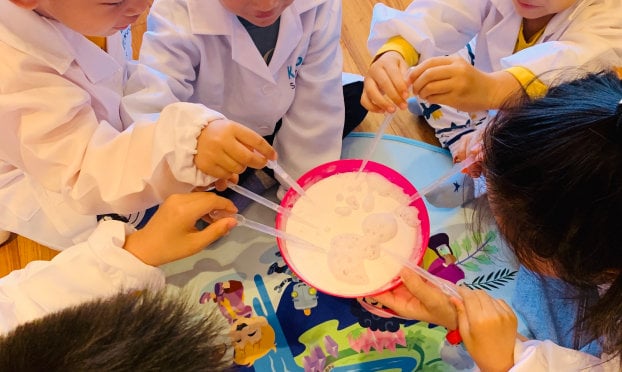
Was just observed doing one of these lessons. Principal was shocked and so was I - one of the kids with pretty severe attention issues was engaged the entire time!
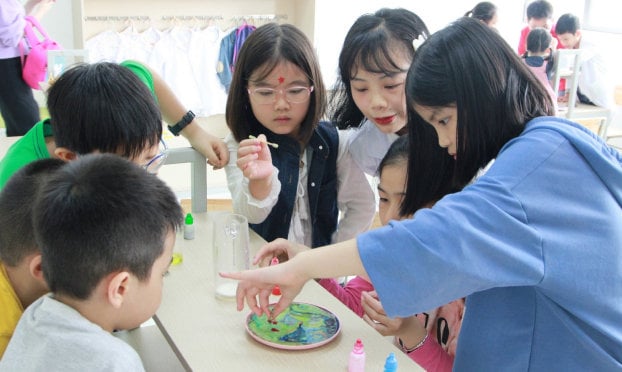
Trusted by brands, loved by over 100,000 children globally
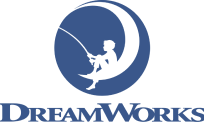
100s of free activities are waiting for you
Recommended based on your interests:
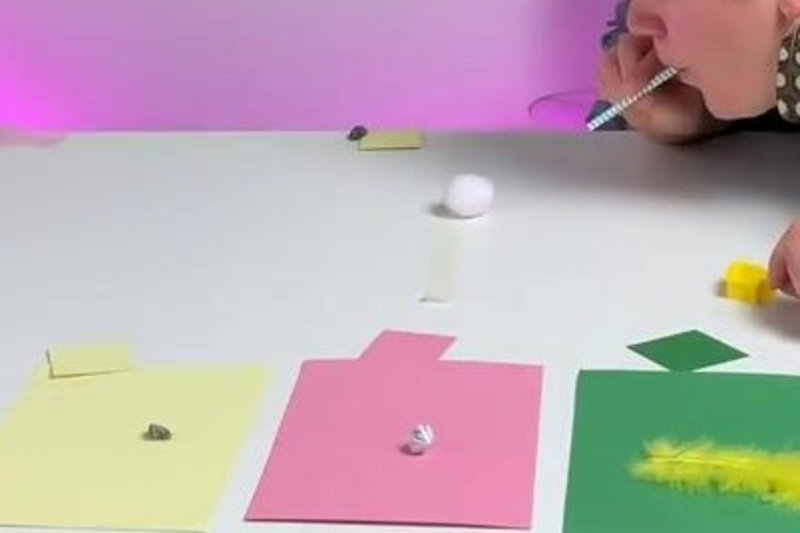
Force of the Wind
Science Mathematics
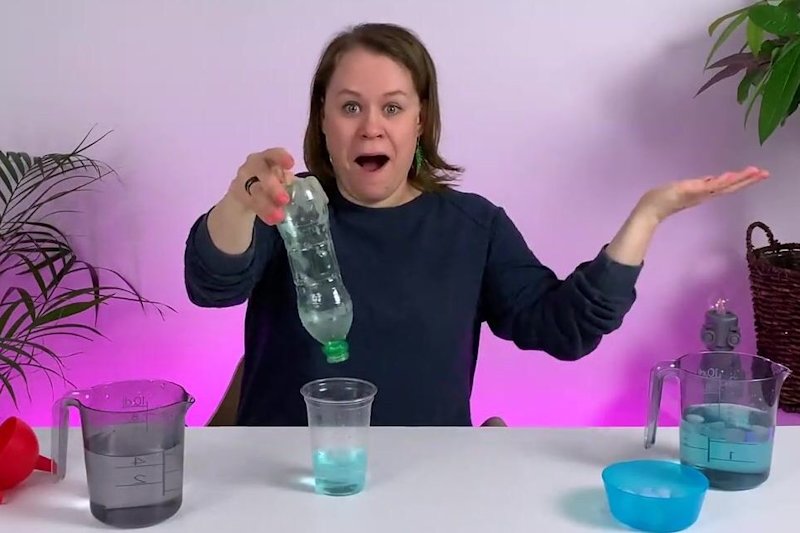
Bottled Heat
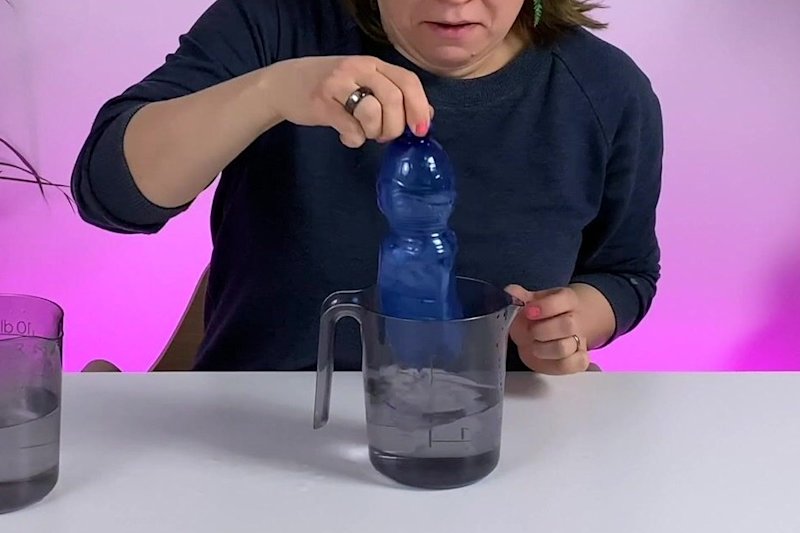
Hot and Cold Bottles
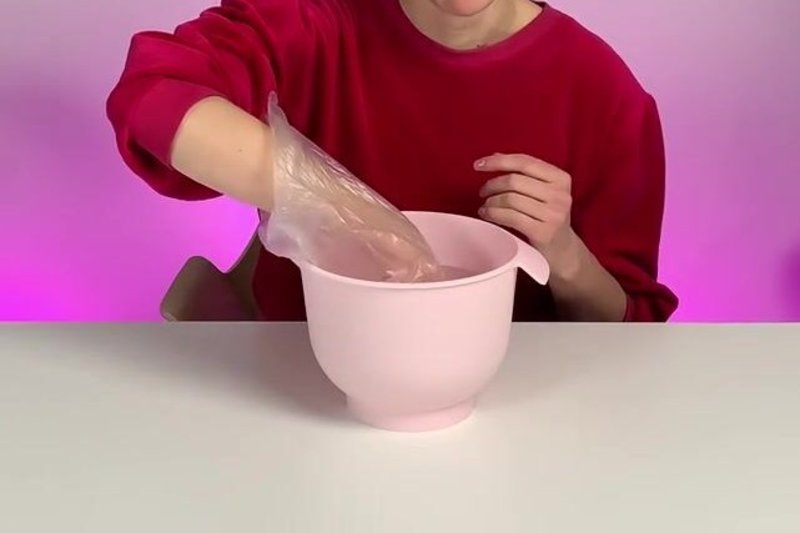
Pressure in the Puddle
Can water feel tight?
Ready to get started and create the best learning experience for your children.
Get lesson plans
It is free and no credit card needed! With the free subscription, you get 1 free lesson per week. See our pricing to get unlimited access to all lesson plans and training materials. Learn more about subscription plans
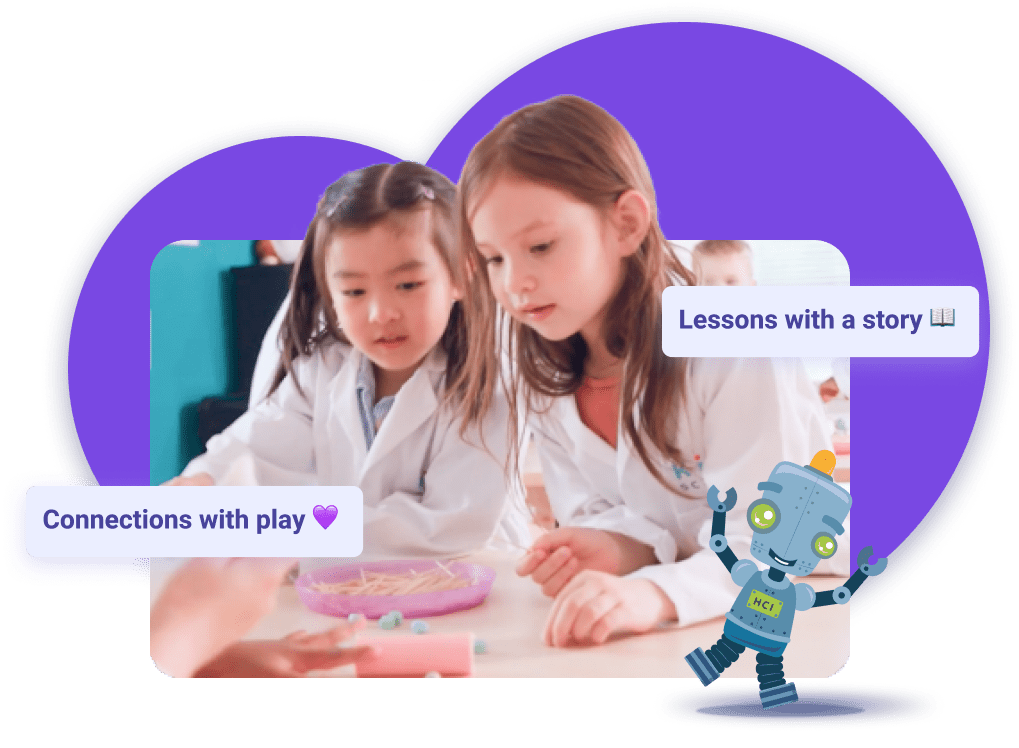
Copying/printing is not contractually allowed due to copyright reasons.
You can find printable materials in our lesson plan attachments and extras section.
Check out our Easy Learn to Draw lessons →
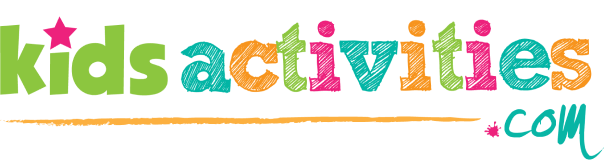
Home › Blog › Kids Activities › Learning Activities › 2 Easy Hands-On Air Pressure Science Experiments for Kids
2 Easy Hands-On Air Pressure Science Experiments for Kids

Published Dec 01, 2023
Updated Jun 15, 2024
Let’s explore air pressure with kids with these fun and easy science experiments that work for kids of all ages, even toddlers and preschoolers. Here are two air pressure experiments that are super simple, use items from around the house and are a great way to play with science at home or in the classroom.
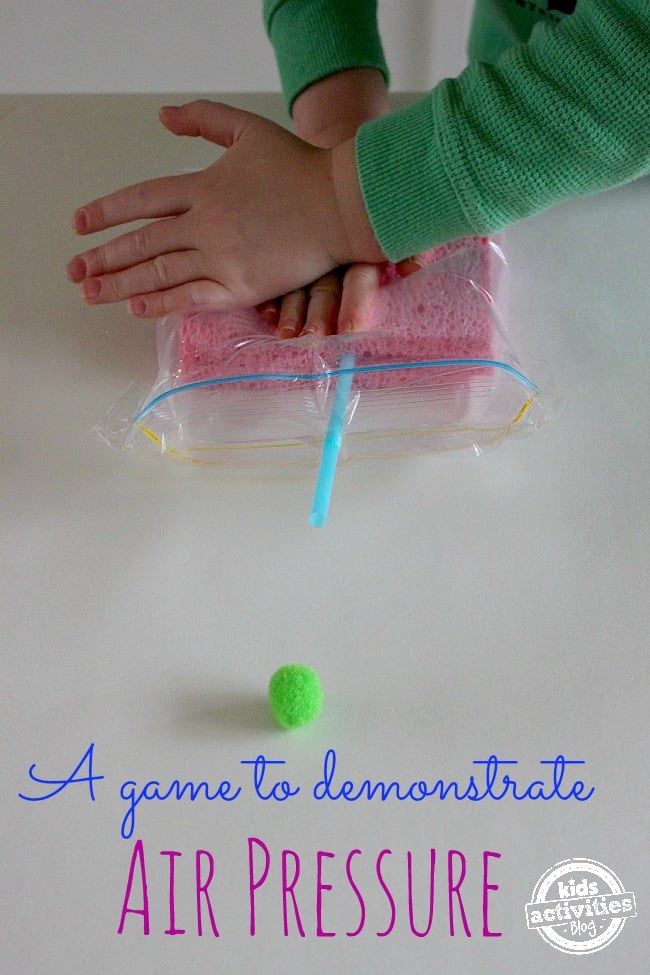
Explaining the concept of air pressure to preschoolers can be tricky. It can sometimes be difficult for them to grasp the idea that spaces are filled with air, and that air can have force and move objects.
Related: Simple Machines for Kids
Air pressure is the weight of air molecules pressing down on the Earth. The pressure of the air molecules changes as you move upward from sea level into the atmosphere. The highest pressure is at sea level where the density of the air molecules is the greatest. #savethearticle form.seva-form.formkit-form .formkit-field:nth-child(2), #savethearticle form.seva-form.formkit-form .formkit-field:nth-child(3) { display: none; } .formkit-form { background: none !important; border: 4px solid #06B5A5 !important; } .formkit-form [data-style="minimal"]{ padding: 20px !important; } .formkit-form .formkit-header h2, .formkit-form p { text-align: left; } .formkit-form .formkit-header { margin-bottom: 0 !important; } .formkit-form .formkit-header h2 { font-size: 32px !important; margin: 10px 0 !important; color: #E21872 !Important; } .formkit-form .formkit-subheader { margin-top: 0 !important; } .formkit-form .formkit-subheader p { font-size: 16px !important } .formkit-form .seva-fields.formkit-fields .formkit-field { flex: 70% !important; margin-right: 10px !important; width: 70%; } .formkit-form .seva-fields.formkit-fields button { flex: 20% !important; width: 20%; flex: 11% !important; background-color: #8BC63F !important; text-decoration: none; color: #fff !important; height: 46px; } .formkit-form .seva-fields.formkit-fields button > * { transition: unset !important } .formkit-form .seva-fields.formkit-fields button:hover { background-color: #F6941D !important; color: #fff !important; } .formkit-form .formkit-guarantee { display: none } .formkit-form .formkit-alert-success { background: none !important; border-color: transparent !important; color: #000 !important; } @media (max-width: 580px){ .formkit-form .seva-fields.formkit-fields button { flex: 25% !important; } .formkit-form .seva-fields.formkit-fields { display: grid !important } .formkit-form .seva-fields.formkit-fields .formkit-field { flex: 100% !important; width: 100%; margin-right: 0 !important; min-width: auto; } .formkit-form .seva-fields.formkit-fields button { width: 100%; flex: 100% !important; min-width: auto; } } jQuery(document).ready(function(){ setTimeout(() => { let _title = jQuery('.single .entry-title:first').text(); let _url = window.location.href; jQuery('#savethearticle form.seva-form.formkit-form .formkit-field:nth-child(2) input').attr('value', _title); jQuery('#savethearticle form.seva-form.formkit-form .formkit-field:nth-child(3) input').attr('value', _url); }, 1000) }); – Kids Fun Science , What is Air Pressure
Air Pressure Experiments for Kids
These simple air pressure experiments are great way to offer kids a visual demonstration of these concepts. They can feel the air on their skin and see it moving objects. It is also a lot of fun!
Air Pressure Experiment 1: Force and Pressure Experiment
In the first of our air pressure experiments for kids we will be investigating force and pressure and how that relates to air pressure. It is an easy way to demonstrate air pressure. Using simple things from around the house like a sponge and straw, they will be able to physically propel an object with just air!
Air Pressure Experiment 2: Water Bottle Air Pressure Experiment
In the second of our air pressure experiments, kids will see how air pressure can be used in a water bottle in the most unexpected way. They will be able to control actions inside the water based on the air pressure. This experiment uses a soda bottle.
This article contains affiliate links.
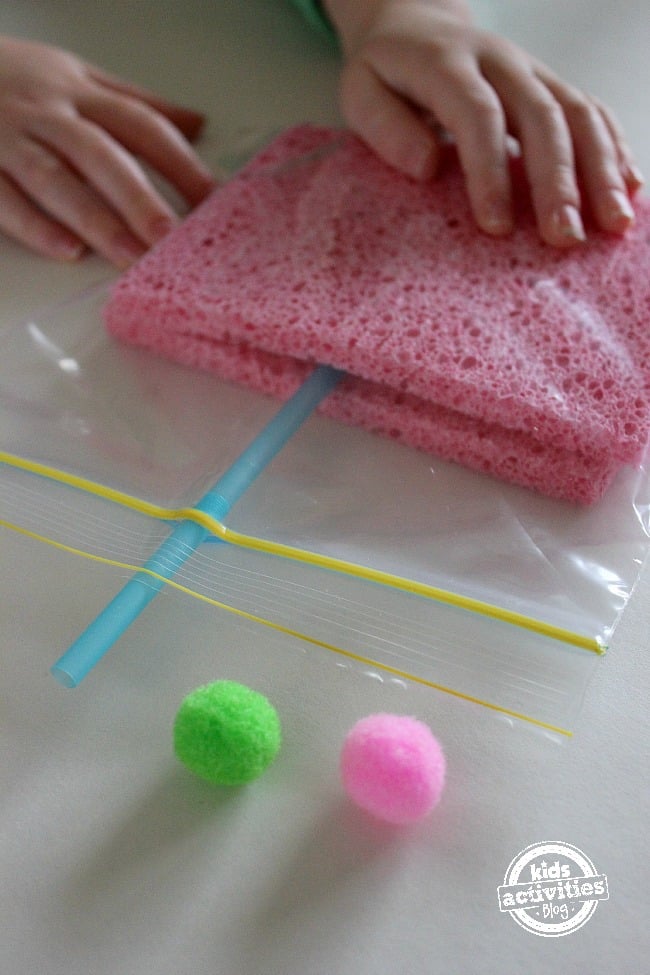
Force and Pressure Experiment
This air pressure activity is so simple to set up.
Supplies Needed
- 2 Kitchen sponges
- Drinking straw
- Plastic bag that zips like a Ziploc bag
- Pom poms , toy cars and anything else that they might want to move
Instructions for Simple Air Pressure Experiment
- Simply place the two kitchen sponges, one on top of the other, inside the plastic zip lock bag.
- Place the drinking straw between the two sponges so that one end of the straw is inside the bag and the other end is sitting outside the bag.
- Seal up the bag.
- In addition to closing the zip lock seal on the bag, you will also need to seal it with adhesive tape. The tape is not showing in the photo above. We first tested it out by just closing the zip seal but the bag burst open during the experiment. So, seal up your bag with adhesive tape and then you are ready to play!
- Blow into the straw to inflate the bag.
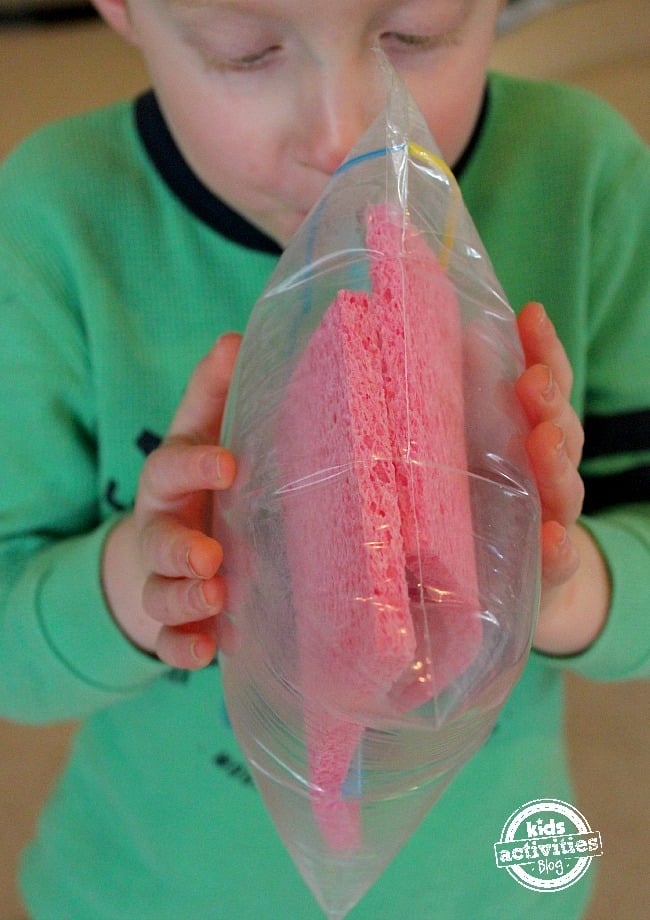
Ziploc Bag Experiment that Moves Items
6. Now place a pom pom on a flat surface and place the bag behind them so that the straw is positioned to blow the pom pom.
7. Press down hard on the sponges and watch the pom pom roll away!
Our Experience with this Science Activity
My son decided he wanted the pom pom to be moved with much greater momentum so he blew air into the straw, filling up the bag as much as he could. He then gave it another try and the pom pom really flew off the table this time around. He loved experimenting with low pressure and high pressure moments.
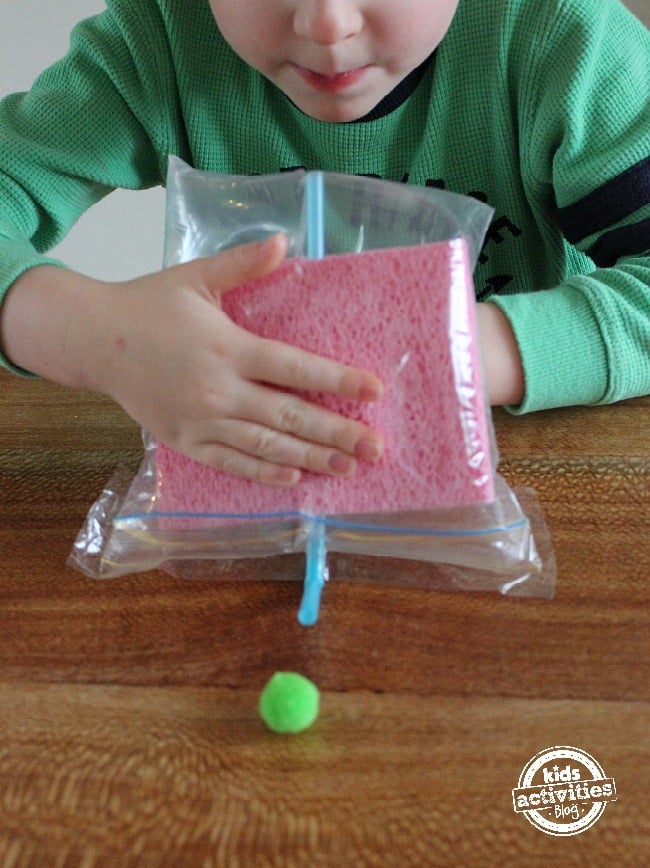
Car Ziploc Bag Experiment
Here are some simple ways to vary the air pressure activity for more hands-on science learning:
- Try holding the bag at different angles. How does this affect the pom pom?
- It would be fun to turn this into a game of air-soccer.
- Simply lay a container (eg a tin can, a paper cup or an empty tin can) on its side and try to shoot the pom pom into the goal.
- We also tried to move other objects such as toy cars and LEGO mini figures. Some of them moved and others didn’t.
- Our favorite was definitely the car ziploc bag combination! It was really cool to see a Hot Wheels car speed across the table only powered by air pressure.
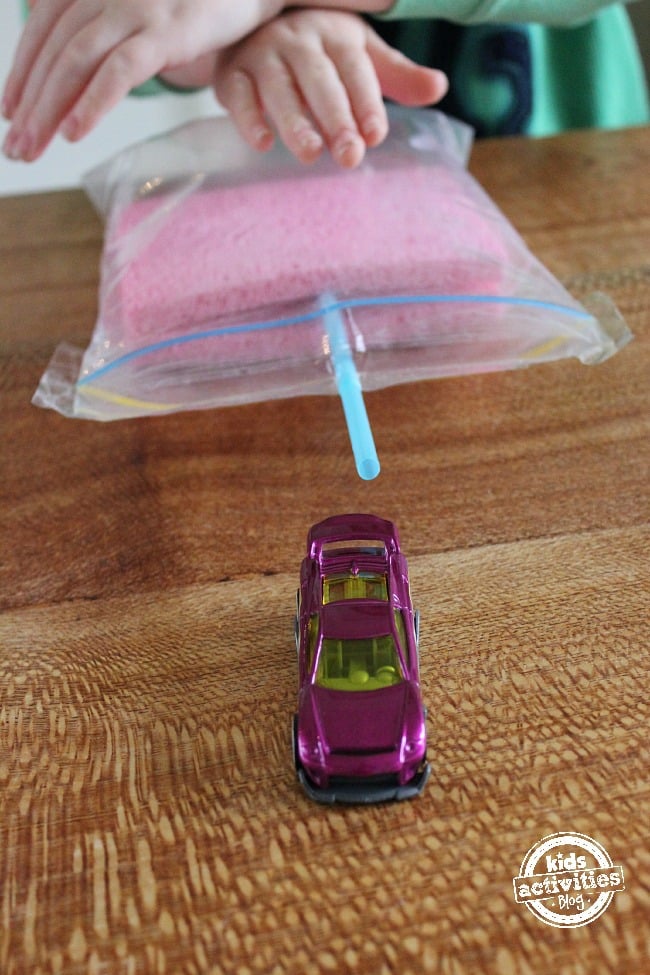
Air Pressure Experiment #1 Results
Try to make predictions about what you think will be moved and then test your theories.
Can you think why some objects are moved by the air and others aren’t?
Have kids record what happens. Younger kids like preschoolers can make drawings of their predictions and then results. Older kids can write out what they think will happen and then what did.
That was fun! Let’s go on to the next of our air pressure experiments…
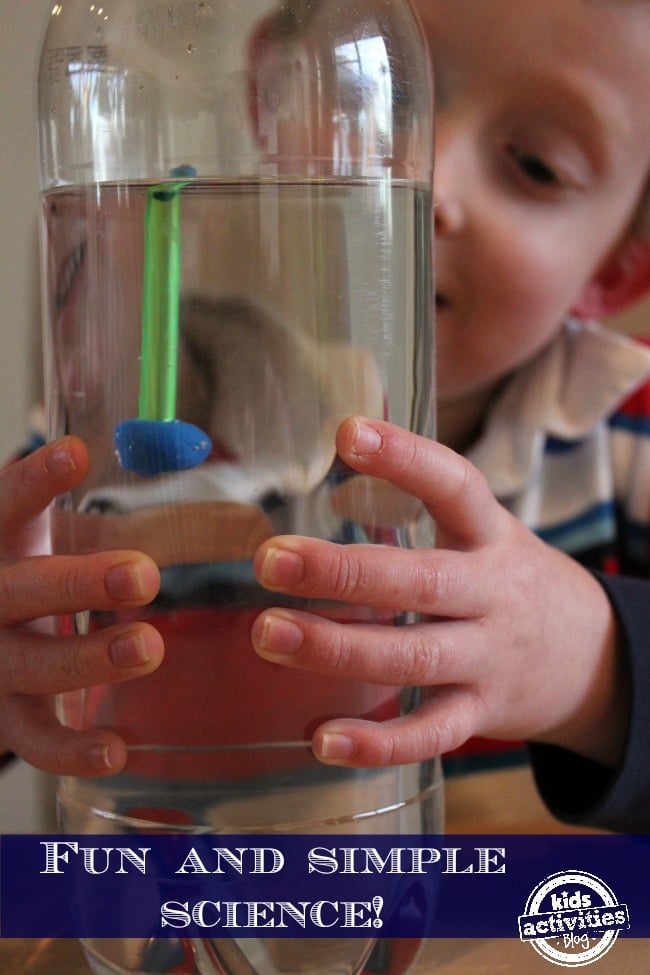
Water Bottle Air Pressure Experiment
Since air pressure is a big concept for preschoolers to understand, this fun and simple science experiment is a great hands-on demonstration of air pressure.
Kids love watching the straw dancing and bobbing around in the water bottle and it’s a great activity for strengthening hand muscles as they squeeze the bottle as hard as they can, to move the straw up and down.
You likely have everything you already need at the house.
- An empty soda or water bottle with the label removed (you need to be able to see inside!)
- A small amount of play dough, plasticine or clay
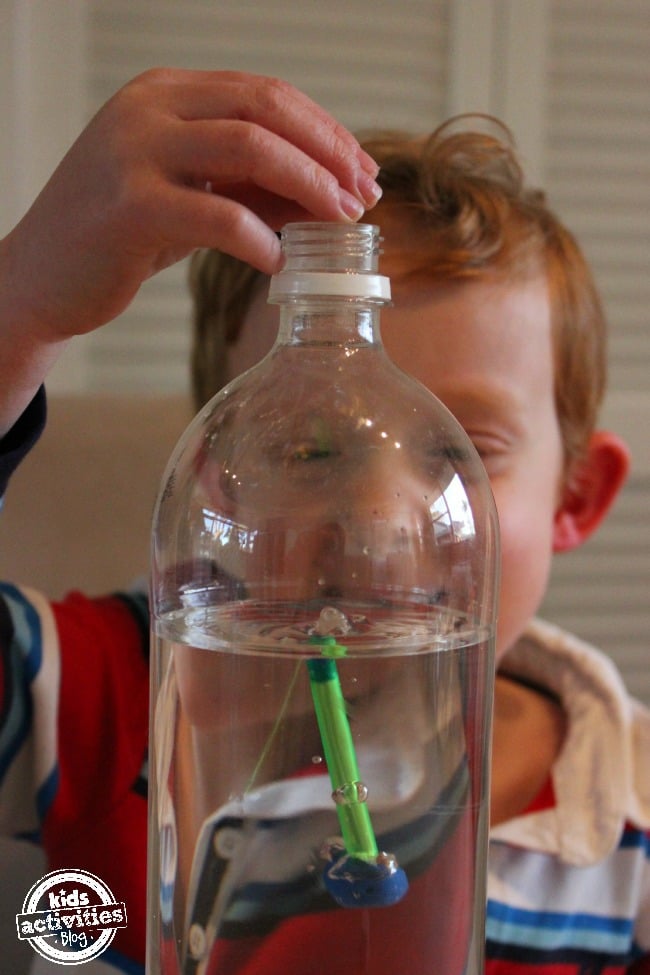
How To Demonstrate Air Pressure To Kids with a Water Bottle
This air pressure activity is easy to do. Here are the steps to the second of our air pressure experiments:
- Cut a small length of straw
- Plug one end of the straw with the play dough
- On the opposite end of the straw, make a ring of play dough around the outside of the straw (so the straw is weighted but is open at that end)
- Fill the bottle three quarters full with water
- Drop the straw into the mouth of the bottle and screw on the lid
- Now to the fun part…
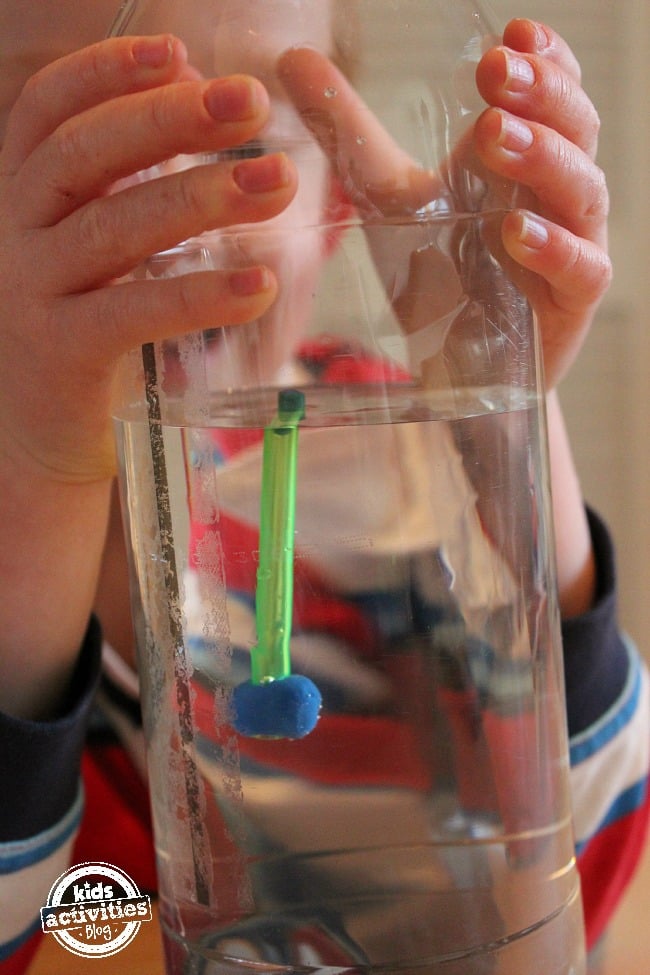
Squeeze the bottle as hard as you can and watch what happens……
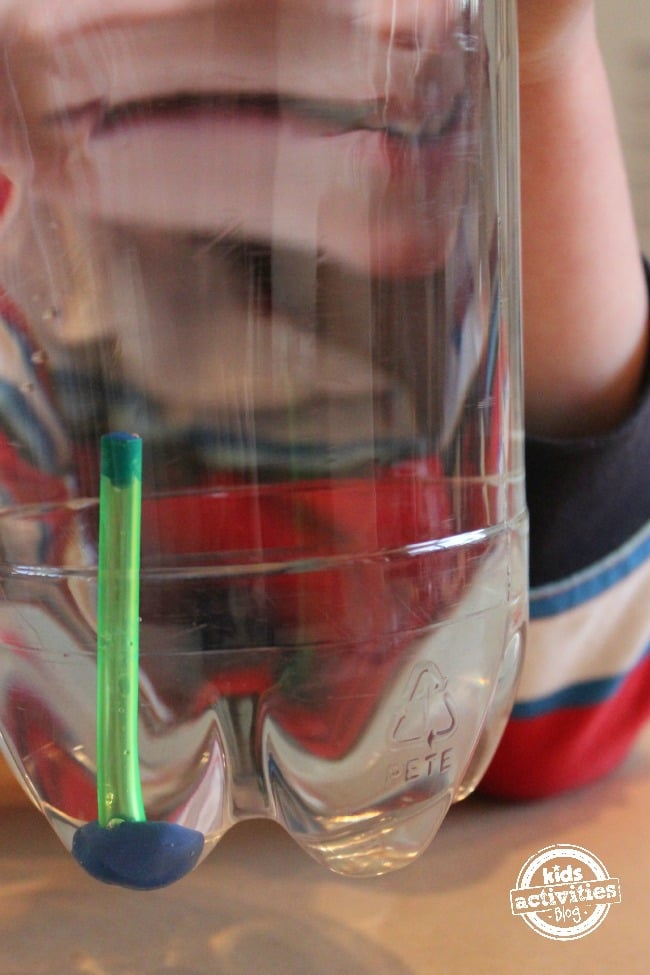
The straw will sink!
TIP : If your straw is not sinking try squeezing the bottle at the top where there is no water. Also try lifting the bottle off the table when you squeeze.
Air Pressure Experiment #2 Results
So what is air pressure and what’s actually happening in the bottle?
- When you drop the straw into the bottle, the air inside it makes it float. When you squeeze the bottle, the space inside is compressed, that is, there is less space for the air to circulate so the pressure inside the bottle increases.
- The increased air pressure pushes water up into the straw, making it heavier, so it sinks.
- When you release the pressure on the bottle, the air pressure decreases (ie the air has more space to move around) so the air fills the straw again and the straw floats back to the top of the bottle.
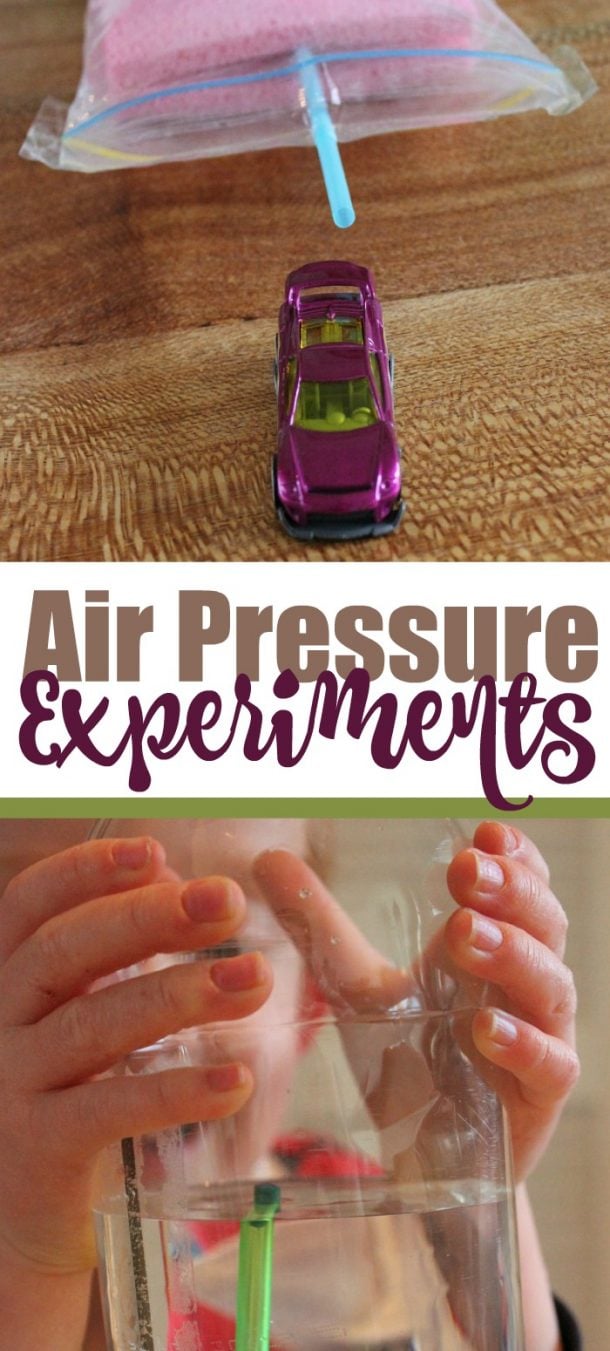
Air Pressure Experiments Questions Answered…
1. what is an example of air pressure.
Anytime force is exerted onto a surface by air is an example of air pressure. One that we see everyday (and may not even think about it) is the tires on our car. The car tires are filled with air that cannot escape. The weight of the car pushes on the air in the tires and the air “pushes” back.
2. What is the principle of air pressure?
Bernoulli’s principle stats that an increase in the speed of a fluid occurs simultaneously with a decrease in pressure or a decrease in the fluid’s potential energy.
Air is fluid. It flows and can change shape.
3. What is the earth’s atmospheric pressure?
The standard, or near-average, atmospheric pressure at sea level on the Earth is 1013 millibars, or 760 millimeters of mercury. I liked the way this article at National Geographic explained it.
4. Is humidity related to air pressure?
Yes because air that is humid is much lighter and has less weight to press down resulting in lower pressure.
5. What does air pressure mean for kids?
Even though it might not feel like it, air has weight and presses in on anything it touches. These are the forces that we are talking about. Anytime force is exerted onto a surface by air is an example of air pressure. Another example is the pressure the weight of an air molecule exerts pressing down on the earth. This is a big component of weather, sea level and atmospheric pressure.
More Science Experiment Fun from Kids Activities Blog
- We have a whole bunch of fun science experiments for kids !
- These Halloween science experiments are scary fun year round!
- Have you read our book on cool science experiments ?
- Let’s make a lemon battery .
- These fun science projects for kids are easy and keep kids curious.
- This baking soda and vinegar experiment is part art.
- Candy corn experiment is tasty fun!
- Science fair project ideas for kids of all ages.
- Make an electromagnetic train
- Let’s learn the heart !
- And don’t miss this really fun list of science activities for preschool .
- Check out what happens when you put marshmallow in a vacuum !
- You can make your very own catapults!
- Check out our list of 20 hands on activities for kids !
- Learn about and how to make pulleys !
- Try building your own atom model !
- Make muscles with balloons with this experiment!
- Print our simple scientific method worksheet for kids
- Balloon static electricity experiment for kids
- Easy science fair projects : experiment with salt!
- Let’s play science games
- Learn how to make a catapult
- Try a defying gravity experiment : cool gravity trick for kids
- Check out this salt and ice easy science experiment !
- Learn how to draw an atom in 6 easy steps!
How did your air pressure experiment go?
Elementary Age Activities for Kids Indoor Kid's Activities Kids Activities Learning Activities Preschool Activities Science Experiments for Kids Science Projects for Kids STEM Activities
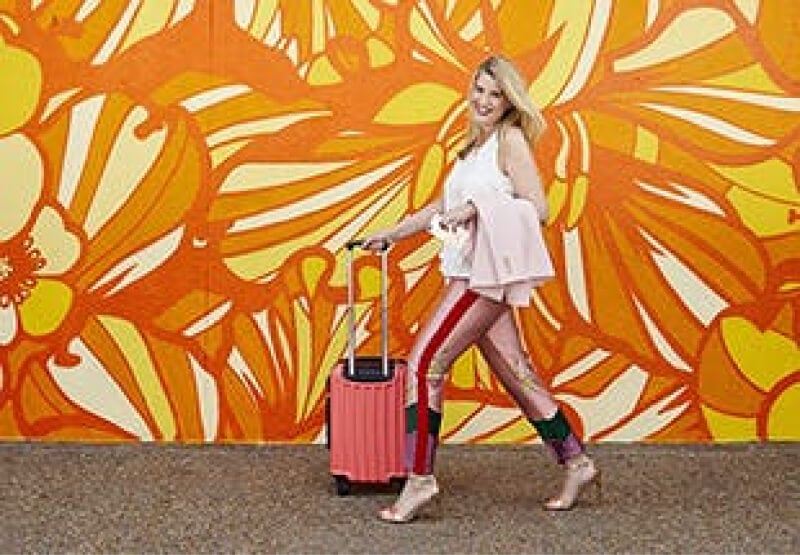
Welcome to Kids Activities!
My name is Holly Homer & I am the Dallas mom of three boys…
Holly's Favorite Activites
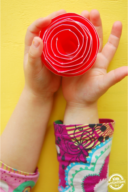
You Might Also Like
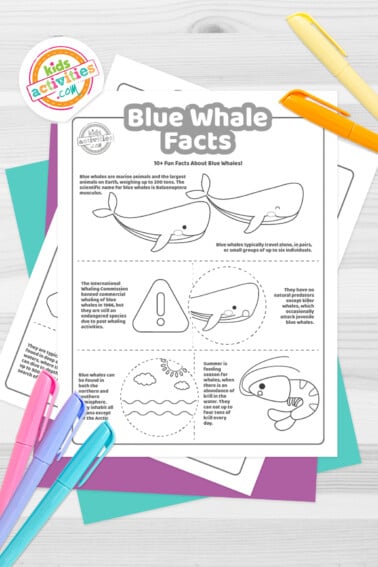
Fun Blue Whale Facts
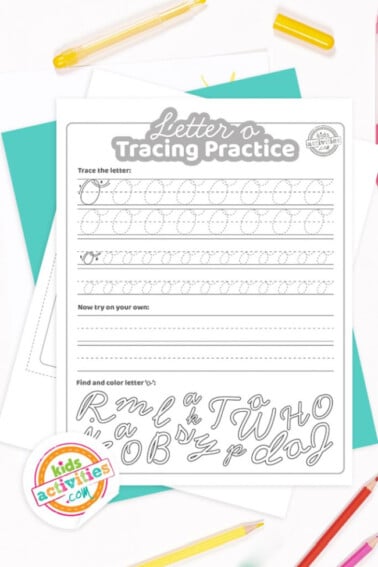
Cursive O Worksheets- Free Printable Cursive Practice Sheets For Letter O
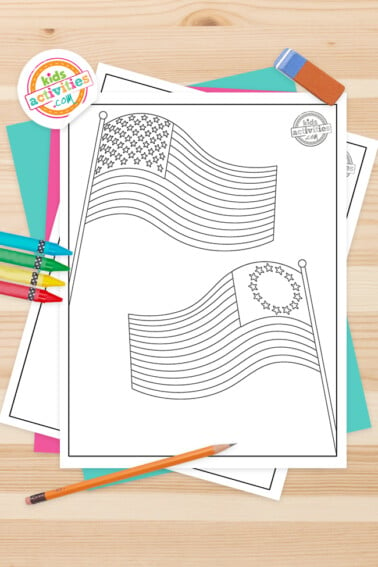
Printable Fun & Patriotic American Flag Coloring Pages

Cursive N Worksheets- Free Printable Cursive Practice Sheets For Letter N
Leave a comment cancel reply.
Your email address will not be published. Required fields are marked *
Display a Gravatar image next to my comments.
18 Comments
Love these activities. Slight edit: (science/math teacher here…) the reason the straw sinks is that the pressure causes the contents of the straw to become MORE DENSE, not heavier. (Think about huge, extremely heavy ships which float, while tiny light pebbles sink). When you squeeze the bottle, the weight of the bottle does not change, but it takes up a smaller amount of space.
Thank you so much for posting these air pressure activities- they are great! I plan to use them as precursors for some paper airplane STEM investigations.
We are so glad that you are enjoying our STEM activities! Thank you for the information!
This is really funny … My boys will like it indeed!????
We tried this but it worked best without the sponges. We can’t figure out the purpose of the sponges.
This is such a great fun idea! We love doing science experiments, especially those that involve a little playing 🙂 Thank you for stopping by the Thoughtful Weekly Blog Hop and linking up! You are featured this week as one of my favorites from last weeks hop!
This is a really cute hands-on science experiment that looks like a lot of fun. We are doing my Weather Detective unit on Pressure soon and I think I will do this experiment with it 🙂 Thank you for sharing and for linking up this week to the Thoughtful Spot Weekly Blog Hop.
My boys would love this acti9vity
Great little science activity for kids! I like it. 🙂
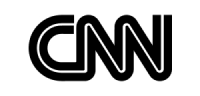
Keep in touch
Connect with us on your favorite social network below!
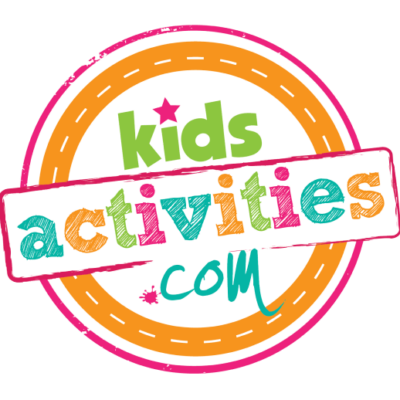
Explore Activities
5 Min Crafts
©2024 Kids Activities Blog. All rights reserved. Disclosure and Privacy Policy • Powered by CultivateWP .
We use cookies on this website to give you the best experience. To find out more, read our Privacy Policy .
8 Student Experiments to Measure Air Quality
As pollution is becoming more rampant worldwide, educators are deeply encouraged to teach students about the science of pollution and what they can do to care for the environment. From the release of greenhouse gases, worsening climate change, and burning of fossil fuels, the rate at which pollutants are produced is unprecedented. Hence, we have suggested 8 experimental ideas that teachers can use to actively involve their students to measure air quality. These ideas range from qualitative to quantitative methods and promote active discussion on which type of method would prove the most effective in producing reliable data. By measuring air quality, students can make sense of the different standards of air quality and recognize when it becomes appropriate to mitigate their effects. Without further ado, let’s jump into it!
PM 2.5 Meter
Particulate Matter (PM) 2.5 refers to particles or compounds which spans less than 2.5 micrometers in diameter. They are fine particles that are commonly produced from factories, vehicle exhaust, burning of crops or volcanic eruptions. Long-term exposure to high concentrations of these particles can put people at risk of developing respiratory illnesses, inflammation, trigger asthma and can even lead to lung cancer.
There are many types of PM 2.5 meters in the market, with some of them capable of measuring other types of pollutants as well. PM 2.5 is most commonly measured in units of µg/m3 (micrograms per meter cubed), or through AQI measurements (meter below ).
Depending on the meter you purchase, it may display either one of the units of measurement. However, keep in mind that the two measurements are not directly proportional to each other as measurements in µg/m3 are representative of the actual amount of PM in the area whereas AQI measurements are subjective to the air quality standards that have been internationally agreed upon for your particular region. This difference in proportionality is represented in the following graph :
Having a corresponding AQI level alongside PM2.5 measurements can help students measure the precise amount of PM2.5 in a certain area and use them to determine the quality of the air. Students could measure air quality in different areas, comparing the results obtained and discuss the probable causes and sources of PM from the location. It is also a great way to measure quantitative values that are useful for writing scientific reports while reinforcing the numbers with a qualitative interpretation of the air quality.
You could perform these measurements with your students for a variety of experiments. For example, by comparing the PM measurements from outdoor areas to indoors, students would become aware of the differences in air quality and discuss the possible outdoor/indoor pollutants that may cause one to have higher PM levels than the other. Another great experiment would be to compare the PM levels of rooms that use purifiers and those that do not. This would demonstrate the effectiveness of air purifiers to students and how they are beneficial to maintaining their general wellbeing.
The following graph compares the different levels of PM 2.5 and US AQI PM 2.5 measurements provided by sarta.innovations2019.org :
Blue Sky Test
The color of the sky is a reliable qualitative method to measure air quality. The color can change due to airborne particles that reflect and refract light. For example, a blue sky would indicate little to no air pollution whereas bright red ones are a result of heavy pollution.
The University of Southern California developed an algorithm through its mobile sensing project to measure the air quality by analyzing pictures of the sky taken from the Android app that they created. The pictures that would be taken would take into account the user’s location, orientation, time taken and transfer the data collected into their server. It would calibrate the image and compare it with their own model of the sky.
Although their app isn’t officially listed in the Play Store, all you have to do is click this link from your Android mobile device and click ‘Download Android App’ on from their website. If your phone is preventing the download, click here to find out more about installing APK files on your phone.
Mountain Visibility Test
Similar to the blue sky test, checking the visibility of mountains, or a large construction that can be seen from a long distance is also a qualitative indicator for air pollution. When we see places that are more polluted, we easily recognize the thick haze and dust that clearly obscures the view. But if we live in a polluted area, a clear view of mountains or other constructions may seem foreign in comparison as demonstrated from the following pictures provided by the US National Park Service :
This method measures visibility as an indicator of air pollution. A great idea is to get in touch with schools from different areas to see if they would like to collaborate to gather picture samples of their view. This way, teachers could show their students what mountains or distant constructions from various places look like. This can prompt a discussion about why some areas are more visible than others while explaining how air pollution impacts the view.
Students could also compare the images with public available AQI data from the region and see if there is a direct correlation between the AQI and visibility. Using the previous AQI Index table, students would be able to understand the different standards of air quality and associate it to qualitative observations on their surrounding environment. Furthermore, you could perform this experiment using AQI websites such as AirVisual or aqicn.org to identify the AQI values of different locations worldwide and compare it with images of landmarks in a particular country from which students could effectively assess the visibility.
This is a simple and easy qualitative analysis that can be performed anywhere in the world. However, it is advisable to make observations in the morning when there is the least fog and other factors impacting visibility .
Sticky Tape Method
Although the most dangerous particles are smaller in size, it is still a good indicator of air pollution to also measure the amount of larger particles such as dust, soot, dirt, smoke that can be potentially seen.
The sticky tape method is very simple, all you have to do is cut a small piece of transparent sticky tape and attach it to the bark of a tree or the surface of a building. Leave it for 10 seconds to let any PM on the surface stick onto the tape, peel the tape off and stick it onto a piece of paper. Students should be advised to label the time and location at which they took the sample.
Students could perform experiments by either collecting tape samples in the same location over different periods of time or taking samples in different locations at a certain period of time depending on their chosen independent and dependent variables. They can make qualitative observations of how PM levels change in different times and locations. This can be expanded by discussing the possible reasons as to why some areas or times have more PM in the air than others.
Lichen Observation & App
Sulfur dioxide (SO2) is a gas with a pungent scent which is known to be harmful towards our health. It is mostly generated from the burning of fossil fuels from industrial processes such as the generation of electricity from burning coal. It reacts to evaporated moisture in the air to produce several acidic compounds such as sulfuric acid, which can cause acid rain when dissolved in rainwater, leading to the acidification of forests.
Nitrogen can also be an overlooked pollutant as it is a common constituent in fertilizers and organic waste from households and sewage. When they have washed away into water bodies, it increases the acidity of the water, causing numerous wildlife deaths and disrupting the ecosystem. Like sulfur dioxide, it also causes acid rain when neutral nitrogen particles react with lightning in the air and mix with rainwater.
Lichen is an effective bio-indicator of sulfur and nitrogen pollutants. If lichen is a naturally occurring substance in your area, it will not be present if they are in the air and there would be green algae in its place. Many more species can act as a bioindicator for particular pollutants depending on vegetation that are sensitive or tolerant to them. A massive study was conducted using lichens to measure the air quality throughout the UK by the OPAL Air Survey .
The study conducted modeled the relationship between lichens as a bioindicator, nitrogenous pollutants, and their climate. Furthermore, the data was easily collected by everyday citizens throughout the UK and can be performed as school experiments as well. The map of the UK on the left demonstrated the amount of nitrogen dioxide (NO2) around the country while the one on the right referred to NHx radicals such as ammonia (NH3) and ammonium (NH4), which can cause ammonia pollution. The following image is their result:
The UK Centre for Ecology & Hydrology developed the Lichen Web-App , which provides guidelines on how to identify what type of lichen is suitable for testing, how to perform chemical tests on them and a comprehensive list of different species that are sensitive or tolerant towards nitrogen. It also enables you to track and record any trunks and branches that have lichens on them. They also created a measurement system called Nitrogen Air Quality Index (NAQI) to accurately associate the different levels of nitrogen to indicate their corresponding level of air quality.
Students could emulate this study on a much smaller scale and explore their environment for lichen or other similar species. This would also make them aware of how vegetation is often sensitive towards pollution.
Palmes Passive Diffusion Tubes
Nitrogen can exist in many forms, one of them being nitrogen dioxide (NO2). Nitrogen dioxide is a gaseous pollutant produced from the burning of fossil fuels such as those in power plants and vehicle exhausts. It undergoes a process in which neutral nitrogen (N2) and oxygen (O2) particles react in high temperatures to produce nitrous oxides (NOx) including NO2, all of which can inflict respiratory conditions such as inflammation, coughing, irritations, etc. This is clearly demonstrated from the image on the right which was performed in an experiment from the University of Edinburgh.
Passive diffusion tubes are an effective long-term method to measure nitrogen dioxide. These small plastic tubes contain a mesh disc made of steel covered with a chemical called triethanolamine (TEA). If nitrogen dioxide is present and passes through the mesh, it would react with TEA and change the color and chemical composition. Diffusion tubes can measure the change in nitrogen dioxide levels over many months inside classrooms or outside your school based on how much TEA is left in the tube.
Ozone Testing Experiments
Ozone (O3) is a gas that is popularly known as a gaseous layer in the stratosphere which protects the earth from harmful UV radiation from the sun. However, ones at the troposphere are mainly the result of the chemical reactions between nitrous oxides (NOx), volatile organic compounds (VOCs) and the sunlight. At high concentrations, they can cause chest pains, coughs, throat irritations and are especially harmful to those suffering from respiratory conditions such as asthma.
We can test for the presence of ozone in two different ways:
Ozone badges are very simple and can be made into different forms. All of them rely on a change in color when high concentrations of ozone are present. The badges as seen from the image are commercially produced indicators that are commonly used by workers who are required to operate in areas with elevated ozone concentrations.
For a more advanced chemical experiment, you could also perform the Schoenbein experiment. Students would require cornstarch and potassium iodide to make indicator strips that would react with ozone if present in the air, evidently turning blue or purple. According to the resulting Schoenbein number from the color scale below, we can determine the amount of ozone present in parts per billion (ppb) as seen from the following from the graph.
It is important to perform this experiment in days with low humidity (the lines from the graph represent how the Schoenbein numbers vary based on the different percentages of humidity in the air). Under these circumstances, ozone would be more likely to break apart into atmospheric oxygen. This experiment also yields the best results in the ozone season, which occurs during heated temperatures throughout the summer and in areas with high vehicle activity.
While this method is relatively safe, it is advised to perform this under the supervision of Chemistry teachers who can provide them with the chemicals and laboratory equipment needed.
Surface Wipes
Surface wipes are similar to the sticky tape method, which simply involves wiping a cotton bud on a surface to observe how much PM was released in a particular time or area. Students can compare the cotton buds that were wiped on the surfaces that are more exposed to the ones less exposed to pollutants, such as on the opposite sides of a handrail or bench. The following video is a lighthearted and entertaining experiment performed by a YouTuber from Sydney to observe the city’s air quality, which has dramatically worsened as of late due to the Australian bushfires:
Teachers and students are encouraged to be creative, improvise and innovate experiments similar to this. That way, educators could create a stimulating and critical learning environment for students to teach them about scientific research methods.
As a teacher or parent, you can choose from a myriad of creative options to teach your child how to measure air quality. Depending on their style of learning and personal preference, you can weigh the benefits of performing qualitative or quantitative methods to help them understand the state of the environment. By performing diverse experiments, they would be able to understand how different collection methods result in corresponding data types. After experimenting with multiple methods, they can then determine which type would be the most suitable to fulfill the research’s purpose. We hope that these experiments would be able to pique their curiosity and encourage them to make meaningful discussions about the health effects and environmental impacts of air pollution!
by Carisa A. Feb 16, 2020
Open and Accurate Air Quality Monitors
We design professional, accurate and long-lasting air quality monitors that are open-source and open-hardware so that you have full control on how you want to use the monitor.
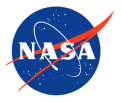
Suggested Searches
Climate Change
- Expedition 64
- Mars perseverance
- SpaceX Crew-2
- International Space Station
- View All Topics A-Z
Humans in Space
Earth & Climate
The solar system, the universe, aeronautics, learning resources, news & events.
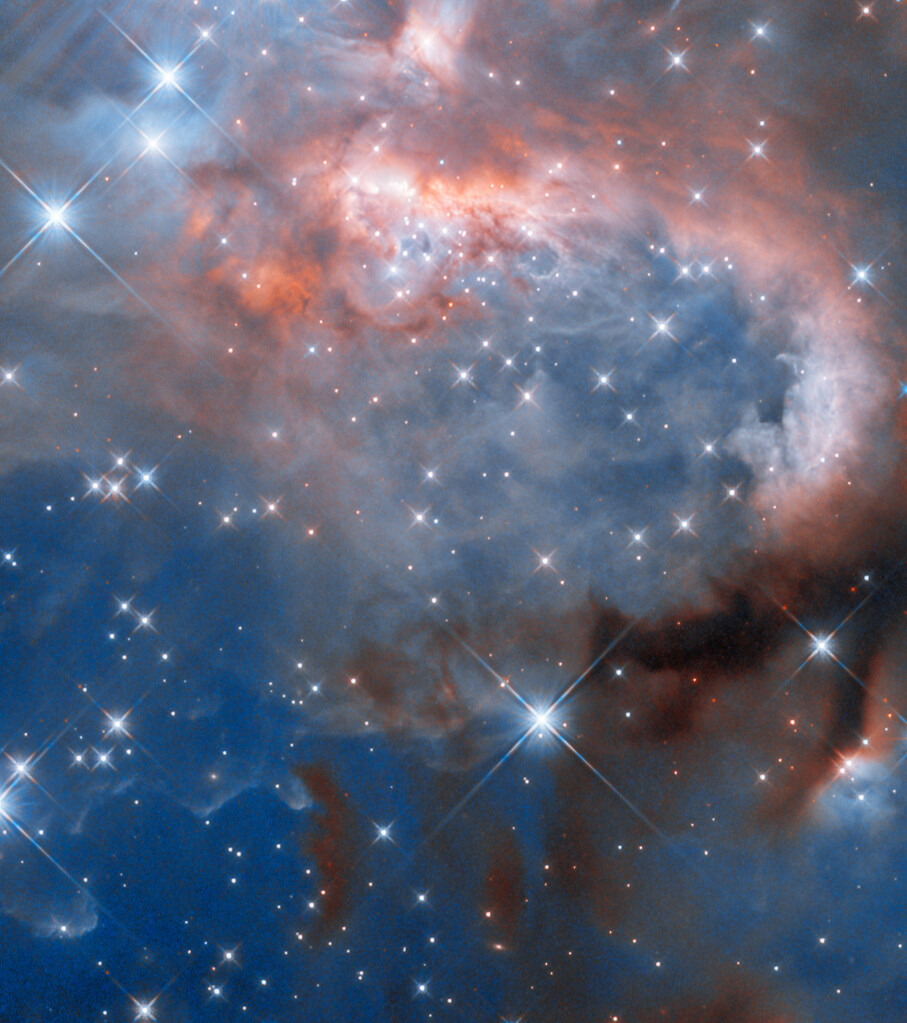
Hubble Captures Infant Stars Transforming a Nebula
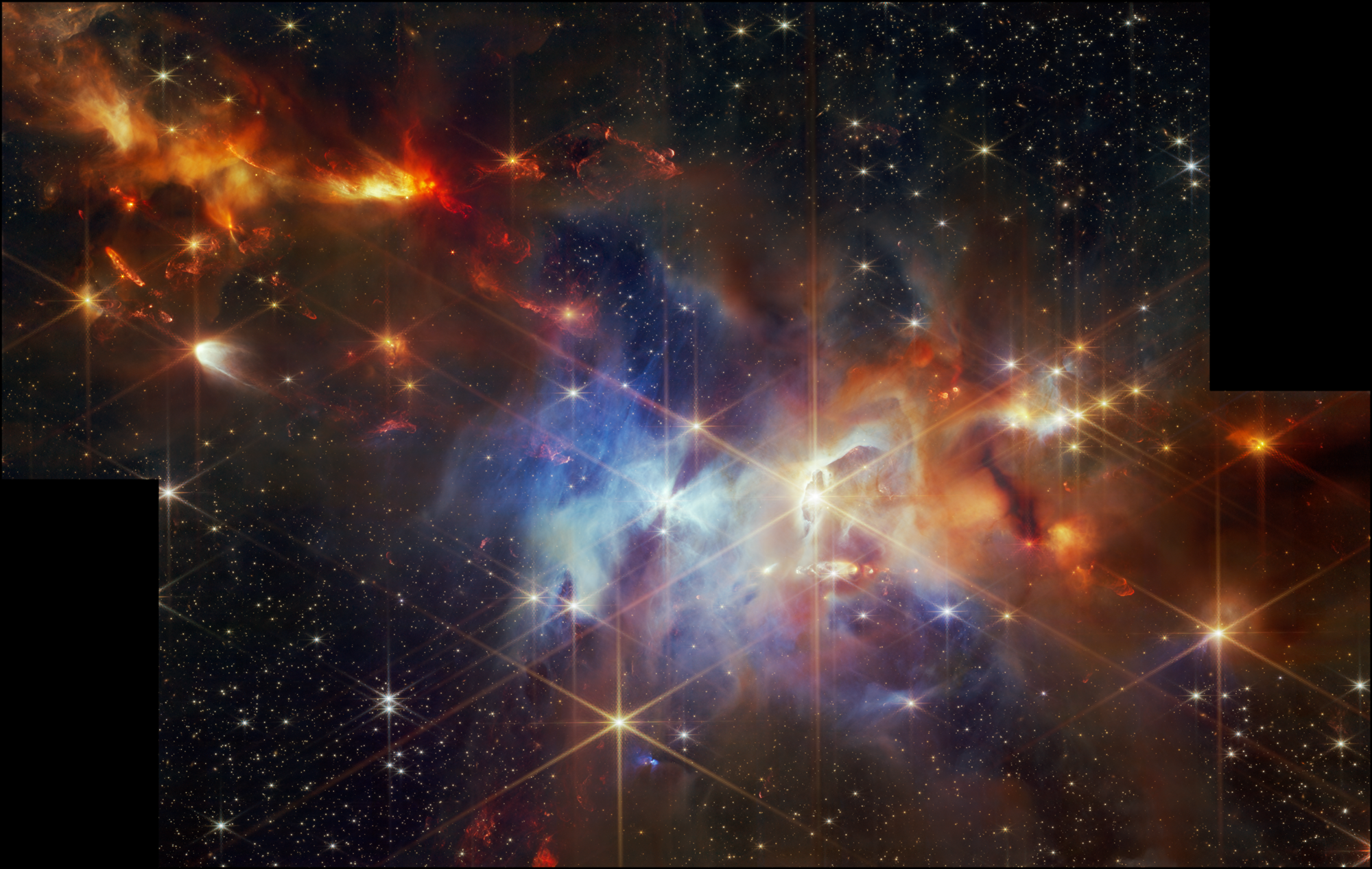
First of Its Kind Detection Made in Striking New Webb Image
Why scientists are intrigued by air in nasa’s mars sample tubes.
- Search All NASA Missions
- A to Z List of Missions
- Upcoming Launches and Landings
- Spaceships and Rockets
- Communicating with Missions
- James Webb Space Telescope
- Hubble Space Telescope
- Why Go to Space
- Commercial Space
- Destinations
- Living in Space
- Explore Earth Science
- Earth, Our Planet
- Earth Science in Action
- Earth Multimedia
- Earth Science Researchers
- Pluto & Dwarf Planets
- Asteroids, Comets & Meteors
- The Kuiper Belt
- The Oort Cloud
- Skywatching
- The Search for Life in the Universe
- Black Holes
- The Big Bang
- Dark Energy & Dark Matter
- Earth Science
- Planetary Science
- Astrophysics & Space Science
- The Sun & Heliophysics
- Biological & Physical Sciences
- Lunar Science
- Citizen Science
- Astromaterials
- Aeronautics Research
- Human Space Travel Research
- Science in the Air
- NASA Aircraft
- Flight Innovation
- Supersonic Flight
- Air Traffic Solutions
- Green Aviation Tech
- Drones & You
- Technology Transfer & Spinoffs
- Space Travel Technology
- Technology Living in Space
- Manufacturing and Materials
- Science Instruments
- For Kids and Students
- For Educators
- For Colleges and Universities
- For Professionals
- Science for Everyone
- Requests for Exhibits, Artifacts, or Speakers
- STEM Engagement at NASA
- NASA's Impacts
- Centers and Facilities
- Directorates
- Organizations
- People of NASA
- Internships
- Our History
- Doing Business with NASA
- Get Involved
- Aeronáutica
- Ciencias Terrestres
- Sistema Solar
- All NASA News
- Video Series on NASA+
- Newsletters
- Social Media
- Media Resources
- Upcoming Launches & Landings
- Virtual Events
- Sounds and Ringtones
- Interactives
- STEM Multimedia

Unity in Orbit: Astronauts Soar with Pride Aboard Station

Six Adapters for Crewed Artemis Flights Tested, Built at NASA Marshall

Gateway: Up Close in Stunning Detail
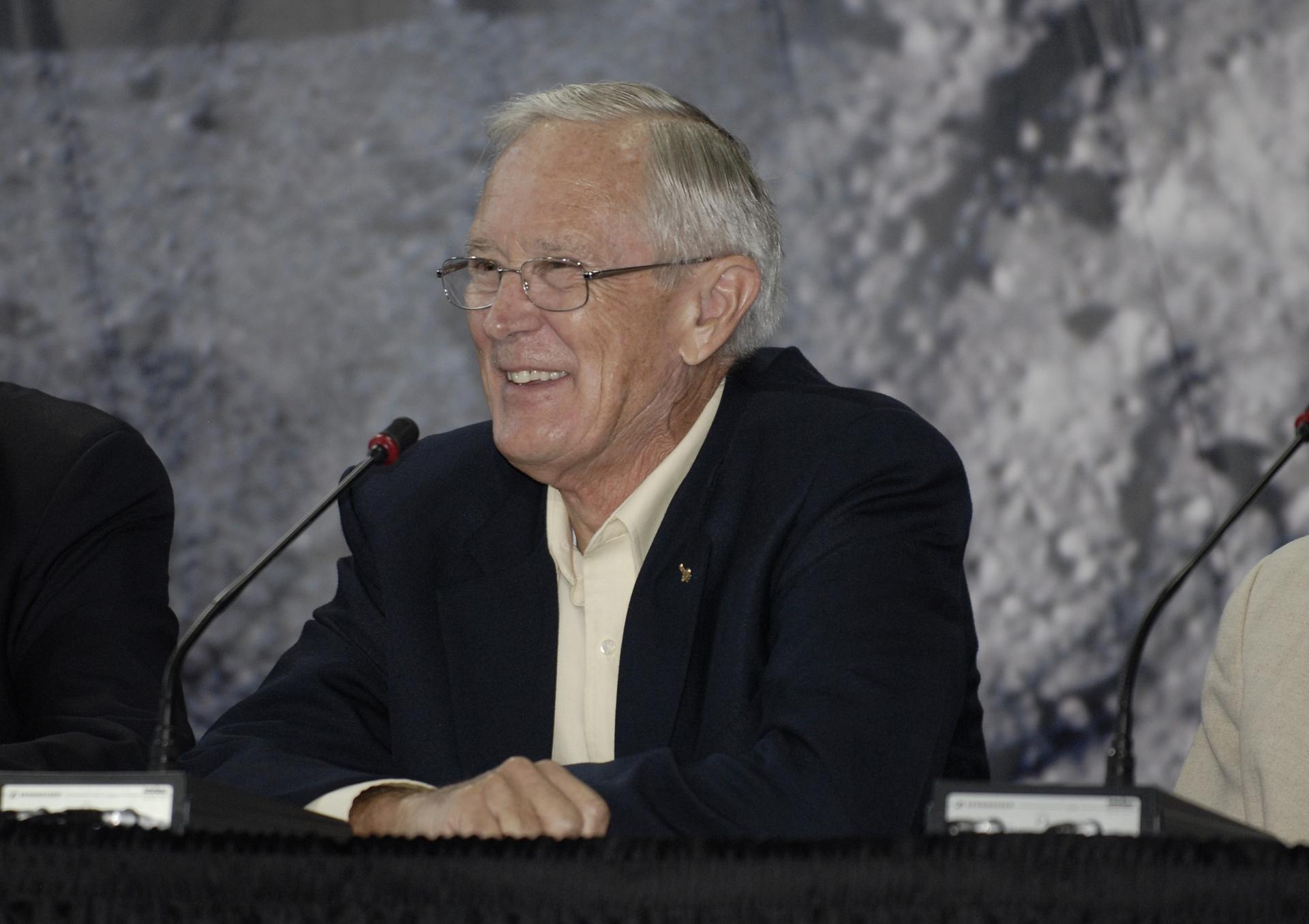
Former Astronaut Charles M. Duke, Jr.
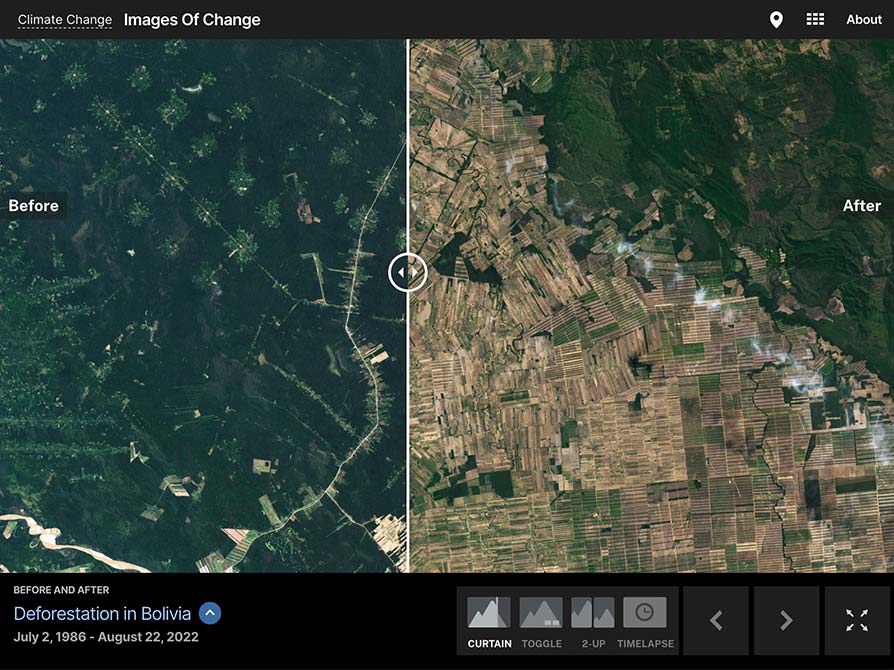
Climate Interactives
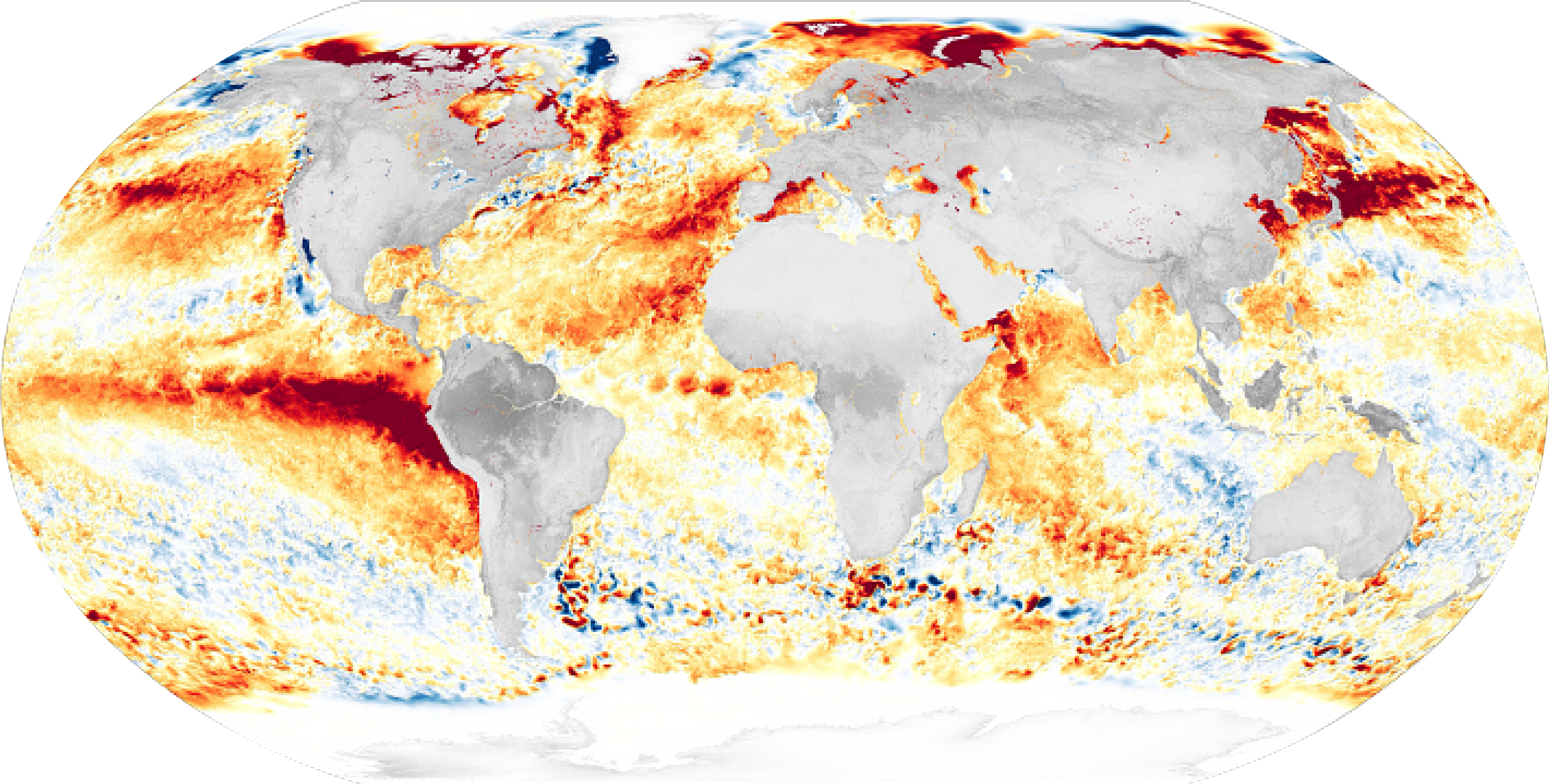
The Ocean and Climate Change
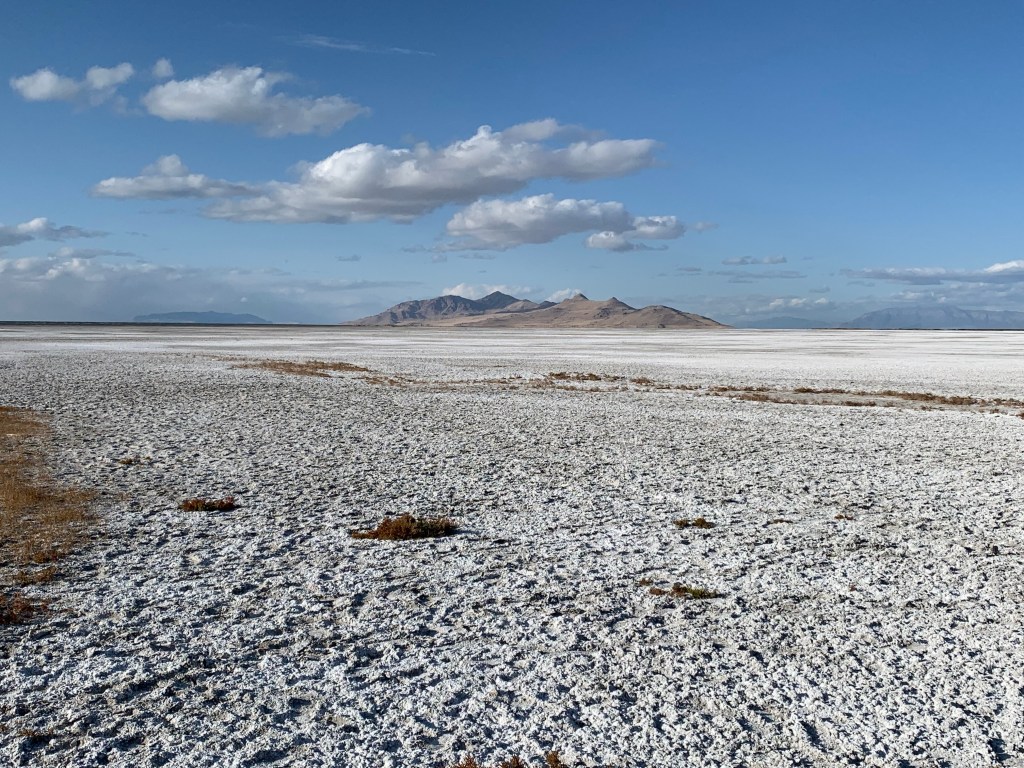
NASA Satellites Find Snow Didn’t Offset Southwest US Groundwater Loss
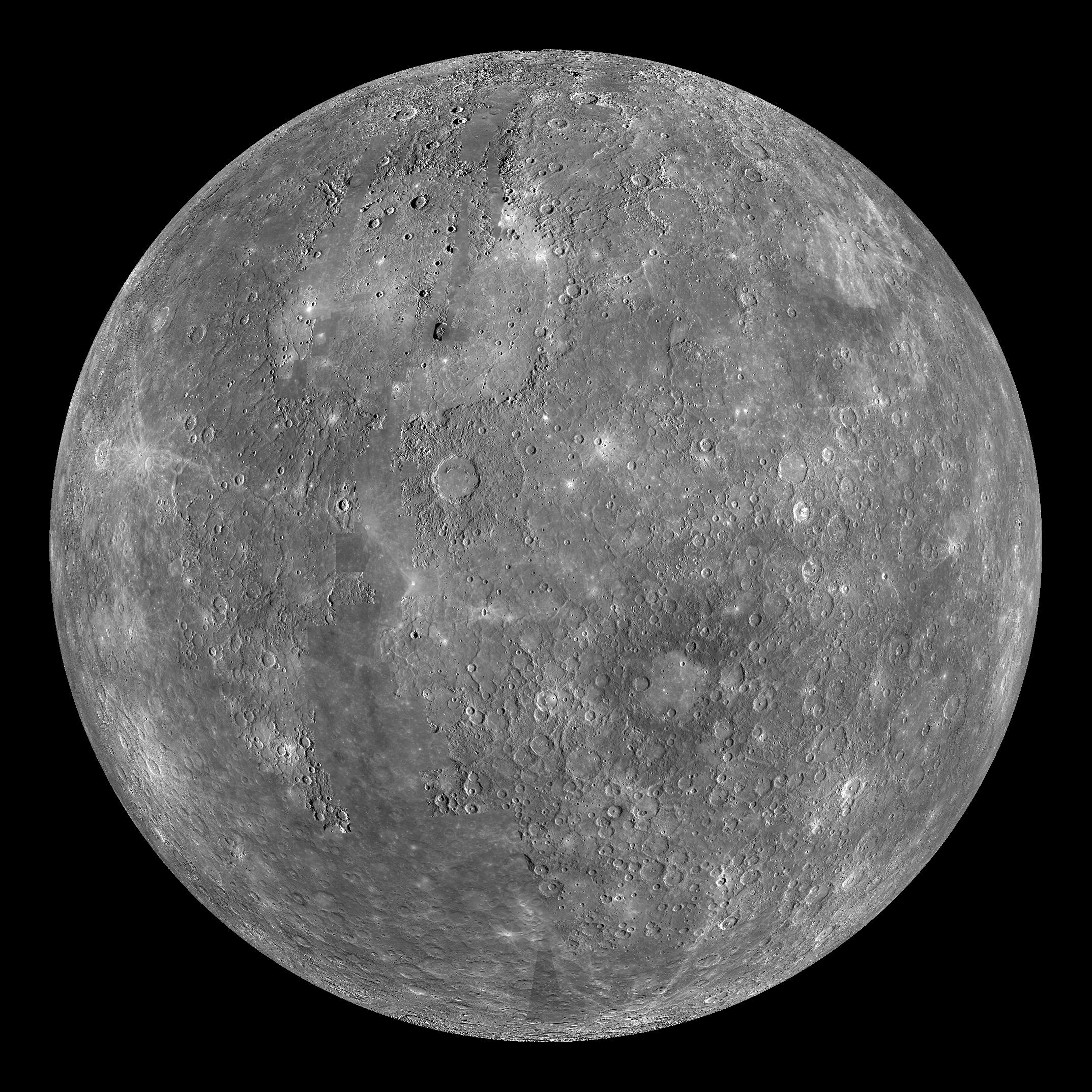
Mercury Resources
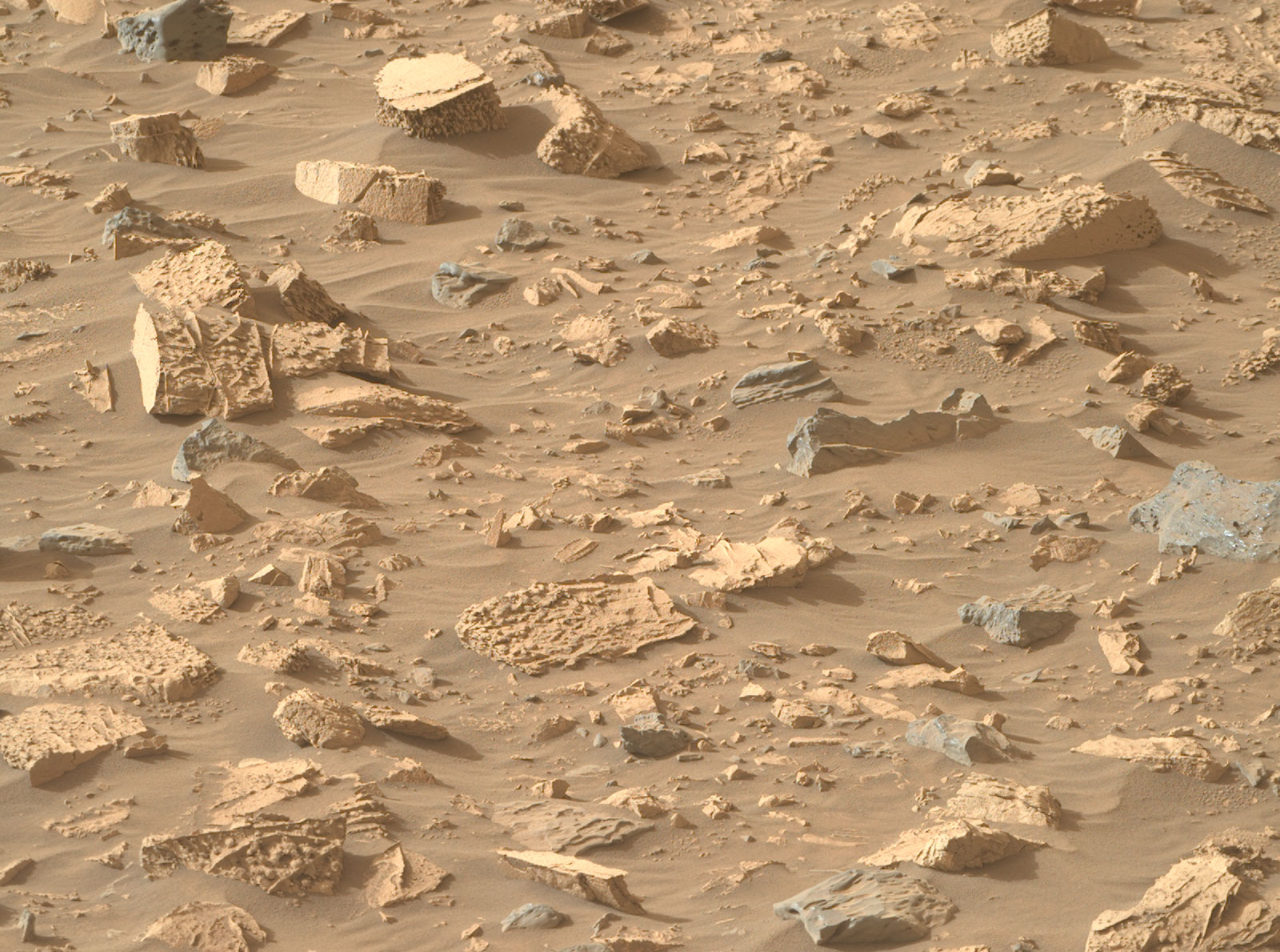
Perseverance Finds Popcorn on Planet Mars
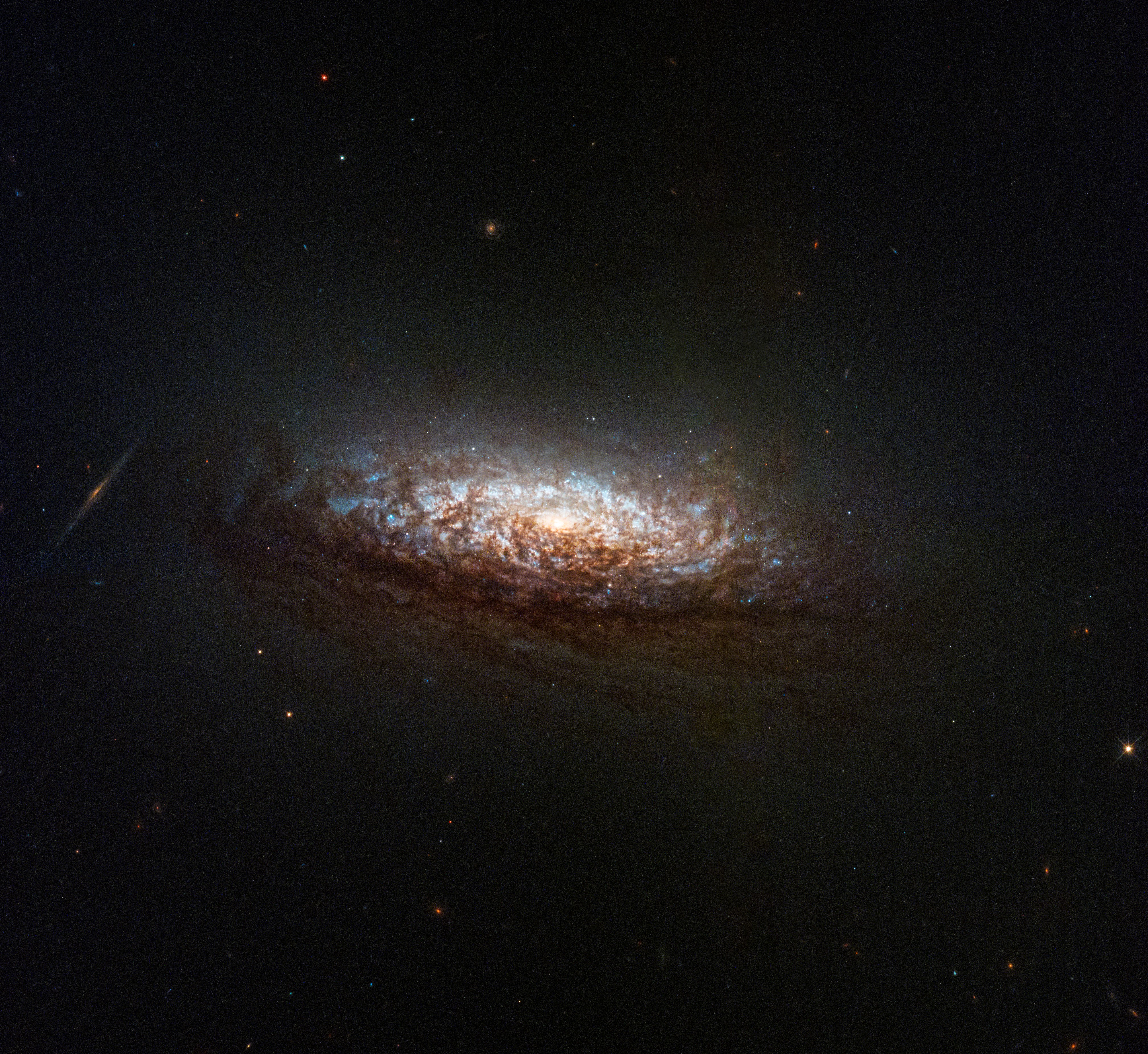
NASA Releases Hubble Image Taken in New Pointing Mode
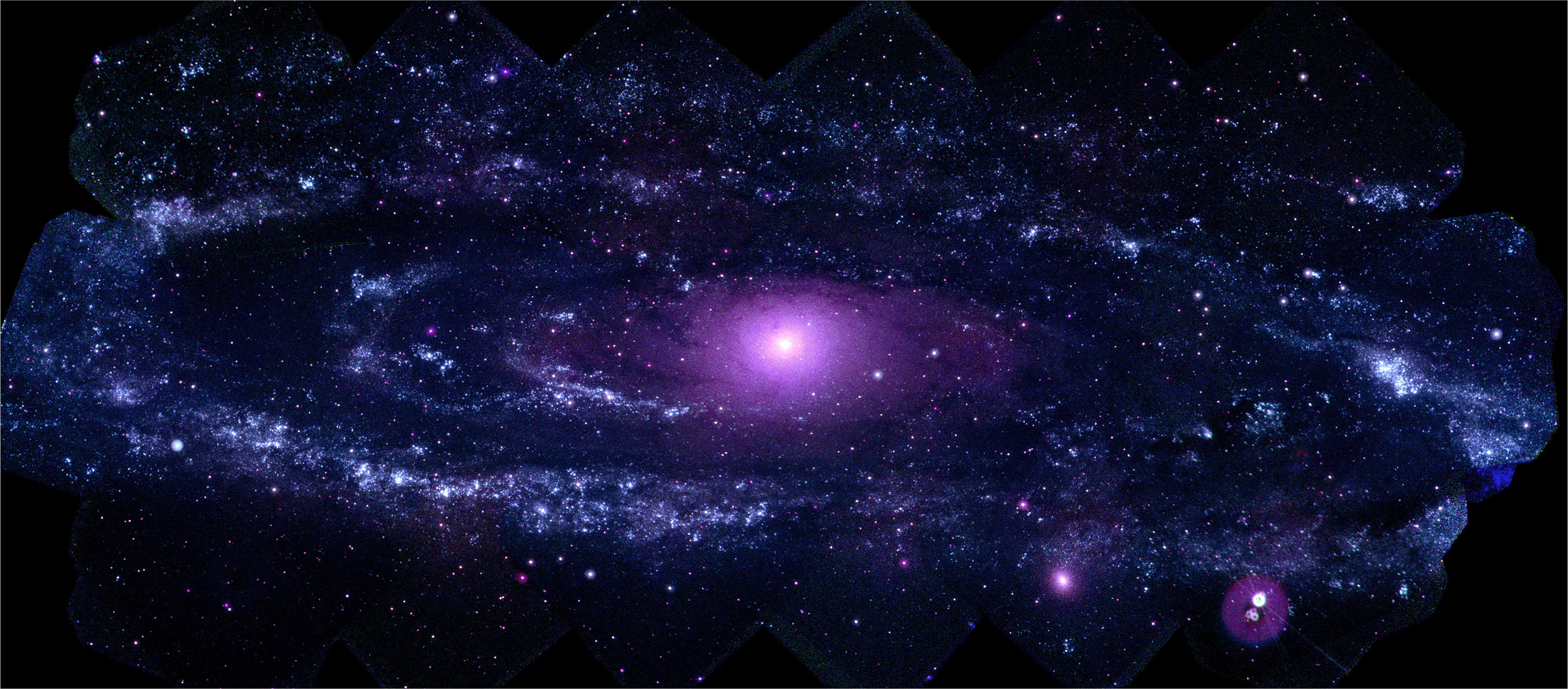
Amendment 23: D.5 Swift General Investigator Amendment: IXPE Joint Observing Program and Change to NuSTAR Exclusive-Use Period.
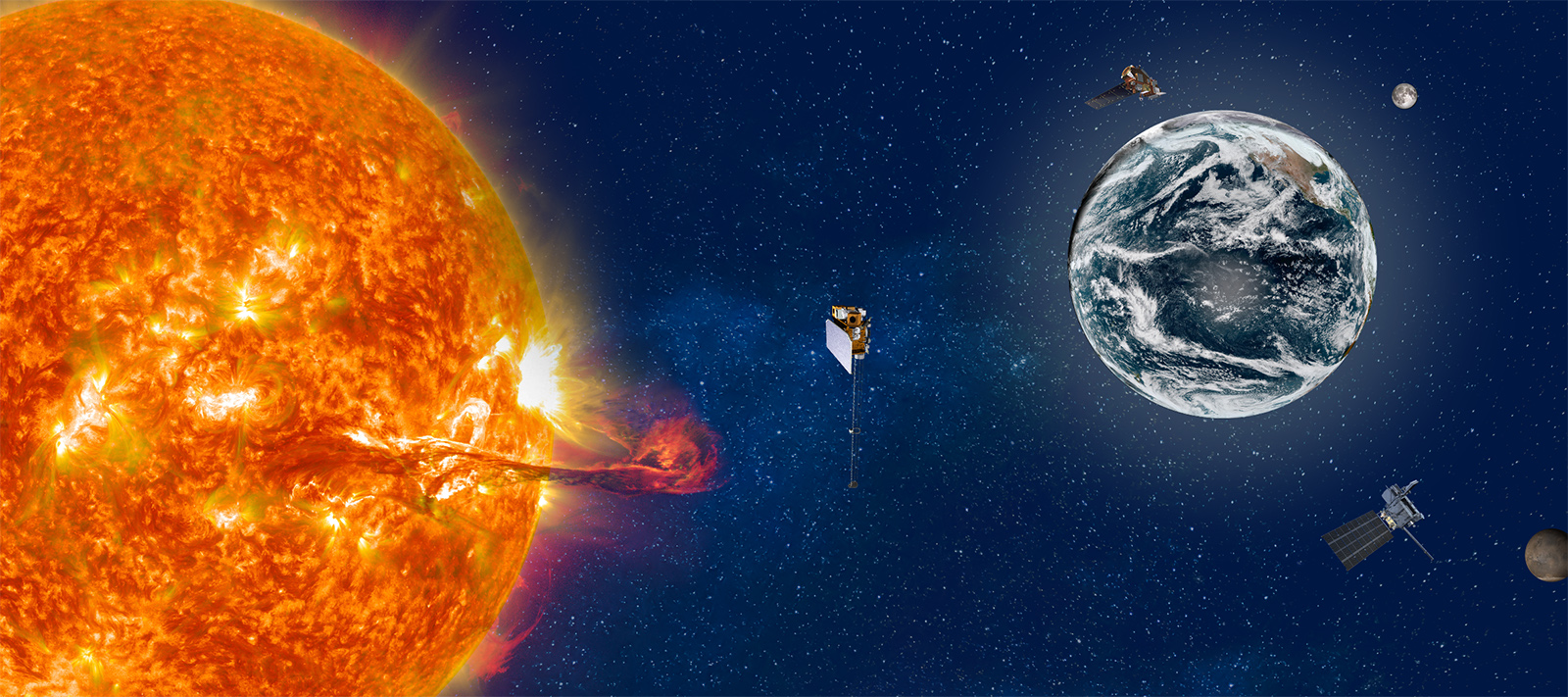
Amendment 22: Heliophysics Flight Opportunities in Research and Technology Final Text and Due Date
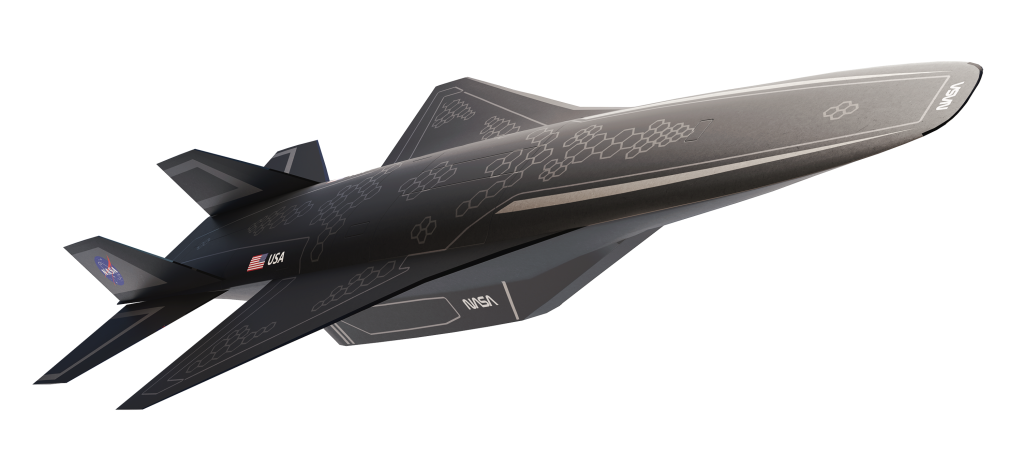
Hypersonic Technology Project
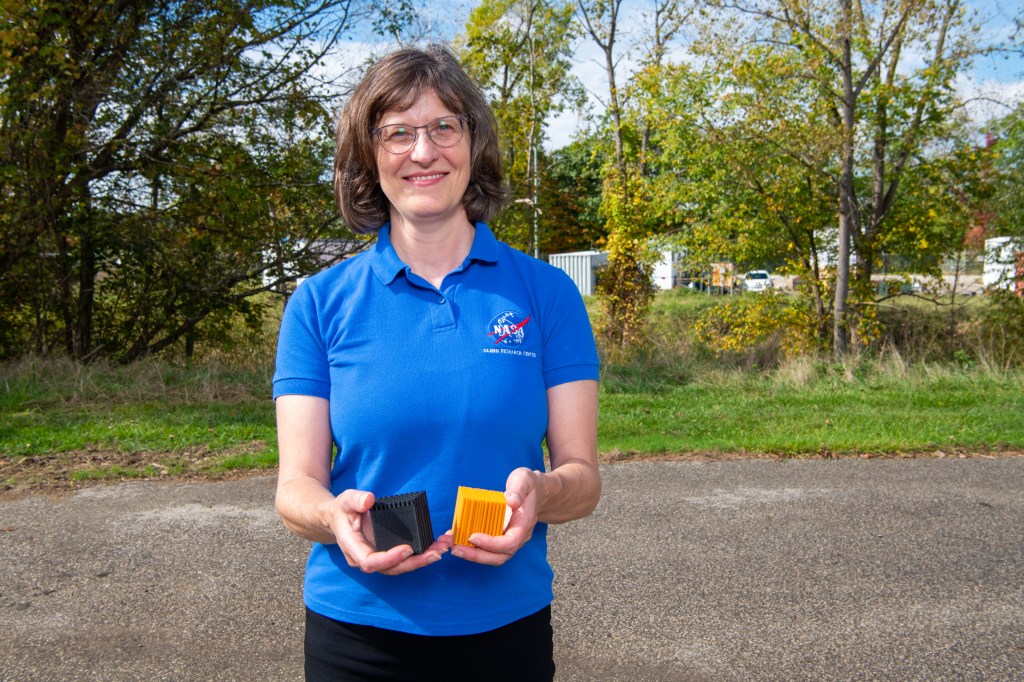
NASA Engineer Honored as Girl Scouts ‘Woman of Distinction’
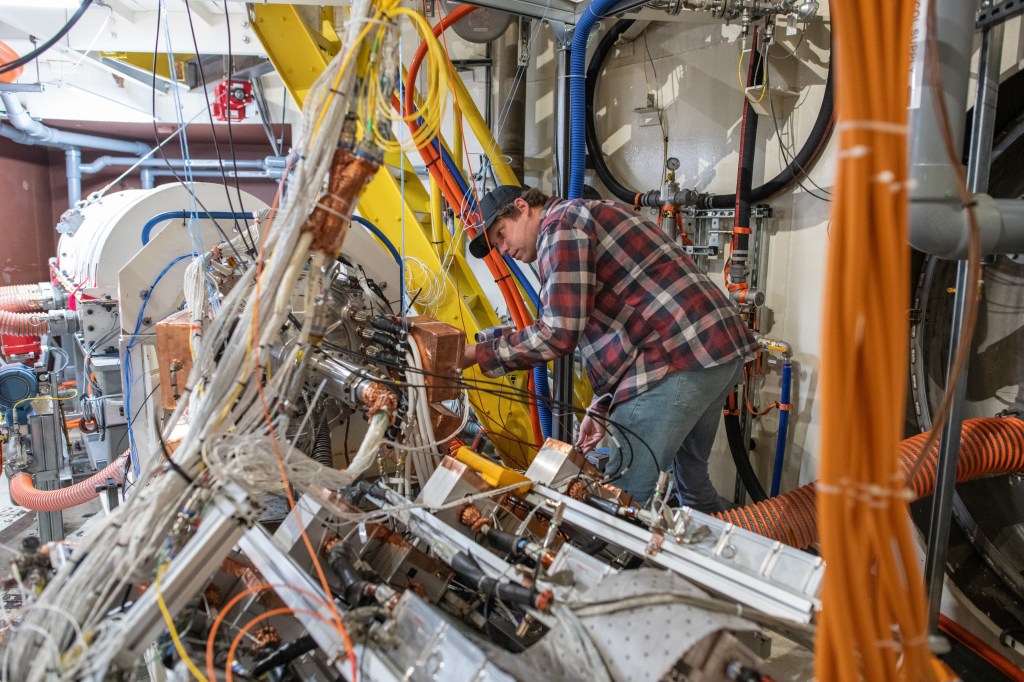
NASA, MagniX Altitude Tests Lay Groundwork for Hybrid Electric Planes

Augmented Reality Speeds Spacecraft Construction at NASA Goddard
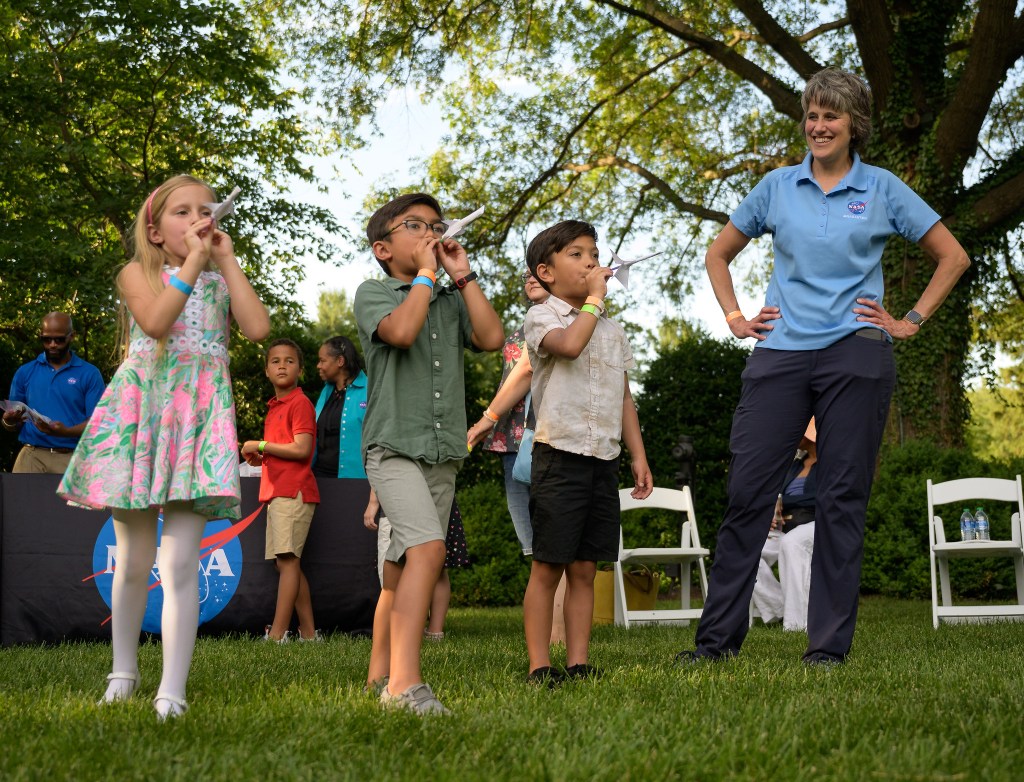
Slow Your Student’s ‘Summer Slide’ and Beat Boredom With NASA STEM

NASA Webb, Hubble Scientist Marcia Rieke Awarded Gruber Cosmology Prize
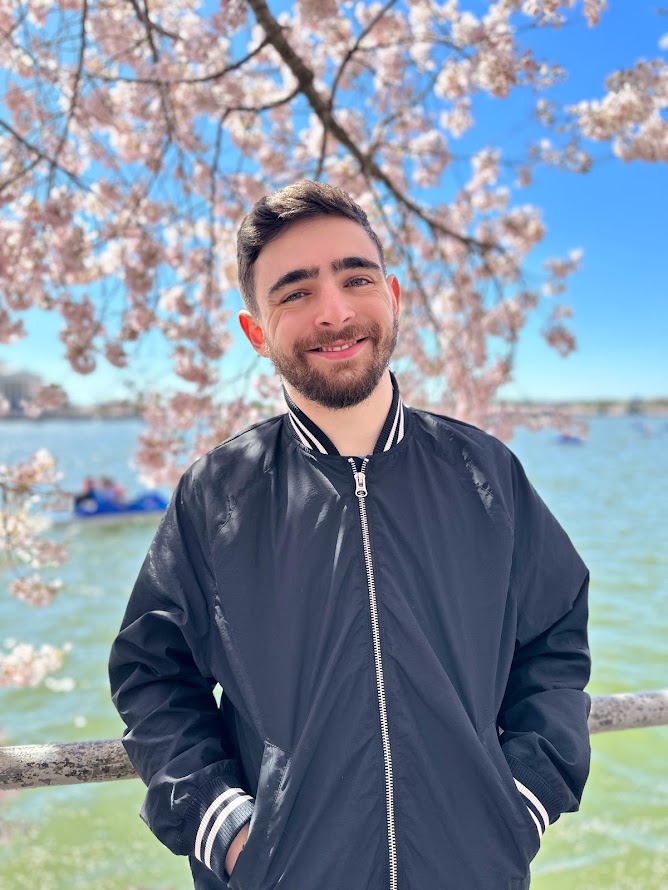
Jake Cupani: Increasing Visibility in Data Science
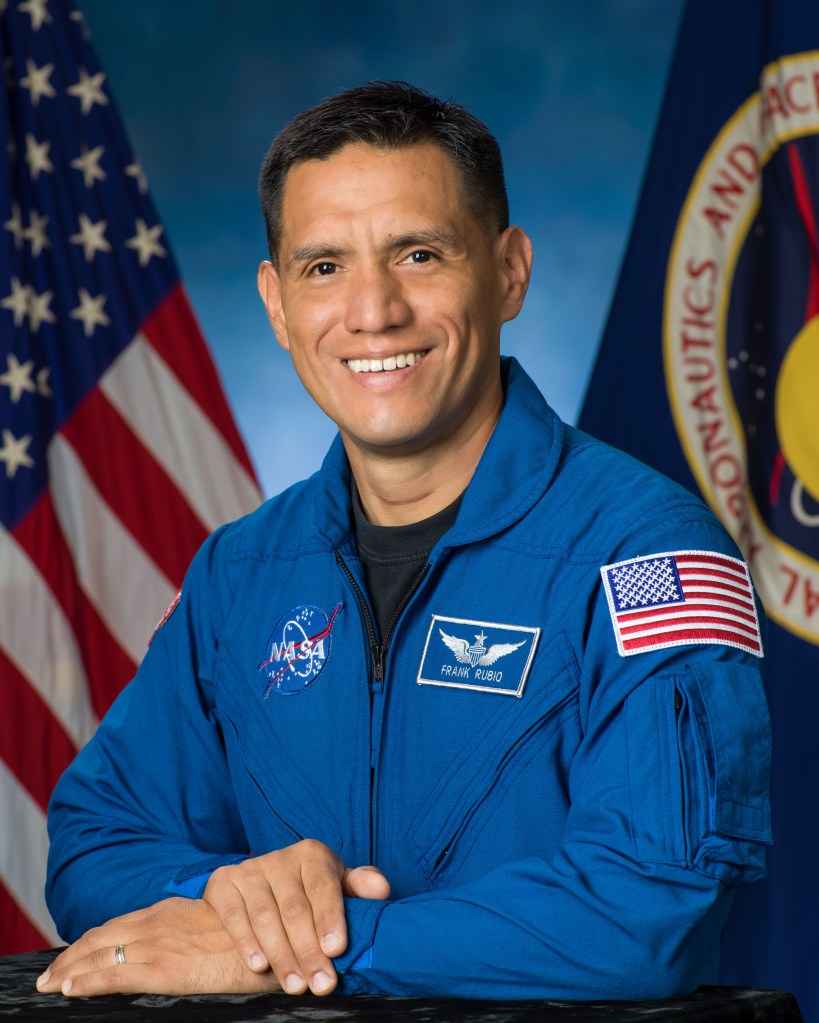
Astronauta de la NASA Frank Rubio
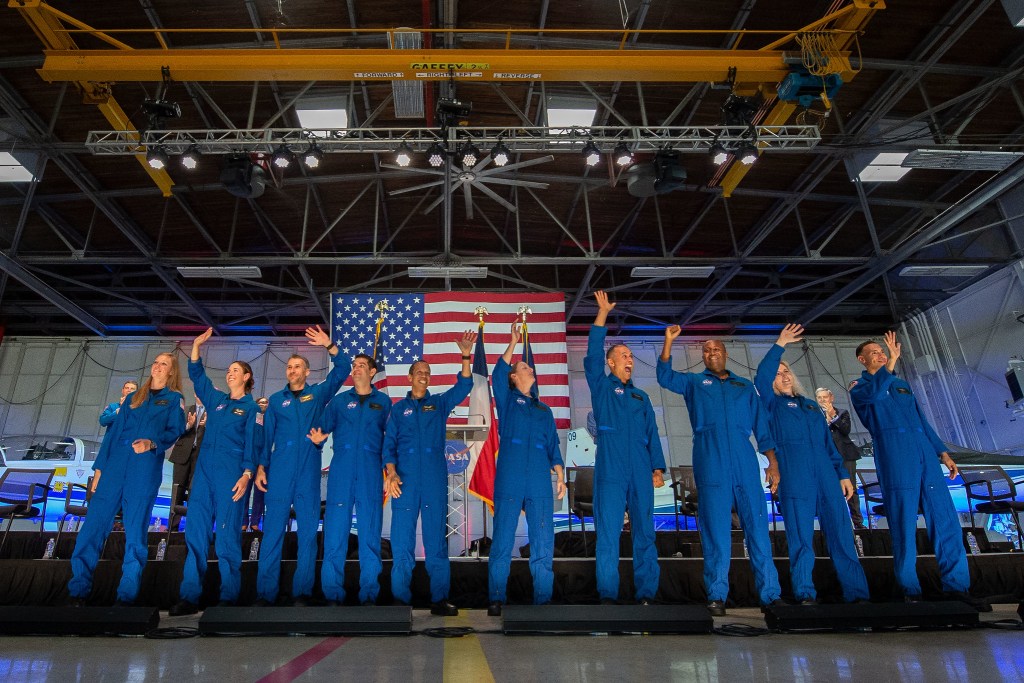
Diez maneras en que los estudiantes pueden prepararse para ser astronautas
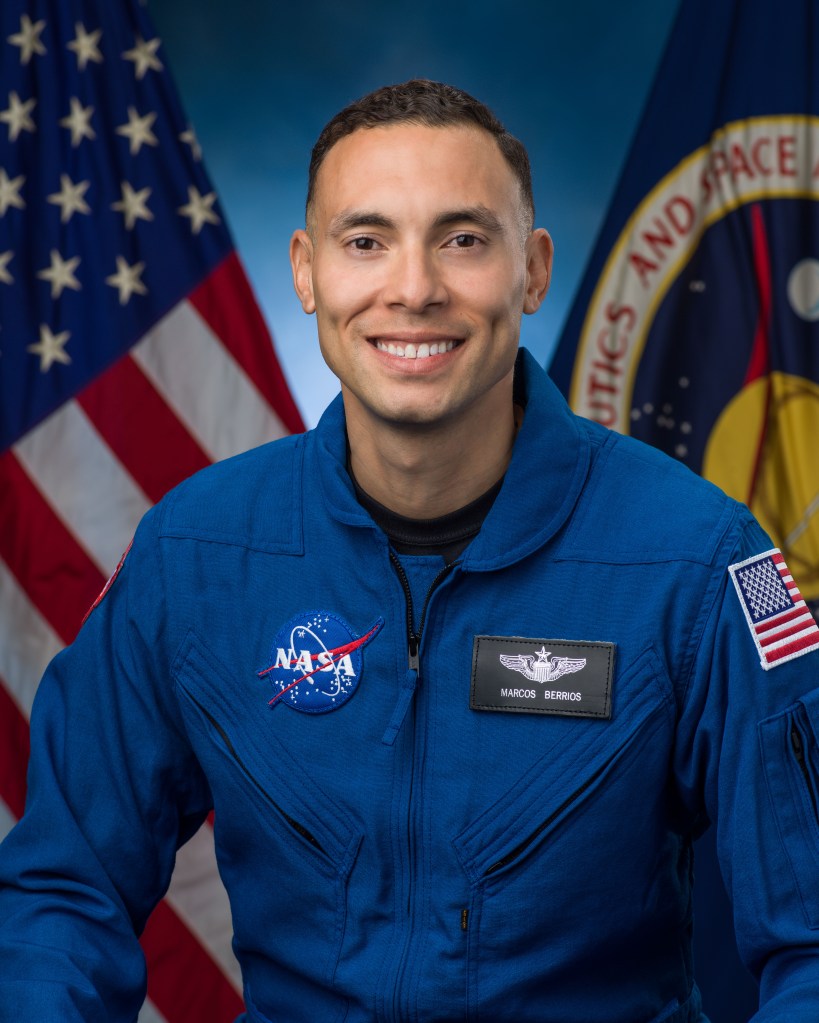
Astronauta de la NASA Marcos Berríos
- Jet Propulsion Laboratory
The Value of Headspace
Apollo’s air samples, more about the mission, news media contacts.
Tucked away with each rock and soil sample collected by the agency’s Perseverance rover is a potential boon for atmospheric scientists.
Atmospheric scientists get a little more excited with every rock core NASA’s Perseverance Mars rover seals in its titanium sample tubes, which are being gathered for eventual delivery to Earth as part of the Mars Sample Return campaign . Twenty-four have been taken so far.
Most of those samples consist of rock cores or regolith (broken rock and dust) that might reveal important information about the history of the planet and whether microbial life was present billions of years ago. But some scientists are just as thrilled at the prospect of studying the “headspace,” or air in the extra room around the rocky material, in the tubes.
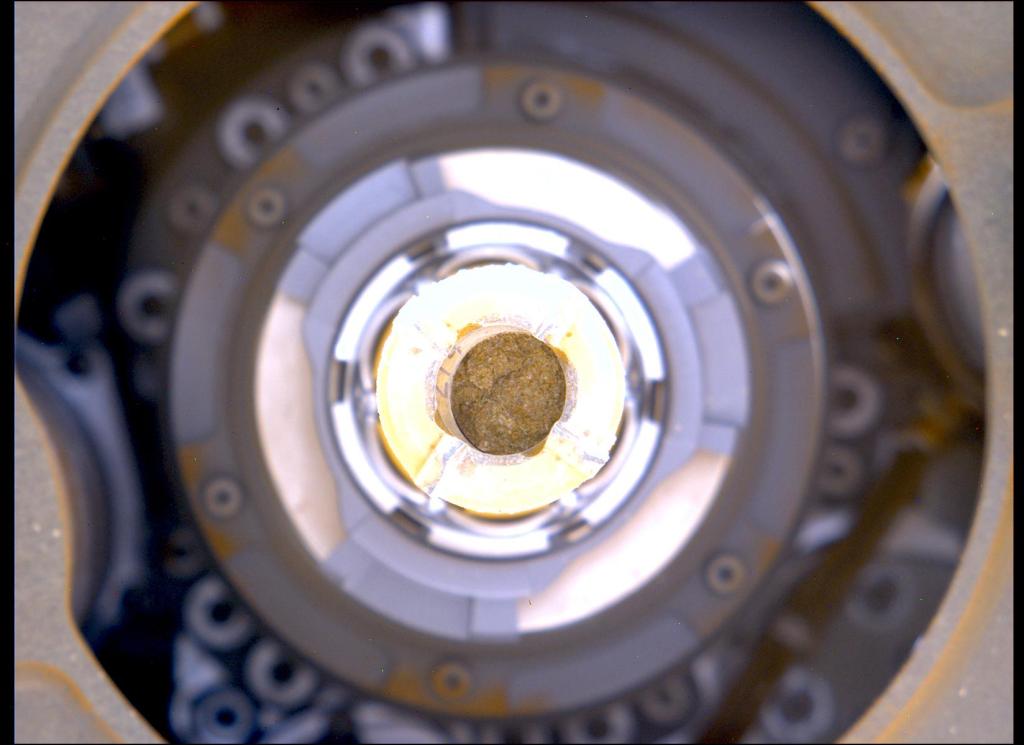
They want to learn more about the Martian atmosphere, which is composed mostly of carbon dioxide but could also include trace amounts of other gases that may have been around since the planet’s formation.
“The air samples from Mars would tell us not just about the current climate and atmosphere, but how it’s changed over time,” said Brandi Carrier, a planetary scientist at NASA’s Jet Propulsion Laboratory in Southern California. “It will help us understand how climates different from our own evolve.”
Among the samples that could be brought to Earth is one tube filled solely with gas deposited on the Martian surface as part of a sample depot . But far more of the gas in the rover’s collection is within the headspace of rock samples. These are unique because the gas will be interacting with rocky material inside the tubes for years before the samples can be opened and analyzed in laboratories on Earth. What scientists glean from them will lend insight into how much water vapor hovers near the Martian surface, one factor that determines why ice forms where it does on the planet and how Mars’ water cycle has evolved over time.
Scientists also want a better understanding of trace gases in the air at Mars. Most scientifically tantalizing would be the detection of noble gases (such as neon, argon, and xenon), which are so nonreactive that they may have been around, unchanged in the atmosphere, since forming billions of years ago. If captured, those gases could reveal whether Mars started with an atmosphere. (Ancient Mars had a much thicker atmosphere than it does today, but scientists aren’t sure whether it was always there or whether it developed later). There are also big questions about how the planet’s ancient atmosphere compared with early Earth’s.
The headspace would additionally provide a chance to assess the size and toxicity of dust particles — information that will help future astronauts on Mars.
“The gas samples have a lot to offer Mars scientists,” said Justin Simon, a geochemist at NASA’s Johnson Space Center in Houston, who is part of a group of over a dozen international experts that helps decide which samples the rover should collect. “Even scientists who don’t study Mars would be interested because it will shed light on how planets form and evolve.”
In 2021, a group of planetary researchers, including scientists from NASA, studied the air brought back from the Moon in a steel container by Apollo 17 astronauts some 50 years earlier.
“People think of the Moon as airless, but it has a very tenuous atmosphere that interacts with the lunar surface rocks over time,” said Simon, who studies a variety of planetary samples at Johnson. “That includes noble gases leaking out of the Moon’s interior and collecting at the lunar surface.”
The way Simon’s team extracted the gas for study is similar to what could be done with Perseverance’s air samples. First, they put the previously unopened container into an airtight enclosure. Then they pierced the steel with a needle to extract the gas into a cold trap — essentially a U-shaped pipe that extends into a liquid, like nitrogen, with a low freezing point. By changing the temperature of the liquid, scientists captured some of the gases with lower freezing points at the bottom of the cold trap.
“There’s maybe 25 labs in the world that manipulate gas in this way,” Simon said. Besides being used to study the origin of planetary materials, this approach can be applied to gases from hot springs and those emitted from the walls of active volcanoes, he added.
Of course, those sources provide much more gas than Perseverance has in its sample tubes. But if a single tube doesn’t carry enough gas for a particular experiment, Mars scientists could combine gases from multiple tubes to get a larger aggregate sample — one more way the headspace offers a bonus opportunity for science.
A key objective for Perseverance’s mission on Mars is astrobiology , including the search for signs of ancient microbial life. The rover is also characterizing the planet’s geology and past climate, which paves the way for human exploration of the Red Planet. JPL, which is managed for NASA by Caltech in Pasadena, California, built and manages operations of the Perseverance rover.
For more about Perseverance:
mars.nasa.gov/mars2020/
Andrew Good Jet Propulsion Laboratory, Pasadena, Calif. 818-393-2433 [email protected]
Karen Fox / Charles Blue NASA Headquarters, Washington 202-285-1600 / 202-802-5345 [email protected] / [email protected]
Related Terms
- Perseverance (Rover)
- Astrobiology
- Johnson Space Center
- Planetary Environments & Atmospheres
Explore More
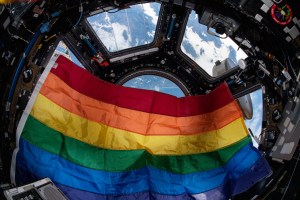
Witness Gateway in stunning detail with this video that brings the future of lunar exploration…
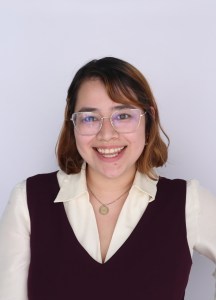
Johnson Celebrates LGBTQI+ Pride Month: Eva Granger
Discover related topics.
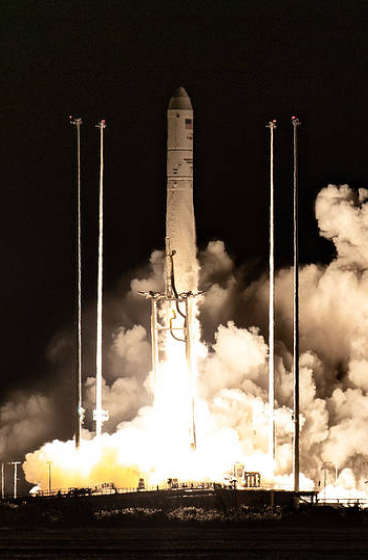
Solar System
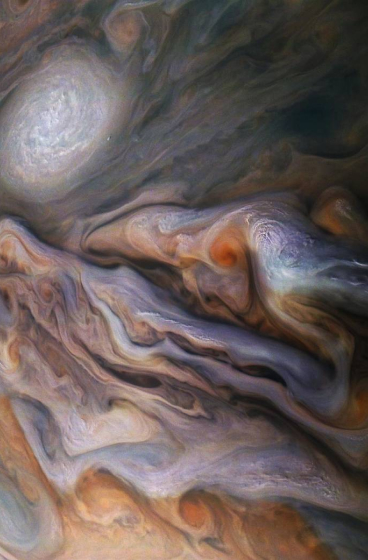
share this!
June 20, 2024
This article has been reviewed according to Science X's editorial process and policies . Editors have highlighted the following attributes while ensuring the content's credibility:
fact-checked
trusted source
Why scientists are intrigued by air in NASA's Mars sample tubes
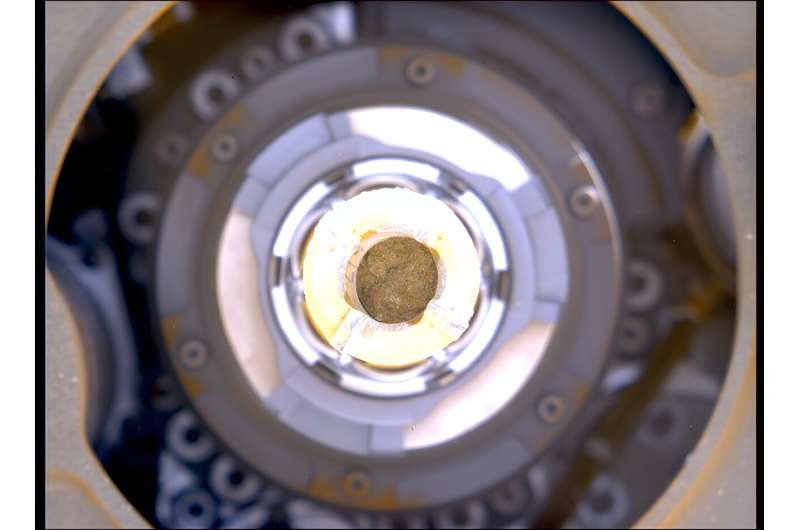
Atmospheric scientists get a little more excited with every rock core NASA's Perseverance Mars rover seals in its titanium sample tubes, which are being gathered for eventual delivery to Earth as part of the Mars Sample Return campaign. Twenty-four have been taken so far.
Most of those samples consist of rock cores or regolith (broken rock and dust) that might reveal important information about the history of the planet and whether microbial life was present billions of years ago. But some scientists are just as thrilled at the prospect of studying the "headspace," or air in the extra room around the rocky material, in the tubes.
They want to learn more about the Martian atmosphere, which is composed mostly of carbon dioxide but could also include trace amounts of other gases that may have been around since the planet's formation.
"The air samples from Mars would tell us not just about the current climate and atmosphere, but how it's changed over time," said Brandi Carrier, a planetary scientist at NASA's Jet Propulsion Laboratory in Southern California. "It will help us understand how climates different from our own evolve."
The value of headspace
Among the samples that could be brought to Earth is one tube filled solely with gas deposited on the Martian surface as part of a sample depot. But far more of the gas in the rover's collection is within the headspace of rock samples. These are unique because the gas will be interacting with rocky material inside the tubes for years before the samples can be opened and analyzed in laboratories on Earth.
What scientists glean from them will lend insight into how much water vapor hovers near the Martian surface, one factor that determines why ice forms where it does on the planet and how Mars' water cycle has evolved over time.
Scientists also want a better understanding of trace gases in the air at Mars. Most scientifically tantalizing would be the detection of noble gases (such as neon, argon, and xenon), which are so nonreactive that they may have been around, unchanged in the atmosphere, since forming billions of years ago.
If captured, those gases could reveal whether Mars started with an atmosphere. (Ancient Mars had a much thicker atmosphere than it does today, but scientists aren't sure whether it was always there or whether it developed later). There are also big questions about how the planet's ancient atmosphere compared with early Earth's.
The headspace would additionally provide a chance to assess the size and toxicity of dust particles—information that will help future astronauts on Mars.
"The gas samples have a lot to offer Mars scientists," said Justin Simon, a geochemist at NASA's Johnson Space Center in Houston, who is part of a group of over a dozen international experts that helps decide which samples the rover should collect. "Even scientists who don't study Mars would be interested because it will shed light on how planets form and evolve."
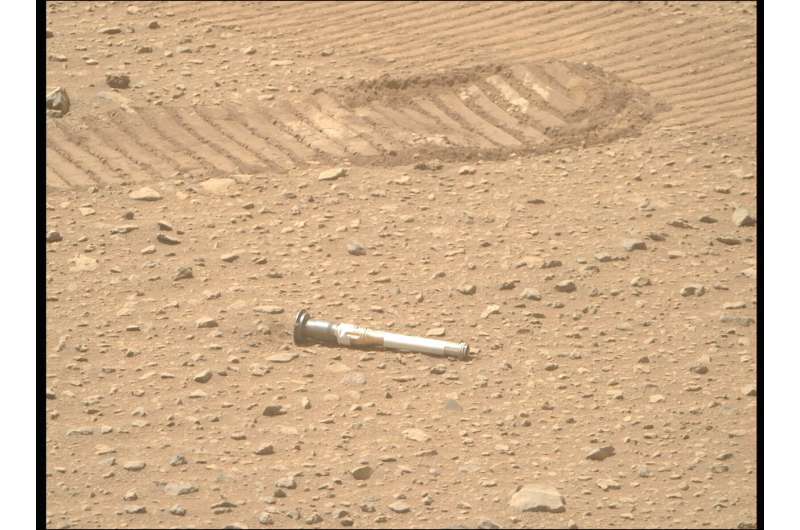
Apollo's air samples
In 2021, a group of planetary researchers, including scientists from NASA, studied the air brought back from the moon in a steel container by Apollo 17 astronauts some 50 years earlier.
"People think of the moon as airless, but it has a very tenuous atmosphere that interacts with the lunar surface rocks over time," said Simon, who studies a variety of planetary samples at Johnson. "That includes noble gases leaking out of the moon's interior and collecting at the lunar surface."
The way Simon's team extracted the gas for study is similar to what could be done with Perseverance's air samples. First, they put the previously unopened container into an airtight enclosure. Then they pierced the steel with a needle to extract the gas into a cold trap—essentially a U-shaped pipe that extends into a liquid, like nitrogen, with a low freezing point. By changing the temperature of the liquid, scientists captured some of the gases with lower freezing points at the bottom of the cold trap.
"There's maybe 25 labs in the world that manipulate gas in this way," Simon said. Besides being used to study the origin of planetary materials, this approach can be applied to gases from hot springs and those emitted from the walls of active volcanoes, he added.
Of course, those sources provide much more gas than Perseverance has in its sample tubes. But if a single tube doesn't carry enough gas for a particular experiment, Mars scientists could combine gases from multiple tubes to get a larger aggregate sample—one more way the headspace offers a bonus opportunity for science.
Provided by NASA
Explore further
Feedback to editors

Discovery of vast sex differences in cellular activity has major implications for disease treatment
7 minutes ago

Researchers discover new flat electronic bands, paving way for advanced quantum materials
21 minutes ago

Not all calcite crystals perfect; synthesis methods can alter internal structure, affect chemical reactivity
22 minutes ago

Boosting 'natural killer' cell activity could improve cancer therapy
3 hours ago

AI predicts upper secondary education dropout as early as the end of primary school

Study reveals how one enzyme hitches a ride on another to recognize tRNA

1,500-year-old reliquary discovered

Multidrug-resistant fungi found in commercial soil, compost, flower bulbs
4 hours ago

3D-printed chip sensor detects foodborne pathogens for safer products
5 hours ago

Bats use four key tactics for accurate target tracking
Relevant physicsforums posts, interstellar navigation.
2 hours ago
Questions about dark matter/energy
6 hours ago
Some photos of Antares and Sigma sagittarii (Nunki)
Jun 23, 2024
Why is the CNO Cycle considered catalytic in stellar nucleosynthesis?
Is the universe older than we think.
Jun 22, 2024
Will we ever communicate with extraterrestial life in a reasonable time frame?
Jun 19, 2024
More from Astronomy and Astrophysics
Related Stories

Could Martian atmospheric samples teach us more about the red planet than surface samples?
May 27, 2024

NASA's Perseverance rover shows off collection of Mars samples
Feb 14, 2023

Perseverance rover collects first Mars sample of new science campaign
Apr 1, 2023

NASA is seeking a faster, cheaper way to bring Mars samples to Earth
Apr 16, 2024

NASA's Perseverance rover collects two samples of Martian regolith
Dec 7, 2022

Video: Picking up lightsabers for Mars
May 29, 2023
Recommended for you

Jupiter's upper atmosphere surprises astronomers
8 hours ago

Detecting intelligent life that's light years away: Greenhouse gases could signal alien activity
9 hours ago

Hydrothermal vents on seafloors of 'ocean worlds' could support life, new study says
Jun 24, 2024

Geologists expect Chang'e-6 lunar surface samples to contain volcanic rock and impact ejecta

Astronomers find three potential super-Earths around nearby star

Mystery of massive aurora in Arctic skies in December 2022 solved by astronomers
Let us know if there is a problem with our content.
Use this form if you have come across a typo, inaccuracy or would like to send an edit request for the content on this page. For general inquiries, please use our contact form . For general feedback, use the public comments section below (please adhere to guidelines ).
Please select the most appropriate category to facilitate processing of your request
Thank you for taking time to provide your feedback to the editors.
Your feedback is important to us. However, we do not guarantee individual replies due to the high volume of messages.
E-mail the story
Your email address is used only to let the recipient know who sent the email. Neither your address nor the recipient's address will be used for any other purpose. The information you enter will appear in your e-mail message and is not retained by Phys.org in any form.
Newsletter sign up
Get weekly and/or daily updates delivered to your inbox. You can unsubscribe at any time and we'll never share your details to third parties.
More information Privacy policy
Donate and enjoy an ad-free experience
We keep our content available to everyone. Consider supporting Science X's mission by getting a premium account.
E-mail newsletter
Thank you for visiting nature.com. You are using a browser version with limited support for CSS. To obtain the best experience, we recommend you use a more up to date browser (or turn off compatibility mode in Internet Explorer). In the meantime, to ensure continued support, we are displaying the site without styles and JavaScript.
- View all journals
- Explore content
- About the journal
- Publish with us
- Sign up for alerts
- Open access
- Published: 25 June 2024
Large contribution of in-cloud production of secondary organic aerosol from biomass burning emissions
- Tiantian Wang 1 ,
- Kun Li 1 nAff2 ,
- David M. Bell 1 ,
- Jun Zhang 1 ,
- Tianqu Cui 1 ,
- Mihnea Surdu 1 ,
- Urs Baltensperger ORCID: orcid.org/0000-0003-0079-8713 1 ,
- Jay G. Slowik 1 ,
- Houssni Lamkaddam 1 ,
- Imad El Haddad ORCID: orcid.org/0000-0002-2461-7238 1 &
- Andre S. H. Prevot ORCID: orcid.org/0000-0002-9243-8194 1
npj Climate and Atmospheric Science volume 7 , Article number: 149 ( 2024 ) Cite this article
Metrics details
- Atmospheric chemistry
- Environmental impact
Organic compounds released from wildfires and residential biomass burning play a crucial role in shaping the composition of the atmosphere. The solubility and subsequent reactions of these compounds in the aqueous phase of clouds and fog remain poorly understood. Nevertheless, these compounds have the potential to become an important source of secondary organic aerosol (SOA). In this study, we simulated the aqueous SOA (aqSOA) from residential wood burning emissions under atmospherically relevant conditions of gas-liquid phase partitioning, using a wetted-wall flow reactor (WFR). We analyzed and quantified the specific compounds present in these emissions at a molecular level and determined their solubility in clouds. Our findings reveal that while 1% of organic compounds are fully water-soluble, 19% exhibit moderate solubility and can partition into the aqueous phase in a thick cloud. Furthermore, it is found that the aqSOA generated in our laboratory experiments has a substantial fraction being attributed to the formation of oligomers in the aqueous phase. We also determined an aqSOA yield of 20% from residential wood combustion, which surpasses current estimates based on gas-phase oxidation. These results indicate that in-cloud chemistry of organic gases emitted from wood burning can serve as an efficient pathway to produce organic aerosols, thus potentially influencing climate and air quality.
Introduction
Organic aerosol (OA) accounts for a substantial mass fraction of submicron aerosol 1 . Understanding OA sources is a critical environmental challenge that is required to reduce some of the larger uncertainties in assessing radiative climate forcing and air quality management policies 2 , 3 , 4 . OA consists of primary organic aerosol (POA), directly emitted into the atmosphere, and secondary organic aerosol (SOA), formed via the conversion of volatile organic compounds (VOCs) into low-volatility species. Bottom-up and top-down estimates confirm that SOA accounts for up to 76% of ambient OA 5 , 6 , 7 . Over the past decades, our knowledge of SOA production via gas-phase chemistry has greatly improved, whereas SOA production via in-cloud chemistry has received far less attention. Water-soluble organic gases (WSOGs) can react in aqueous media to form highly oxygenated, low-volatility, aqueous SOA (aqSOA) 8 , 9 , 10 , 11 . Notably, during haze episodes in China and foggy periods in the Po Valley, remarkably high concentrations of aqSOA have been observed 10 , 12 . Although it is expected that biomass burning emissions are present during these events, the vapors contributing to aqueous SOA production during these events remain unknown. Biomass burning (BB) emissions, including those from residential burning, represent the second largest global source of non-methane organic gases (NMOGs) after biogenic emissions 13 , 14 . Despite advancements in the chemical characterization of organic gases and a comprehensive summary of Henry’s law constants 15 , 16 , 17 , the water solubility and potential contribution of BB gases to aqSOA formation remain unknown. BB emissions consist of numerous compounds, many of which could play a role in aqSOA formation 18 . Consequently, the impact of complex primary BB emissions on SOA formation remains largely understudied, necessitating a holistic characterization and quantification.
Because of technological limitations, typical laboratory experiments fail to mimic aqSOA formation from in-cloud processes under atmospherically relevant conditions. Cloud chambers in laboratories produce clouds with extremely short lifetimes (a few minutes), while bulk solution experiments overestimate the liquid water content (LWC) in the atmosphere by at least six orders of magnitude (10 6 g m −3 in bulk water vs. 1 g m −3 in thick clouds). Furthermore, the current literature on aqSOA formation primarily focuses on individual dissolved compounds (e.g., glycolaldehyde, acetic acid, levoglucosan, phenol, vanillin, and guaiacol) 19 , 20 , 21 , 22 . However, there is a lack of studies on aqSOA formation from complex mixtures of WSOGs from real-world biomass burning emissions. The highly variable nature of biomass burning emissions poses a great challenge for their investigation using continuous flow reactors.
To overcome these limitations, a wetted-wall flow reactor (WFR) was used in combination with a holding tank to simulate in-cloud production of aqSOA from residential wood burning emissions. The WFR, previously utilized for investigating isoprene cloud chemistry with an atmospherically relevant aqueous solution composition 23 , was applied to a system of complex emissions. The WFR was maintained at approximately 100% relative humidity (RH), while organic gases from residential wood burning were introduced into the WFR along with OH radicals and water vapor under kinetic conditions. The gas-phase chemical composition was quantified and characterized using a suite of mass spectrometers. We determined the water solubility of biomass burning vapors based on their uptake into the water layer. Compounds formed through aqueous-phase oxidation in the WFR were nebulized to determine the yields, bulk chemical composition and molecular composition of the resulting aqSOA. We compared our laboratory findings with the chemical composition of gasSOA from the same BB emissions.
Molecular composition of gaseous organic compounds from primary residential wood burning emissions
Previous online measurements of organic gases from residential wood burning primarily utilized a proton transfer reaction mass spectrometer (PTR-MS), and over 85% of the measured NMOG mass from residential wood combustion emissions have been identified, which provides assigned molecular formulae and structural information to observed ions using the PTR-MS 24 , 25 . As shown in Fig. 1 , the 15 species with the highest concentration as measured by the PTR-MS are structurally assigned based on previously identified residential wood combustion products, which account for roughly 80% of the total NMOGs across our entire experiment sets and belong to the VOC volatility class (log 10 ( C *) > 6.5 μg m −3 ). However, in previous studies intermediate-volatility organic compounds (IVOCs, 2.5 μg m −3 < log 10 ( C *) < 6.5 μg m −3 ) were likely not detected by the PTR-MS. To measure the IVOCs, a Vocus PTR-TOF-MS (Tofwerk; hereafter referred to as Vocus) and a dual-phase extractive electrospray ionization time-of-flight mass spectrometer (Dual-EESI for short) (Tofwerk/Aerodyne) were also used 26 . The combination of the Vocus and Dual-EESI detected 680 elemental formulae between m/z 40 and m/z 350 (Supplementary Fig. 1 ). The IVOCs identified by both instruments contributed approximately 15.7% of the total vapor concentration.
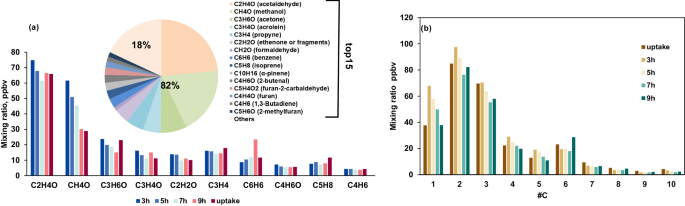
a Concentrations of the 10 most abundant VOCs (or VOC fragments) measured by the PTR-MS in wood burning primary emissions, where the bar colors denote the different experiments in the WFR. The pie chart presents the average contribution of mixing ratios in one wood burning experiment. b Carbon number distributions of wood burning primary emissions measured by the PTR-MS for the different WFR experiments.
The gas-phase analysis revealed several dominant species: C 2 H 4 O 2 (acetic acid, 10.4% of the total concentration), C 2 H 4 O (acetaldehyde, 7%), C 3 H 6 O 2 (methyl acetate, 6.1%), C 3 H 6 O (acetone, 5.8%), and C 5 H 4 O 2 (furfural, 5.5%). Figure 2a presents the mass defect plot of organic compounds grouped into different ion families from a representative experiment, including CH, CHO, CHN and CHON compounds. The volatilities, represented by the saturation vapor concentrations (log 10 ( C *) μg m −3 ), of all organic compounds were predicted as a function of elemental composition as determined by the Vocus and Dual-EESI, using the parameterization by Li et al. 27 . Figure 2b displays the volatility distribution as a function of the carbon oxidation state (OSc, calculated as 2 × O/C – H/C ). Low-volatility compounds are predominantly in the CHO and CHON families, while high-volatility compounds fall into the CH family. The CHO group accounts for the largest fraction of the total primary organic gases (87.4% of the total mixing ratio), followed by the CH (7.5%), CHN (2.6%) and CHON (2.5%). CHO compounds are present across a large volatility range, with 14% assigned to the IVOCs and semi-volatile organic compounds (SVOCs, −0.5 μg m −3 < log 10 ( C *) < 2.5 μg m −3 ).
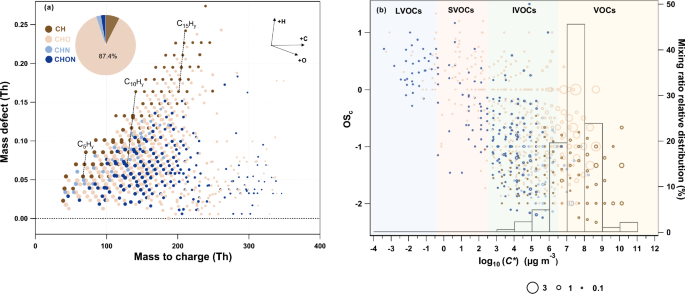
a Mass-defect plot of the ions identified by high-resolution analysis of the Vocus and Dual-EESI data sets. Data points are color-coded by ion family (CH, CHO, CHN, CHON) and sized by the logarithm of their concentration. b Two-dimensional volatility basis set (2D-VBS) of primary biomass burning products and volatility distribution. Compounds are plotted as carbon oxidation states (OS C , calculated as 2 × O/C – H/C ) versus estimated volatility (log 10 ( C *)). Markers are colored according to ion family and sized by the square root of their fractional contribution. Shaded areas indicate the volatility ranges of low-volatility (LVOC, log 10 ( C *) < −0.5 μg m −3 , orange), semi-volatile (SVOC, −0.5 μg m −3 < log 10 ( C *) < 2.5 μg m −3 , green), intermediate-volatility (IVOC, 2.5 μg m −3 < log 10 ( C *) < 6.5 μg m −3 , blue) and volatile organic compounds (VOC, log 10 ( C *) > 6.5 μg m −3 , yellow).
Water solubility of biomass burning organic vapors
After the characterization of the primary gas-phase BB emissions, Xe-excimer lamps were switched on to generate OH radicals (Supplementary Fig. 2 ) 28 . A slight decrease ( ~ 9.8%) in the concentrations of the total primary organic gases was observed due to their consumption by OH radicals, and a corresponding increase of oxygenated compounds was observed (Supplementary Fig. 5c ). Once a relatively steady state was reached, we simulated the presence of a cloud experimentally by introducing liquid water into the WFR, resulting in the formation of an aqueous microlayer on the reactor wall. A considerable decrease of the concentrations of some gas-phase species occurred, due to their uptake into the aqueous microlayer. Representative time series of species with different solubility are depicted in Supplementary Fig. 6 . The partitioning of gas-phase species into the aqueous phase depends on their bulk solubility. Non-soluble compounds (e.g., C 6 H 8 , 1,3-cyclohexadiene or fragments) remain unperturbed, while soluble compounds (e.g., C 4 H 6 O 4 ) experience a continuous and irreversible uptake. Moderately-soluble compounds (e.g., C 5 H 4 O 2 , furan-2-carbaldehyde) quickly reach their aqueous saturation concentration, and their mixing ratio in the gas phase recovers to their initial steady-state values.
EPI freeware v4.10 (EPI Suite, refer to the Supplementary Information for details on the EPI model) was used to estimate Henry’s law constants using the group contribution and bond contribution methods 29 , 30 , 31 , 32 . Figure 3a shows the Henry’s law constants of some species estimated by parameterizing modeled constants from the EPI Suite model (details of the species names are in Supplementary Table 3 ) and the net uptake ratio, which is the measured uptake ratio normalized by the maximum uptake ratio of 0.54 (due to diffusion limitations of the WFR, see Supplementary Information for details). To describe the partitioning of molecules with different solubility, we employed a kinetic model called QEMRA (see Supplementary Information for details). For organic gases at log 10 H below 2.5 M atm −1 , the uptake ratio is close to 0. It increases from 2.5 to 5.5 M atm −1 and becomes constant above 5.5 M atm −1 . The uptake coefficients of compounds against these Henry’s law constants follow the same behavior estimated by the QEMRA model, which gives confidence in the parameterized Henry’s law constants and suggests a minor deviation from ideality, expected given the diluted conditions in the water film representative of cloud water. In this work, the pH values of our solutions (pH = 4–5) are similar to those of cloud and fog samples 33 , 34 , 35 . Based on their solubility under our conditions, we categorized the organic gases into three classes: non-soluble gases (log 10 H ≤ 2.5 M atm −1 ), moderately-soluble gases (2.5 M atm −1 < log 10 H ≤ 5.5 M atm −1 ), and fully-soluble gases with irreversible uptake (log 10 H > 5.5 M atm −1 ). Estimated Henry’s law constants for all detected wood-burning gaseous species were calculated through a simplified parameterization of detected gaseous species and Supplementary Fig. 7 shows a comparison between EPI suite model log 10 H and parameterized log 10 H , highlighting the general agreement between the two approaches.

a Representative net uptake ratio (after 3 h of cloud processing) as a function of Henry’s law constants (log 10 H ). Grey markers represent uptake ratios calculated for oxidized organic gases measured by the Vocus vs. H values of individual molecules calculated by the EPI model (see details in the Supplementary Information). Black squares show the average net uptake ratio with vertical error bars as 1 standard deviation of the measured net uptake ratio. Also shown are the predicted aqueous-phase fractions using the QEMRA model for the measurements (red line, see details in ref. 23 ). b Relative mixing ratios of organic gases with different solubility as measured by the Vocus and Dual-EESI for the primary emissions, for the conditions in the WFR, thick cloud and thin cloud. The solubility is classified into three classes: non-soluble gases with log 10 H ≤ 2.5 M atm −1 , moderately-soluble gases with 2.5 M atm −1 < log 10 H ≤ 5.5 M atm −1 , and fully-soluble gases with log 10 H > 5.5 M atm −1 under WFR conditions.
By calculating the parameterized log H for all organic gases, we estimated that non-soluble and moderately-soluble gases make up 51.9% and 45% of the total mixing ratio of organic gases, respectively under WFR conditions. In our previous WFR study, we demonstrated through parameterization that the liquid water content (LWC) has a significant impact on the aqueous fraction of a given compound 23 . In Fig. 3b , we compare the relative mixing ratios of organic gases with different solubilities under WFR conditions to those in thick and thin clouds (1 g m −3 and 0.1 g m −3 ) 36 , 37 . The definition of the non-soluble, moderately-soluble and soluble gases under thick and thin cloud conditions depends on the curve in Fig. 3a . The fraction of non-soluble gases is higher for thick-cloud and thin-cloud conditions than in the WFR (79.6% and 80.6%, respectively, compared to 51.9%). Overall, 45% of the total NMOG mixing ratio is classified as moderately soluble under WFR conditions, whereas for thick-cloud conditions, it is expected to decrease to 19.3%. This indicates that the fraction of moderately-soluble compounds is to some extent overestimated in the WFR, while still being close to real ambient conditions. To achieve an even more representative simulation of the uptake, a thinner water film would be required. However, under such conditions, the aqSOA composition and yield cannot be determined due to experimental limitations.
aqSOA composition
The bulk composition of the aqSOA, as determined by the AMS, shows prominent signals of CHO + ( m/z 29), C 2 H 3 O + ( m/z 43), and CO 2 + ( m/z 44), oxygen-containing ions, which typically result from carbonyl and acid functional groups (Supplementary Fig. 8 ) 10 , 38 , 39 . Additionally, previous studies found that methylglyoxal and glyoxal from biomass burning are key precursors globally for secondary organic aerosol (SOA) formation 25 , 40 , 41 . Characteristic signals at C 2 H 2 O 2 + ( m/z 58), C 2 O 2 + ( m/z 56) and CH 2 O 2 + ( m/z 46) are observed, which have also been reported in aqSOA derived from methylglyoxal and glyoxal 42 , 43 . The aqSOA is characterized by a high oxygen-to-carbon ( O/C ) ratio of 0.9 ± 0.1. Such elevated O/C ratios for aqSOA are also observed in the field (e.g., San Pietro Capofiume and Beijing), indicating an increase in oxygenation as the aqSOA mass fraction increases 10 , 44 .
In addition to the bulk characterization by the AMS, the particle-phase EESI-TOF-MS (Particle-EESI) provides detailed molecular information about the aqSOA. We identified an increase in the number of ions above the detection limit with oxidation time. For the pure uptake experiment (without oxidation), 34 ions were detected, whereas, for the 1-hour and 3-hour aqueous phase oxidation experiments, 307 ions and 1275 ions were detected, respectively (Supplementary Fig. 9a ). After 3 h, the number of detectable ions remained roughly constant (excess oxidation may lead to fragmentation). To categorize the aqSOA molecular composition, we aggregated the data into three organic classes: CHO, CHN, and CHON. Among these, the CHO class was the largest, constituting 80% of the detected signal (Supplementary Fig. 9b ).
The complex oxidation processes can also be illustrated using the carbon oxidation state (OS C ) and the number of carbon atoms (#C) 45 . Supplementary Fig. 10 shows OS C vs. #C for different oxidation times and demonstrates that the abundance of highly oxygenated molecules with #C > 14 increases substantially with reaction time (from pure uptake to 3 h). The average carbon and oxygen distributions of aqSOA at different reaction times, as depicted in Supplementary Fig. 11 , exhibit considerable similarity (after 3 h). The double-bond equivalent (DBE) of aqSOA was calculated based on elemental formulae measured by the EESI-MS. The DBE for the aqSOA did not change any more substantially after 3 h of oxidation (with values between 4.5 and 4.7). These findings suggest that the composition of the aqSOA undergoes minimal changes beyond approximately 3 h of aqueous phase processing (Supplementary Fig. 11 ). Within the CHO compounds, aqueous-phase oxidation products of three representative phenols from BB were detected in the oxidation samples. The typical oxidation product of guaiacol dimers in the aqueous phase (e.g., C 14 H 14 O 4 , C 14 H 14 O 5 , C 14 H 14 O 6 , C 13 H 12 O 4 , C 13 H 12 O 6 ), and oxygenated ring-opening products (e.g., C 6 H 6 O 4 , C 7 H 10 O 6 ) were detected after three-hour aqueous processing. These species were also detected in the aqueous-phase oxidation of phenols with hydroxyl radical or triplet excited states of organic carbon in laboratory studies, as well as in cloud water samples and aqSOA from biomass burning in field campaigns 21 , 44 , 46 , 47 , 48 .
Figure 4 displays the molecular composition of aqSOA compared to the gaseous species dissolved in the WFR (determined as the difference in the gas-phase composition with and without the water microfilm). A wide range of ions with carbon numbers ranging from C4 to C23 is observed, with the highest signal attributed to compounds with C8-10 (Fig. 4a ). Our findings demonstrate that the composition of aqSOA is significantly more oxygenated and is dominated by molecules containing a greater number of carbon atoms compared to the dissolved gases (Fig. 4b ). The number of oxygen atoms (#O) per molecule in aqSOA species, ranging from 3 to 7, is considerably higher compared to the number of oxygen atoms in species dissolved into the WFR water microlayer. The increase in the oxygen content shows that the dissolution of oxidized organic vapors alone cannot explain the aqSOA production in terms of amount and composition. The disconnect between the dissolved gases and observed molecules also provides strong evidence that aqSOA results from the gas-phase water-soluble precursors that partition and react with OH to yield products with a much higher oxidation state, forming aqSOA. The top 50 aqSOA molecules, accounting for approximately 36.7%, consist mainly of oxidized monomers with four to six oxygen atoms. In contrast, the major dissolved gas molecules contain only one to three oxygen atoms.
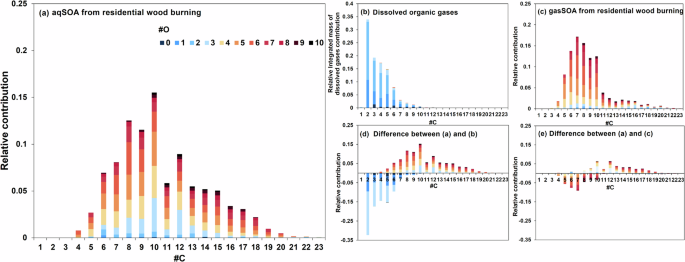
The average carbon number distribution ( x -axis) is colored by oxygen number. a AqSOA composition after 5 h of cloud processing measured by the Particle-EESI after nebulization, ( b ) Integrated dissolved gases (determined as the difference in the gas-phase composition with and without the water microfilm) measured by the Vocus and the Dual-EESI and calculated based on experimental data and the QEMRA model result from Fig. 2 , ( c ) GasSOA composition from gas-phase oxidation of the same wood burning experiment using an oxidation flow reactor (OFR) measured by the Particle-EESI. The aqueous and equivalent photochemical age for both ( a ) (aqSOA) and ( c ) (gasSOA) are ~5 days. The differences between aqSOA and integrated dissolved gas and gasSOA are shown in d and e , respectively (positive signal means aqSOA is higher).
Besides, aqSOA also has a larger contribution from larger carbon-containing species with substantially lower volatility (Supplementary Fig. 12 ). Homologous series of monomer (C ≤ 5) compounds account for the major dissolved gas fraction (53%), whereas the carbon number per molecule in aqSOA species (C ≥ 8, 85%) is substantially higher than in dissolved gas molecules, indicating that oligomerization plays an important role in the formation of aqSOA (Fig. 5a ). By using the nebulization, we cannot exclude that a fraction of the observed oligomers are formed during the aerosol drying process 49 , which also occurs in the ambient atmosphere.

a Carbon mass distribution and cumulative carbon mass of the oxidation products of aqSOA (light blue bars and light blue line, measured by the Particle-EESI), and of dissolved gases (dark blue bars and dark blue line, calculated based on the gas concentrations measured by the Vocus and the Dual-EESI and calculated from the QEMRA model after 5 h of experiment). b Measured aqSOA yields (red circles) compared to yields for SOA from gas phase oxidation (black squares). The aqueous time and photochemical age were calculated based on global average OH concentrations (1.5 × 10 6 molecules cm −3 ) and typical ambient conditions in cloud droplets. The error bars are the one standard deviation of the aqSOA yield calculated by two labeled isotopes. The yields were calculated as described in the Supplementary Information.
Mass spectral signatures of aqSOA from residential wood burning are characterized by an abundance of highly oxygenated and high molecular weight species. Additionally, we used an oxidation flow reactor (OFR) to investigate gasSOA formation from the same BB emissions after a simulation of approximately five days of photochemical aging 50 . In comparison to aqSOA, the dominant signals in gasSOA are attributed to C 6-8 H x O 3-7 compounds, while more highly oxygenated and carbon-containing species are observed in aqSOA (Fig. 4c ), due to extensive oxidation in the gas phase and to oligomerization processes in the aqueous phase.
The resulting aqueous solutions from different oxidation experiments (1–9 h) were spiked with 5 ppm solutions of isotopically labeled ammonium sulfate (NH 4 ) 2 34 SO 4 and ammonium nitrate NH 4 15 NO 3 , and used for quantification of the organic concentration, resulting in several micrograms per cubic meter of OA as measured by the AMS. The normalized integrated aqSOA mass (aqSOA mass divided by total NMOG concentration) increases with the increase of the aqueous phase processing time (Supplementary Fig. 13 , Supplementary Table 4 ). This suggested that the composition of aqSOA is not significantly dependent on the aging time (after 3 h), but the amount of aqSOA produced increases with aging. In this study, we assume the aqSOA yield by the ratio between the integrated aqSOA mass and the integrated mass of all NMOGs (including non-soluble gases) from primary emission that diffused to the WFR wetted wall (see the Supplementary Information). The aqSOA yield decreased from 0.43 (after 1 h) to 0.20 (after 9 h), as shown in Fig. 5b . This trend can be attributed to the solubility of the NMOGs in water, where fully soluble NMOGs continue to contribute to the formation of SOA, while moderately-soluble NMOGs mainly contribute to aqSOA during the initial minutes. Non-soluble NMOGs have limited influence on aqSOA mass (Supplementary Fig. 6 ). This result will be helpful for SOA modeling and field campaign studies to estimate the formation of aqSOA if only the total NMOGs from biomass burning are available. Besides, using the same method, we also estimated the average yield of gasSOA from wood burning using the OFR, which reached a maximum of approximately 0.17, lower than that of aqSOA.
The large contribution of BB-derived cloud chemistry to aqueous secondary organic aerosol, as highlighted in this study, has important implications for radiative forcing and regional air quality. While our experiment focused on residential wood burning, it is important to acknowledge that aqueous SOA formation will also occur in wildfire plumes, influencing regional climates. By experimentally simulating cloud processing, we demonstrate that the OH-radical-initiated oxidation and oligomerization of biomass burning emissions in the aqueous phase results in significant aqSOA formation, with a yield of 0.2–0.43, higher than the maximum gas-phase SOA yields (0.17). Such low gas-phase SOA yield implies that more than 80% of the oxidation products remain in the gas phase. As these oxidation products are more oxygenated than their precursors, they can potentially form additional aqSOA through their irreversible partitioning and further oxidation in the aqueous phase. The solubility and aqueous phase reactivity of oxidized biomass smoke vapors warrant further future investigations.
To date, some studies have observed that reactions of biomass burning emissions occur in the atmospheric liquid phase from regions including the Po Valley 10 , 51 and China 12 , 52 , 53 , representing a significant source of SOA mass. However, the detailed aqSOA from field studies is far from enough and the only time aqSOA has been reported with molecular information is during the Chinese haze using an EESI. In this study, Tong et al. 12 found that under high-NO x and RH conditions aqueous-phase chemistry can contribute substantially to SOA formation, accounting for 53.7% of the total SOA in Beijing. As shown in Supplementary Fig. 14b , the ambient aqSOA in Beijing exhibited a high proportion of O3-6 molecules and an overall enhanced signal from ions with highly oxygenated low carbon ions (e.g., C 6 H 6 O 5 ) relative to the other oxygenated organic aerosol. The highest yields of aqSOA were found to be associated with limited atmospheric aging (1 day) and highlight the large aqSOA potential close to emission sources during haze-like conditions with high relative humidity. Although a smaller fraction of soluble gases partitions under fog or haze conditions due to the relatively lower LWC (10 −3 -10 −1 g m −3 ) compared to clouds 54 , 55 , these soluble gases will still react in the liquid phase and form aqSOA. However, due to the more complex condition and source for Chinese haze uncertainty compared to our WFR condition (LWC, OH reactivity and potential other chemical pathways) 56 , 57 , more evidence of aqSOA chemical composition from the field is needed and more haze-relevant experiments should be designed for the lab to do a further comparison.
Extrapolating our results to the other residential wood-burning experiments we did using an OFR or smog chamber, we estimate that the contribution of aqSOA is 2–7 times higher than the contribution of primary biomass-burning organic aerosol (BBOA) emissions, depending on the emission factors of the primary organic gases and BBOA. This result is similar to the ambient atmosphere during haze formation, with the contribution of aqSOA surpassing that of BBOA by a factor of 2–4 12 , 52 , 53 . Our simulation experiments suggest that aqueous processing of biomass burning vapors can be one of the important sources of observed aqSOA during haze conditions.
In addition to residential wood burning, it is crucial to note that wildfires or prescribed open burning have become more frequent in many regions due to heatwaves and droughts 58 , 59 . Expanding upon our experiment’s focus on organic gases from primary residential wood burning, the WE-CAN aircraft campaign systematically characterized the western U.S. wildfire plumes and found that wildfires are also a major source of VOCs to the atmosphere, emitting hundreds to thousands of different organic gas-phase species 60 , 61 . Some of these organic gas-phase species will not only react with OH radicals in the gas phase but will also dissolve in cloud water, contributing to aqSOA formation. Therefore, further research is needed to investigate the solubility of organic vapors from wildfires and their resulting aqueous SOA yield.
In the atmosphere, organic gases from photochemical reactions in the ambient atmosphere are more oxidized than primary emissions. A higher oxidation state makes them more water soluble and they can partition even more than primary products into the aqueous phase, where their reactions can form additional aqSOA mass under higher RH conditions during the day, and primary emissions from biomass burning are the major aqSOA precursors at night 62 , 63 . Additionally, Wang et al. 63 found that gasSOA could also be transformed to aqSOA during daytime under higher NO x . Therefore, our results represent the first step toward a better representation of in-cloud aqSOA formation from residential wood burning while additional experiments beyond the conditions presented here are needed to investigate aqSOA formation (e.g., in the presence of extra UVB light, varied pH, NO x and temperature).
Wetted-wall flow reactor
An experimental simulation of aqSOA formation from residential wood burning emissions was conducted using a wetted-wall flow reactor (WFR) and a suite of mass spectrometers. In this study, an in-house built WFR operating in continuous flow mode was used to simulate the in-cloud formation and aging process 23 . The WFR consists of a quartz glass cylinder (length, 125 cm; internal diameter, 6 cm), a Xe-excimer laser, a humidifier, and a water injection system. The inner surface is sandblasted to increase the wettability of the glass. The cylinder rotates at a speed of 15 rotations per minute, maintaining an 85 µm water microlayer on the wall. A stirring bar (length = 123 cm, diameter = 3 mm) was placed at the bottom of the cylinder, facilitating the wetting of the wall and maintaining the microfilm. The Xe-excimer laser (7.2 eV, 172 nm) is used to induce photolysis of H 2 O and O 2 , resulting in the formation of OH radicals as well as O 3 and HO 2 molecules. All experiments were conducted with a stream of synthetic clean air (9.5–9.75 L min −1 ) generated by a zero air generator. This dry air stream was passed through a porous PTFE Gore-Tex tube (Gore™) immersed in ultrapure water to keep a constant RH of 95%–100%. The temperature of the WFR was measured with two thermocouples of type K, with one positioned at the inlet and the second at the outlet. RH was monitored with a hydroclip (Rotronic HygroClip). At the end of the WFR, there is a water injection system, which consists of a tube with one side in the quartz glass cylinder and another side connected to the injector. After each experiment, the WFR was cleaned three times by rotating it for 30 min with 500 mL of ultrapure water (18 MΩ cm). Subsequently, the WFR walls were then heated using a heat gun to 200 °C, while a pure dry air stream of 20 L min −1 was maintained for 1 h. Despite the higher LWC (6 × 10 −3 g mL −1 ) in the WFR compared to a cloud, our non-equilibrium system reflects well what will happen in the atmosphere.
Experimental setup
The experimental design is shown in Supplementary Fig. 2 . Before each burn and throughout the WFR experiments, a continuous stream of pure air was passed through the gas lines overnight to prevent cross-contamination between burns and to ensure a low background of VOCs. To simulate residential wood combustion, pine and spruce wood logs from a local forestry company in Würenlingen, Switzerland, weighing between 0.5 and 1.2 kg, were combusted in a modern woodstove (Avant, 2009, Attika). Emissions were sampled from the chimney through a heated line (473 K), diluted by a factor of approximately 10 using an ejector diluter (453 K, DI-1000, Dekati Ltd.) and injected into a holding tank (stainless steel, ~1 m 3 ) through a heated stainless-steel line (423 K). The holding tank ensured a constant concentration of NMOGs needed as input for the WFR experiments. The ejection diluter was operated with an input pressure of ~1.5 bar and the singular injecting time to the holding tank was 10–20 min. During the injection of the emissions into the holding tank, the concentrations of carbon monoxide (CO), carbon dioxide (CO 2 ), total hydrocarbon (THC), NO x and particles were measured in situ (see Supplementary Table 1 ). In most of the experiments, the modified combustion efficiency (MCE, defined as ΔCO 2 / (ΔCO + ΔCO 2 )) ranged from 0.9 to 0.95, indicating a flaming burning phase slightly mixed with a smoldering burning phase. Once the emission concentration in the holding tank reached a considerable level (THC concentrations > 15 ppmv C), the injection was halted and the organic gases from the holding tank were introduced into the WFR after passing through a particle filter (quartz) and mixing with the humidified air stream. The SVOC contribution in the gas phase is low 64 and the SVOCs in the particle phase are filtered and not considered in this study.
Experiments were performed at approximately 100% RH and a temperature of 290–295 K in the WFR. The humidified air stream was measured as the background. In short, this study comprises two distinct types of experiments. One is a pure uptake experiment (blank experiment), conducted with the Xe-excimer laser turned off and no generation of OH radicals. The first phase of this experiment was carried out with the Xe-excimer laser deactivated, meaning no oxidants were introduced, and it was referred to as the ‘primary emissions’ phase. Subsequently, with the Xe-excimer laser still deactivated, 20 mL water was injected into the WFR and rotated (15 rotations min −1 ) to maintain a water microlayer on the wall. After 1 h, the water mixture was collected and analyzed. This experiment is used to verify if the vapor uptake, in the absence of oxidants, leads to the formation of aerosol upon nebulization. The second type involves oxidation experiments lasting 1, 3, 5, 7, and 9 h. Initially, like the blank experiment, organic vapors were continuously injected from the holding tank into the WFR (‘primary emission’ phase with Xe-excimer off). Subsequently, the Xe-excimer laser was turned on to create the oxidants, denoted the ‘oxidation’ phase. The OH concentrations at steady-state conditions in the WFR were 3 × 10 −13 to 5 × 10 −12 M in the aqueous phase and 3 × 10 8 molecules cm −3 in the gas phase. Once the gas concentration in the WFR reached stability (approximately 15 min), 20 mL water was injected and rotated, while the Xe-excimer laser was turned on until the end of the experiment. At this point, the water mixture was collected and stored at 4 °C until chemical analysis, which was analyzed by AMS and EESI within 24 h.
Lee et al. 65 , observed the direct production of hydrogen peroxide and formaldehyde from biomass burning, and these species from biomass burning will contribute to odd-hydrogen radical production, thereby affecting the oxidizing capacity of the atmosphere. However, the results from the blank experiment showed that extremely few organic compounds dissolved into the water and formed aqSOA (Supplementary Figs. 9 , 10 ), suggesting a negligible impact of these compounds compared to the SOA formation in the presence of OH radicals.
Measurements
A dual-phase extractive electrospray ionization time-of-flight mass spectrometer (Dual-EESI) and a PTR-TOF-MS (PTR-MS for short, Ionicon, Series 8000) were used for the chemical characterization of the primary gas-phase compounds and their oxidation products emitted from the wood burning. In a subset of experiments, a Vocus PTR-TOF-MS (Vocus for short, TOFWERK, Aerodyne, Inc.) was also deployed to get a broader spectrum of VOCs, IVOCs and their oxygenated products (compared to the conventional PTR-MS). These additional measurements were used to determine the uptake ratio of gaseous products and the Henry’s law constant of individual molecules for the development of parameterization. Ozone concentrations were monitored using a commercial monitor (Monitor LabsInc., 8810). The uptake ratio was calculated as ( C no-cloud - C cloud )/ C no-cloud , where C no-cloud represents the concentration of organic gases after oxidation and C cloud represents the concentration of organic gases after e.g., 3 h of cloud simulation.
Offline analysis of aqueous-phase chemical composition was explored using two different instruments. A portion of the resulting aqueous solutions was nebulized in N 2 , using a customized Apex Q nebulizer (Elemental Scientific Inc., Omaha, USA) operating at 60 °C. The resulting droplets were dried using a Nafion dryer and analyzed using a high-resolution time-of-flight aerosol mass spectrometer (AMS, Aerodyne) and a Particle-EESI.
Data availability
The data used in this work are archived and can be downloaded from Zenodo ( https://doi.org/10.5281/zenodo.10630474 ).
Zhang, Q. et al. Ubiquity and dominance of oxygenated species in organic aerosols in anthropogenically-influenced Northern Hemisphere midlatitudes. Geophys. Res. Lett. 34 , 3 (2007).
Article Google Scholar
Hand, J. L. & Malm, W. C. Review of aerosol mass scattering efficiencies from ground-based measurements since 1990. J. Geophys. Res. Atmos. 112 , D16 (2007).
Pope, C. A. 3rd & Dockery, D. W. Health effects of fine particulate air pollution: lines that connect. J. Air Waste Manage. Assoc. 56 , 709–742 (2006).
Masson-Delmotte V et al. IPCC, 2021: Climate Change 2021: The Physical Science Basis. Contribution of Working Group I to the Sixth Assessment Report of the Intergovernmental Panel on Climate Change. (Cambridge University Press, 2021).
Hallquist, M. et al. The formation, properties and impact of secondary organic aerosol: current and emerging issues. Atmos. Chem. Phys. 9 , 5155–5236 (2009).
Article CAS Google Scholar
Bond, T. C. et al. Bounding the role of black carbon in the climate system: A scientific assessment. J. Geophys. Res. Atmos. 118 , 5380–5552 (2013).
Goldstein, A. H. & Galbally, I. E. Known and unexplored organic constituents in the earth’s atmosphere. Environ. Sci. Technol. 41 , 1514–1521 (2007).
Ervens, B., Sorooshian, A., Lim, Y. B. & Turpin, B. J. Key parameters controlling OH-initiated formation of secondary organic aerosol in the aqueous phase (aqSOA). J. Geophys. Res. Atmos. 119 , 3997–4016 (2014).
Sullivan, A. P. et al. Evidence for ambient dark aqueous SOA formation in the Po Valley, Italy. Atmos. Chem. Phys. 16 , 8095–8108 (2016).
Gilardoni, S. et al. Direct observation of aqueous secondary organic aerosol from biomass-burning emissions. Proc. Natl Acad. Sci. USA 113 , 10013–10018 (2016).
Faust, J. A., Wong, J. P., Lee, A. K. & Abbatt, J. P. Role of Aerosol Liquid Water in Secondary Organic Aerosol Formation from Volatile Organic Compounds. Environ. Sci. Technol. 51 , 1405–1413 (2017).
Tong, Y. et al. Quantification of solid fuel combustion and aqueous chemistry contributions to secondary organic aerosol during wintertime haze events in Beijing. Atmos. Chem. Phys. 21 , 9859–9886 (2021).
Olsen, Y. et al. Emissions and source allocation of carbonaceous air pollutants from wood stoves in developed countries: A review. Atmos. Pollut. Res. 11 , 234–251 (2020).
Hatch, L. E. et al. Identification and quantification of gaseous organic compounds emitted from biomass burning using two-dimensional gas chromatography–time-of-flight mass spectrometry. Atmos. Chem. Phys. 15 , 1865–1899 (2015).
Koss, A. R. et al. Non-methane organic gas emissions from biomass burning: identification, quantification, and emission factors from PTR-ToF during the FIREX 2016 laboratory experiment. Atmos. Chem. Phys. 18 , 3299–3319 (2018).
Selimovic, V. et al. Aerosol optical properties and trace gas emissions by PAX and OP-FTIR for laboratory-simulated western US wildfires during FIREX. Atmos. Chem. Phys. 18 , 2929–2948 (2018).
Sander, R. Compilation of Henry’s law constants (version 5.0.0) for water as solvent. Atmos. Chem. Phys. 23 , 10901–12440 (2023).
Tomaz, S. et al. Photochemical Cloud Processing of Primary Wildfire Emissions as a Potential Source of Secondary Organic Aerosol. Environ. Sci. Technol. 52 , 11027–11037 (2018).
Sun, Y. L., Zhang, Q., Anastasio, C. & Sun, J. Insights into secondary organic aerosol formed via aqueous-phase reactions of phenolic compounds based on high resolution mass spectrometry. Atmos. Chem. Phys. 10 , 4809–4822 (2010).
Zhao, R., Mungall, E. L., Lee, A. K. Y., Aljawhary, D. & Abbatt, J. P. D. Aqueous-phase photooxidation of levoglucosan – a mechanistic study using aerosol time-of-flight chemical ionization mass spectrometry (Aerosol ToF-CIMS). Atmos. Chem. Phys. 14 , 9695–9706 (2014).
Yu, L. et al. Molecular transformations of phenolic SOA during photochemical aging in the aqueous phase: competition among oligomerization, functionalization, and fragmentation. Atmos. Chem. Phys. 16 , 4511–4527 (2016).
Tan, Y., Lim, Y. B., Altieri, K. E., Seitzinger, S. P. & Turpin, B. J. Mechanisms leading to oligomers and SOA through aqueous photooxidation: insights from OH radical oxidation of acetic acid and methylglyoxal. Atmos. Chem. Phys. 12 , 801–813 (2012).
Lamkaddam, H. et al. Large contribution to secondary organic aerosol from isoprene cloud chemistry. Sci. Adv. 7 , eabe2952 (2021).
McDonald, J. D. et al. Fine particle and gaseous emission rates from residential wood combustion. Environ. Sci. Technol. 34 , 2080–2091 (2000).
Schauer, J. J., Kleeman, M. J., Cass, G. R. & Simoneit, B. R. Measurement of emissions from air pollution sources. 3. C1− C29 organic compounds from fireplace combustion of wood. Environ. Sci. Technol. 35 , 1716–1728 (2001).
Lee, C. P. et al. High-frequency gaseous and particulate chemical characterization using extractive electrospray ionization mass spectrometry (Dual-Phase-EESI-TOF). Atmos. Meas. Tech. 15 , 3747–3760 (2022).
Li, Y., Pöschl, U. & Shiraiwa, M. Molecular corridors and parameterizations of volatility in the chemical evolution of organic aerosols. Atmos. Chem. Phys. 16 , 3327–3344 (2016).
Molteni, U. et al. Formation of highly oxygenated organic molecules from aromatic compounds. Atmos. Chem. Phys. 18 , 1909–1921 (2018).
Chakka S., Boddu V. M. & Maloney S. W., Damavarapu R. Prediction of physicochemical properties of energetic materials via EPI Suite. Energ. Mater . 77–92 (2010).
Wu, Y. & Chang, V. W. C. The Effect of Surface Adsorption and Molecular Geometry on the Determination of Henry’s Law Constants for Fluorotelomer Alcohols. J. Chem. Eng. Data 56 , 3442–3448 (2011).
Kühne, R., Ebert, R.-U. & Schüürmann, G. Prediction of the temperature dependency of Henry’s law constant from chemical structure. Environ. Sci. Technol. 39 , 6705–6711 (2005).
Glüge J., Bogdal C., Scheringer M., Buser A. M. & Hungerbühler K. Calculation of Physicochemical Properties for Short- and Medium-Chain Chlorinated Paraffins. J. Phys. Chem. Ref . 42 , (2013).
Carlton, A. G. et al. Atmospheric oxalic acid and SOA production from glyoxal: Results of aqueous photooxidation experiments. Atmos. Environ. 41 , 7588–7602 (2007).
Amato, P. et al. Microbial population in cloud water at the Puy de Dôme: Implications for the chemistry of clouds. Atmos. Environ. 39 , 4143–4153 (2005).
Pye, H. O. et al. The acidity of atmospheric particles and clouds. Atmos. Chem. Phys. 20 , 4809–4888 (2020).
Zhao, C. et al. Toward understanding of differences in current cloud retrievals of ARM ground-based measurements. J. Geophys. Res. Atmos. 17 , D10 (2012).
Google Scholar
Ishizaka, Y., Kurahashi, Y. & Tsuruta, H. Microphysical properties of winter stratiform clouds over the southwest islands area in Japan. J. Meteorol. Soc. Jpn. Ser. II 73 , 1137–1151 (1995).
He, L. Y. et al. Characterization of high-resolution aerosol mass spectra of primary organic aerosol emissions from Chinese cooking and biomass burning. Atmos. Chem. Phys. 10 , 11535–11543 (2010).
Wang, J. et al. Aqueous production of secondary organic aerosol from fossil-fuel emissions in winter Beijing haze. Proc. Natl Acad. Sci. USA 118 , e2022179118 (2021).
Zarzana, K. J. et al. Primary emissions of glyoxal and methylglyoxal from laboratory measurements of open biomass burning. Atmos. Chem. Phys. 18 , 15451–15470 (2018).
Wang, T. et al. Direct emissions of particulate glyoxal and methylglyoxal from biomass burning and coal combustion. Sci. Total Environ. 862 , 160757 (2023).
Altieri, K. et al. Oligomers formed through in-cloud methylglyoxal reactions: Chemical composition, properties, and mechanisms investigated by ultra-high resolution FT-ICR mass spectrometry. Atmos. Environ. 42 , 1476–1490 (2008).
Carlton, AG. et al. Atmospheric oxalic acid and SOA production from glyoxal: Results of aqueous photooxidation experiments. Atmos. Environ. 41 , 7588–7602 (2007).
Xiao, Y. et al. Aqueous secondary organic aerosol formation attributed to phenols from biomass burning. Sci. Total Environ. 847 , 157582 (2022).
Kroll, J. H. et al. Carbon oxidation state as a metric for describing the chemistry of atmospheric organic aerosol. Nat. Chem. 3 , 133–139 (2011).
Yee, L. D. et al. Secondary organic aerosol formation from biomass burning intermediates: phenol and methoxyphenols. Atmos. Chem. Phys. 13 , 8019–8043 (2013).
Brege, M. et al. Molecular insights on aging and aqueous-phase processing from ambient biomass burning emissions-influenced Po Valley fog and aerosol. Atmos. Chem. Phys. 18 , 13197–13214 (2018).
Cook, R. D. et al. Biogenic, urban, and wildfire influences on the molecular composition of dissolved organic compounds in cloud water. Atmos. Chem. Phys. 17 , 15167–15180 (2017).
De Haan, D. O., Tolbert, M. A. & Jimenez, J. L. Atmospheric condensed-phase reactions of glyoxal with methylamine. Geophys. Res. Lett. 36 , 11 (2009).
Li, K. et al. Uncovering the dominant contribution of intermediate volatility compounds in secondary organic aerosol formation from biomass-burning emissions. Nat. Sci. Rev. 11 , nwae014 (2024).
Paglione, M. et al. The impact of biomass burning and aqueous-phase processing on air quality: a multi-year source apportionment study in the Po Valley, Italy. Atmos. Chem. Phys. 20 , 1233–1254 (2020).
Zhong, H. et al. Enhanced formation of secondary organic aerosol from photochemical oxidation during the COVID-19 lockdown in a background site in Northwest China. Sci. Total Environ. 778 , 144947 (2021).
Zhao, J. et al. Organic Aerosol Processing During Winter Severe Haze Episodes in Beijing. J. Geophys. Res. Atmos. 124 , 10248–10263 (2019).
Guo, L., Guo, X., Fang, C. & Zhu, S. Observation analysis on characteristics of formation, evolution and transition of a long-lasting severe fog and haze episode in North China. Sci. China Earth Sci. 58 , 329–344 (2015).
Quan, J. et al. Analysis of the formation of fog and haze in North China Plain (NCP). Atmos. Chem. Phys. 11 , 8205–8214 (2011).
Tilgner, A. et al. Acidity and the multiphase chemistry of atmospheric aqueous particles and clouds. Atmos. Chem. Phys. 21 , 13483–13536 (2021).
Ervens, B., Turpin, B. J. & Weber, R. J. Secondary organic aerosol formation in cloud droplets and aqueous particles (aqSOA): a review of laboratory, field and model studies. Atmos. Chem. Phys. 11 , 11069–11102 (2011).
Weber, K. T. & Yadav, R. Spatiotemporal trends in wildfires across the Western United States (1950–2019). Remote Sens. 12 , 2959 (2020).
Park Williams, A. et al. Temperature as a potent driver of regional forest drought stress and tree mortality. Nat. Clim. Change. 3 , 292–297 (2013).
Permar, W. et al. Emissions of trace organic gases from Western US wildfires based on WE‐CAN aircraft measurements. J. Geophys. Res. Atmos. 126 , e2020JD033838 (2021).
Permar, W. et al. Assessing formic and acetic acid emissions and chemistry in western U.S. wildfire smoke: implications for atmospheric modeling. Environ. Sci.: Atmos. 3 , 1620–1641 (2023).
CAS Google Scholar
Li, Y. J., Huang, D. D., Cheung, H. Y., Lee, A. K. Y. & Chan, C. K. Aqueous-phase photochemical oxidation and direct photolysis of vanillin – a model compound of methoxy phenols from biomass burning. Atmos. Chem. Phys. 14 , 2871–2885 (2014).
Wang, F. et al. Investigation into the differences and relationships between gasSOA and aqSOA in winter haze pollution on Chongming Island, Shanghai, based on VOCs observation. Environ. Pollut. 316 , 120684 (2023).
Wang, T. et al. Organic vapors from wood, straw, cow dung, and coal burning using Vocus PTR-TOF. EGUsphere 2024 , 1–23 (2024).
Lee, M., Heikes, B. G., Jacob, D. J., Sachse, G. & Anderson, B. Hydrogen peroxide, organic hydroperoxide, and formaldehyde as primary pollutants from biomass burning. J. Geophys. Res. Atmos. 102 , 1301–1309 (1997).
Download references
Acknowledgements
This work was supported by the Swiss National Science Foundation (SNSF) (grant 200020_188624 and 200021_213071), the Swiss Agency For Development and Cooperation (project 7F-09802.01.03) the European Union’s Horizon 2020 research and innovation programme under the Marie Skłodowska-Curie grant agreement No 884104 (PSI-FELLOW-III-3i). We thank Pascal Schneider for the help in constructing the burning platform and other LAC group members for supporting the chamber experiments.
Author information
Present address: Environmental Research Institute, Shandong University, Qingdao, 266237, China
Authors and Affiliations
PSI Center for Energy and Environmental Sciences, Paul Scherrer Institute, 5232, Villigen, Switzerland
Tiantian Wang, Kun Li, David M. Bell, Jun Zhang, Tianqu Cui, Mihnea Surdu, Urs Baltensperger, Jay G. Slowik, Houssni Lamkaddam, Imad El Haddad & Andre S. H. Prevot
You can also search for this author in PubMed Google Scholar
Contributions
T.T.W., K.L., D.M.B., J.Z., and H.L. conducted the burning experiments. T.T.W. analyzed the data and wrote the manuscript. M.S. and T.Q.C. participated in the campaign. D.M.B., K.L., I.E.H., H.L., J.G.S., U.B. and A.S.H.P. participated in the interpretation of data.
Corresponding authors
Correspondence to Houssni Lamkaddam , Imad El Haddad or Andre S. H. Prevot .
Ethics declarations
Competing interests.
The authors declare no competing interests.
Additional information
Publisher’s note Springer Nature remains neutral with regard to jurisdictional claims in published maps and institutional affiliations.
Supplementary information
Supplementary information, rights and permissions.
Open Access This article is licensed under a Creative Commons Attribution 4.0 International License, which permits use, sharing, adaptation, distribution and reproduction in any medium or format, as long as you give appropriate credit to the original author(s) and the source, provide a link to the Creative Commons licence, and indicate if changes were made. The images or other third party material in this article are included in the article’s Creative Commons licence, unless indicated otherwise in a credit line to the material. If material is not included in the article’s Creative Commons licence and your intended use is not permitted by statutory regulation or exceeds the permitted use, you will need to obtain permission directly from the copyright holder. To view a copy of this licence, visit http://creativecommons.org/licenses/by/4.0/ .
Reprints and permissions
About this article
Cite this article.
Wang, T., Li, K., Bell, D.M. et al. Large contribution of in-cloud production of secondary organic aerosol from biomass burning emissions. npj Clim Atmos Sci 7 , 149 (2024). https://doi.org/10.1038/s41612-024-00682-6
Download citation
Received : 14 October 2023
Accepted : 05 June 2024
Published : 25 June 2024
DOI : https://doi.org/10.1038/s41612-024-00682-6
Share this article
Anyone you share the following link with will be able to read this content:
Sorry, a shareable link is not currently available for this article.
Provided by the Springer Nature SharedIt content-sharing initiative
Quick links
- Explore articles by subject
- Guide to authors
- Editorial policies
Sign up for the Nature Briefing: Anthropocene newsletter — what matters in anthropocene research, free to your inbox weekly.

Highly efficient degradation of tetracycline in groundwater by nanoscale zero-valent iron-copper bimetallic biochar: active [H] attack and direct electron transfer mechanism
- Research Article
- Published: 24 June 2024
Cite this article
- Lingling Zhang 1 ,
- Yujiao Wang 1 , 2 &
- Yin Xu ORCID: orcid.org/0000-0002-6055-297X 1 , 2
Development of carbon materials with high activity was important for rapid degradation of emerging pollutants. In this paper, a novel nanoscale zero-valent iron-copper bimetallic biochar (nZVIC-BC) was synthesized by carbothermal reduction of waste pine wood and copper-iron layered double hydroxides (LDHs). Characterization and analysis of its structural, elemental, crystalline, and compositional aspects using XRD, FT-IR, SEM, and TEM confirmed the successful preparation of nZVIC-BC and the high dispersion of Fe-Cu nanoparticles in an ordered carbon matrix. The experimental results showed that the catalytic activity of nZVIC-BC ( K obs of 0.0219 min −1 ) in the degradation of tetracycline (TC) in anoxic water environment was much higher than that of Fe-BC and Cu-BC; the effective degradation rate reached 85%. It was worth noting that the negative effects of Ca 2+ , Mg 2+ , and H 2 PO 4 − on TC degradation at ionic strengths greater than 15 mg/L were due to competition for active sites. Good stability and reusability were demonstrated in five consecutive cycle tests for low leaching of iron and copper. Combined with free radical quenching experiments and XPS analyses, the degradation of TC under air conditions was only 62%, with hydroxyl radicals (·OH) playing a dominant role. The synergistic interaction between Fe 2+ /Fe 3+ and Cu 0 /Cu + /Cu 2+ under nitrogen atmosphere enhances the redox cycling process; π-π adsorption, electron transfer processes, and active [H] were crucial for the degradation of TC; and possible degradation pathways of TC were deduced by LC-MS, which identified seven major aromatic degradation by-products. This study will provide new ideas and materials for the treatment of TC.
Graphical abstract
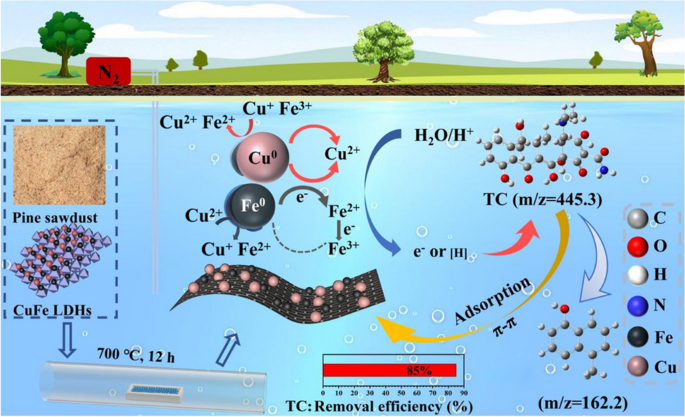
This is a preview of subscription content, log in via an institution to check access.
Access this article
Price includes VAT (Russian Federation)
Instant access to the full article PDF.
Rent this article via DeepDyve
Institutional subscriptions
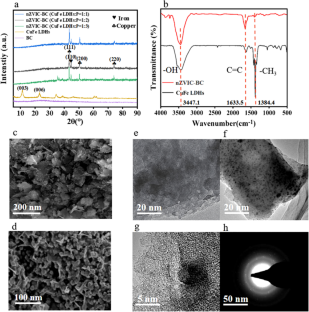
Data availability
All data generated or analyzed during this study are included in this published article.
Burke V, Richter D, Greskowiak J (2016) Occurrence of antibiotics in surface and groundwater of a drinking water catchment area in Germany. Water Environ Res 88:652–659
Article CAS Google Scholar
Che MD, Chen ZG, Qiu S (2021) High chloroform removal using tannic acid to promote the activation of persulfate with Fe/Ni nanoparticles. Environ Chem Lett 19:4015–4020
Che MD, Xiao JZ, Shan CC (2023) Efficient removal of chloroform from groundwater using activated percarbonate by cellulose nanofiber-supported Fe/Cu nanocomposites. Water Res 243:120420
Exall K, Balakrishnan VK, Toito J (2013) Impact of selected wastewater constituents on the removal of sulfonamide antibiotics via ultrafiltration and micellar enhanced ultrafiltration. Sci Total Environ 461-462:371–376
Fang G, Zhu C, Dionysiou DD (2015) Mechanism of hydroxyl radical generation from biochar suspensions: implications to diethyl phthalate degradation. Bioresour Technol 176:210–217
Gao FZ, Zou HY, Wu DL (2016) Swine farming elevated the proliferation of Acinetobacter with the prevalence of antibiotic resistance genes in the groundwater. Environ Int, 136, 105484.
Hua C, Ren, Dong (2011) Preparation of modified nanoscale zero-valent iron particles and its application in removal of tetracycline from wastewater. CJEE 5(4):767–771
Google Scholar
Kandel R, Jang SR, Shrestha S (2021) A bimetallic load-bearing bioceramics of TiO 2 @ ZrO 2 integrated polycaprolactone fibrous tissue construct exhibits anti bactericidal effect and induces osteogenesis in MC3T3-E1 cells. Mater Sci Eng C 131:112501
Kaur M, Umar A, Mehta SK (2018) Reduced graphene oxide-CdS heterostructure: an efficient fluorescent probe for the sensing of Ag(I) and sunset yellow and a visible-light responsive photocatalyst for the degradation of levofloxacin drug in aqueous phase. Appl Catal B-Environ 245:143–158
Article Google Scholar
Kim S, Eichhorn P, Jensen JN (2005) Removal of antibiotics in wastewater: effect of hydraulic and solid retention times on the fate of tetracycline in the activated sludge process. Environ Sci Technol 39:5816–5823
Kong L, Zhu Y, Liu M (2016) Conversion of Fe-rich waste sludge into nano-flake Fe-SC hybrid Fenton-like catalyst for degradation of AOII. Environ Pollut 216:568–574
Lee H, Lee HJ, Jeong J (2015) Activation of persulfates by carbon nanotubes: oxidation of organic compounds by nonradical mechanism. Chem Engi J 266:28–33
Lengliz S, Cheriet S, Raddaoui A (2022) Species distribution and genes encoding antimicrobial resistance in enterococcus spp. isolates from rabbits residing in diverse ecosystems: a new reservoir of linezolid and vancomycin resistance. J. Appl Microbiol 132(4):2760–2772
Li C, Wu J, Peng W (2019) Peroxymonosulfate activation for efficient sulfamethoxazole degradation by Fe 3 O 4 /β-FeOOH nanocomposites: coexistence of radical and non-radical reactions. Chem Eng J 356:904–914
Li L, Zhang Q, She Y (2021) High-efficiency degradation of bisphenol A by heterogeneous Mn–Fe layered double oxides through peroxymonosulfate activation: performance and synergetic mechanism. Separ Purif Technol 270:118770
Liu B, Liu Z, Yu P (2020) Enhanced removal of tris(2-chloroethyl) phosphate using a resin-based nanocomposite hydrated iron oxide through a Fenton-like process: capacity evaluation and pathways. Water Res 175:115655
Makowska N, Koczura R, Mokracka J (2016) Class 1 integrase, sulfonamide and tetracycline resistance genes in wastewater treatment plant and surface water. Chemosphere 144:1665–1673
Martins VV, Zanetti MOB, Pitondo-Silva A (2014) Aquatic environments polluted with antibiotics and heavy metals: a human health hazard. Sci Pollut Res 21:5873–5878
Meng X, Zhang C, Zhuang J (2020) Assessment of schwertmannite, jarosite and goethite as adsorbents for efficient adsorption of phenanthrene in water and the regeneration of spent adsorbents by heterogeneous fenton-like reaction. Chemosphere 244:125523
Peng XX, Wang ZN, Huang JF (2017) Efficient degradation of tetrabromobisphenol A by synergistic integration of Fe/Ni bimetallic catalysis and microbial acclimation. Water Res 122:471–480
Si Q, Guo W, Wang H (2021) Difunctional carbon quantum dots/g-C3N4 with in-plane electron buffer for intense tetracycline degradation under visible light: tight adsorption and smooth electron transfer. Appl Catal B-Environ 299:120694
Sunkara B, Zhan J, He J (2010) Nanoscale zerovalent iron supported on uniform carbon microspheres for the in situ remediation of chlorinated hydrocarbons. ACS Appl Mater Interfaces 2(10):2854–2862
Tian X, Jin H, Nie Y (2017) Heterogeneous Fenton-like degradation of ofloxacin over a wide pH range of 3.6 to 10.0 over modified mesoporous iron oxide. Chem Eng J 328:397–405
Wang A, Jiang Y, Yan Y (2023) Mechanistic and quantitative profiling of electro-Fenton process for wastewater treatment. Water Res 235:119838
Watkinson AJ, Murby EJ, Costanzo SD (2007) Removal of antibiotics in conventional and advanced wastewater treatment: implications for environmental discharge and wastewater recycling. Water Res 41(18):4164–4176
Wen Z, Zhang Y, Dai C (2015) Nanocasted synthesis of magnetic mesoporous iron cerium bimetal oxides (MMIC) as an efficient heterogeneous Fenton-like catalyst for oxidation of arsenite. J Hazard Mater 287:225–233
Weng X, Lin S, Zhong Y (2013) Chitosan stabilized bimetallic Fe/Ni nanoparticles used to remove mixed contaminants-amoxicillin and Cd (II) from aqueous solutions. Chem Eng J 229(22):27–34
Xu K, Ben W, Ling W (2017) Impact of humic acid on the degradation of levofloxacin by aqueous permanganate: kinetics and mechanism. Water Res 123:67–74
Xu X, Huang H, Zhang Y (2018) Biochar as both electron donor and electron shuttle for the reduction transformation of Cr(VI) during its sorption. Environ Pollut 244:423–430
Yang J, Jiangdongwang L, Wennaxie A, Jinsongyan Y (2019) Ultrahigh adsorption of tetracycline on willow branche-derived porous carbons with tunable pore structure: isotherm, kinetics, thermodynamic and new mechanism study. J Taiwan Inst Chem Eng 96:473–482
Yang Z, Lin Q, Zeng G (2023) Ternary hetero-structured BiOBr/Bi 2 MoO 6 @MXene composite membrane: construction and enhanced removal of antibiotics and dyes from water. J Membrane Sci 669:121329
Zhang L, Zeng G, Dong H, Chen Y, Zhang J, Yan M, Zhu Y, Yuan Y, Xie Y, Huang Z (2017) The impact of silver nanoparticles on the co-composting of sewage sludge and agricultural waste: evolutions of organic matter and nitrogen. Bioresour Technol 230:132–139
Zhang Z, Huang X, Ma J (2021) NEfficient removal of bisphenol S by non-radical activation of peroxydisulfate in the presence of nano-graphite. Water Res 201(8):117288
Zhao LX, Li MH, Jiang HL (2022) Activation of peroxymono sulfate by a stable Co-Mg-Al LDO heterogeneous catalyst for the efficient degradation of ofloxacin. Separ Purif Technol 294:121231
Zhao Z, Zhang G, Zhang Y (2020) Fe 3 O 4 accelerates tetracycline degradation during anaerobic digestion: synergistic role of adsorption and microbial metabolism. Water Res 185(1):116225
Zhuang Y, Jin L, Luthy RG (2012) Kinetics and pathways for the debromination of polybrominated diphenyl ethers by bimetallic and nanoscale zerovalent iron: effects of particle properties and catalyst. Chemosphere 89(4):426–432
Download references
This work was supported by the National Natural Science Foundation of China (52270079), Hunan Province Science and Technology Talents (2020TJ-N09), Chenzhou National Sustainable Development Agenda Innovation Demonstration Zone Innovation (2019sfq37), and Key Project of Hunan Provincial Education Department (21A0112).
Author information
Authors and affiliations.
Department of Environment, College of Environment and Resources, Xiangtan University, Xiangtan, Hunan, 411105, P. R. China
Lingling Zhang, Yujiao Wang & Yin Xu
Hunan Key Lab for Environmental Behavior of New Pollutants and Control Principle, Hunan, 411105, P. R. China
Yujiao Wang & Yin Xu
You can also search for this author in PubMed Google Scholar
Contributions
Lingling Zhang (Xiangtan, Hunan 411105, P. R. China): investigation, data curation, formal analysis, writing — original draft. Yujiao Wang (Xiangtan, Hunan 411105, P. R. China): formal analysis; writing — original draft; writing — review and editing; validation. Yin Xu (Xiangtan, Hunan 411105, P. R. China): conceptualization; methodology; writing — review and editing; funding acquisition.
Corresponding author
Correspondence to Yin Xu .
Ethics declarations
Ethical approval.
Not applicable.
Consent to participate
Consent for publication, competing interests.
The authors declare no competing interests.
Additional information
Responsible Editor: Weiming Zhang
Publisher’s Note
Springer Nature remains neutral with regard to jurisdictional claims in published maps and institutional affiliations.
Rights and permissions
Springer Nature or its licensor (e.g. a society or other partner) holds exclusive rights to this article under a publishing agreement with the author(s) or other rightsholder(s); author self-archiving of the accepted manuscript version of this article is solely governed by the terms of such publishing agreement and applicable law.
Reprints and permissions
About this article
Zhang, ., Wang, Y. & Xu, Y. Highly efficient degradation of tetracycline in groundwater by nanoscale zero-valent iron-copper bimetallic biochar: active [H] attack and direct electron transfer mechanism. Environ Sci Pollut Res (2024). https://doi.org/10.1007/s11356-024-33976-6
Download citation
Received : 23 March 2024
Accepted : 08 June 2024
Published : 24 June 2024
DOI : https://doi.org/10.1007/s11356-024-33976-6
Share this article
Anyone you share the following link with will be able to read this content:
Sorry, a shareable link is not currently available for this article.
Provided by the Springer Nature SharedIt content-sharing initiative
- Tetracycline
- Electronic transfer
- Find a journal
- Publish with us
- Track your research

IMAGES
VIDEO
COMMENTS
6. Balloon in a Bottle: Air Pressure Experiment. This is a simple experiment that shows how Air Pressure works. Objective: Kids learn how air and air pressure are able to expand a balloon and can have a great demonstration of air pressure. For more details about the balloon in a bottle: air pressure Browse Balloon in a Bottle: Air Pressure ...
Why it works: Blowing air between the balloons lowers the air pressure and makes the pressure surrounding them higher, pushing them together. Allison Sutcliffe. 4. Levitate Water. You won't need to incant Wingardium Leviosa with perfect pronunciation to suspend water during this exciting experiment.
Experiment with Air Quality Science Projects. (6 results) Measure pollutants in the air and learn about how gases in the atmosphere can cause the temperature to rise. Build your own tool to measure air quality, make a climate change model, or use a free online tool to analyze ozone levels. Air Particles and Air Quality.
Cut the carton into four flat pieces by cutting along the side seams of the carton. Cut each side into 3 square pieces, each piece will be approximately 3 inches long and 3 inches wide. You will have a total of 12 squares when you are done. Using the hole punch, punch a hole in one corner of each square.
First, you can look at the way pressure changes with the depth or height of a fluid. As we saw in Activity #3 above, the pressure in a fluid depends on how deep the fluid is. The deeper you are, the higher the pressure is. So, if you're standing in water, the pressure at your feet is higher than near your head.
In this aerodynamics science project, you will change the basic design of a paper plane and see how this affects its flight. Specifically, you will increase how much drag the plane experiences and see if this changes how far the paper plane flies. There is a lot of cool…. Read more. Make the Wind Work for You!
The balloon lung experiment is a fascinating demonstration that combines the principles of air pressure and the mechanics of the respiratory system. Similar Posts: 68 Best Chemistry Experiments: Learn About Chemical Reactions; 37 Water Science Experiments: Fun & Easy; Top 50 Fun Food Science Experiments
Leaky bottle demonstration (or per group if desired): Plastic water bottle with screw-top lid. Map/push pin. Plastic tray to catch excess water. Water to fill bottle. Main investigation (each group will need): 30 cm ruler. Two identical sheets of newspaper. Clear table top with a straight edge.
Cold water. Hot water. Refrigerator. EXPERIMENT STEPS. Step 1: Peel the labels off each water bottle so kids can see what is happening inside the bottles during the experiment. Step 2: Fill one water bottle with cool water. Step 3: Fill the second water bottle with hot water. Use hot pads, if needed, to avoid burning hands.
Egg into a Jar. Watch an egg magically drop into a jar. This happens because the air inside the jar is heated by the lit matches. After heating, the air starts to escape past the egg and as the air cools, the now higher pressure air on the outside forces the egg into the jar. Drop an egg into a bottle.
How to Conduct the Experiment. Follow these instructions to suck a water balloon into a jar using air pressure: Fill the balloon: Fill the water balloon until it's slightly wider than the neck of the jar and tie the balloon. Place the balloon on the jar: Place the jar on a flat surface and rest the balloon on top of the open jar.
Science has so many powerful phenomenons which can amaze anyone.We tired to explain the air pressure concept to our daughter using some amazing science exper...
Part 1. Inflate two balloons and tie them. Use string to tie a balloon on each end of the ruler. Tie a short string to the middle of the ruler so that you can hold the string to where the ruler is suspended. Move the balloons until the ruler is balanced. Make a prediction of what will happen if one balloon is popped. Record your prediction.
The force of air pressure against the card is stronger than the force of gravity on the water. The air pressure holds the card in place. In fact, the force of air pressure is so great that you can even use it to crush a can. Find out how with the next science project in science projects for kids: air pressure. For more fun science projects ...
Note: when conducting at-home science experiments with children, an adult should always be present. Even the simplest experiments have the potential to go wrong. The ordinary pressure of the air surrounding us is 14.7 pounds per square inch—but this can change based on a few factors, such as when the wind blows or a car or airplane accelerates.
Directions to do Air Pressure on Water Experiment. Step-1: Select a place where you feel free to do experiments with water like sinks or outdoors as this activity messy up things with water. Step-2: Now, pick an old and clear plastic bottle and make a hole of 10cm at the middle of the bottle.
Want to prove that air is a material that has mass and takes up space? Easy! Just try this experiment and you will see how the air prevents the water from re...
Air Resistance Experiment SUPPLIES: Printer/Computer Paper; Origami Science Book (optional for this activity); Other objects to explore air resistance (optional) All you need is a few sheets of paper, an open area, and our handy STEM activity printable sheet if you want to extend the lesson.
5 Fun Play-Based Science Activity Ideas for Kids - Air and More! ... This is NOT just another fact-focused science experiment about wind & friction! In this play-based, story-motivated activity, your children will lead their own learning in a way that keeps them engaged, and as an educator, you simply facilitate the process. ...
This air pressure activity is easy to do. Here are the steps to the second of our air pressure experiments: Cut a small length of straw. Plug one end of the straw with the play dough. On the opposite end of the straw, make a ring of play dough around the outside of the straw (so the straw is weighted but is open at that end) Fill the bottle ...
Blue Sky Test. The color of the sky is a reliable qualitative method to measure air quality. The color can change due to airborne particles that reflect and refract light. For example, a blue sky would indicate little to no air pollution whereas bright red ones are a result of heavy pollution. The University of Southern California developed an ...
The video explains what air is and properties of air. It shows many experiments to prove properties of air like air applies pressure, air has weight, air is ...
Publication date. 1774. Experiments and Observations on Different Kinds of Air (1774-86) is a six-volume work published by 18th-century British polymath Joseph Priestley which reports a series of his experiments on "airs" or gases, most notably his discovery of the oxygen gas (which he called "dephlogisticated air"). [1]
NASA's Perseverance rover viewed these dust devils swirling across the surface of Mars on July 20, 2021. Scientists want to study the air trapped in samples being collected in metal tubes by Perseverance. Those air samples could help them better understand the Martian atmosphere. Tucked away with each rock and soil sample collected by the ...
Apollo's air samples. In 2021, a group of planetary researchers, including scientists from NASA, studied the air brought back from the moon in a steel container by Apollo 17 astronauts some 50 ...
All experiments were conducted with a stream of synthetic clean air (9.5-9.75 L min −1) generated by a zero air generator. This dry air stream was passed through a porous PTFE Gore-Tex tube ...
Under an air atmosphere, the addition of pBQ and TBA led to a decrease in TC removal efficiency from 62 to 51% and 39%, respectively (Fig. 7a). This indicated that in an air atmosphere, the experiments confirmed the involvement of both ·OH and ·O 2 − in TC degradation, with ·OH playing a more significant role in TC degradation compared to ...