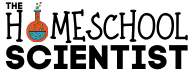

Convection Current Experiment
Sharing is caring!
Convection is one of three main types of heat transfer. The other two are radiation and conduction. Convection is the transfer of heat by the movement of heated particles into an area of cooler particles. You can experience convection when you light a match. The air directly above the lit match is always hotter than the air around the match.
This difference in temperature around the match is caused by the effect of heat on the density of air. Hot air is less dense than cool air and will rise leaving the cooler air below. As the warm air rises, a pattern of air movement is formed called a convection current. We can see these convection currents in the air and in water.
*This post contains affiliate links.
Related post – Weather Study Resources
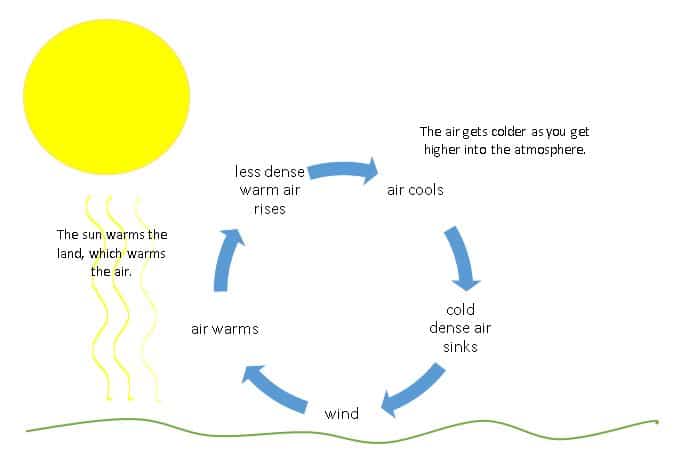
The Gulf Stream off the eastern coast of the States is a convection current. It carries warm water from the tropics up the east coast north toward the cold arctic waters.

Create Your Own Convection Current
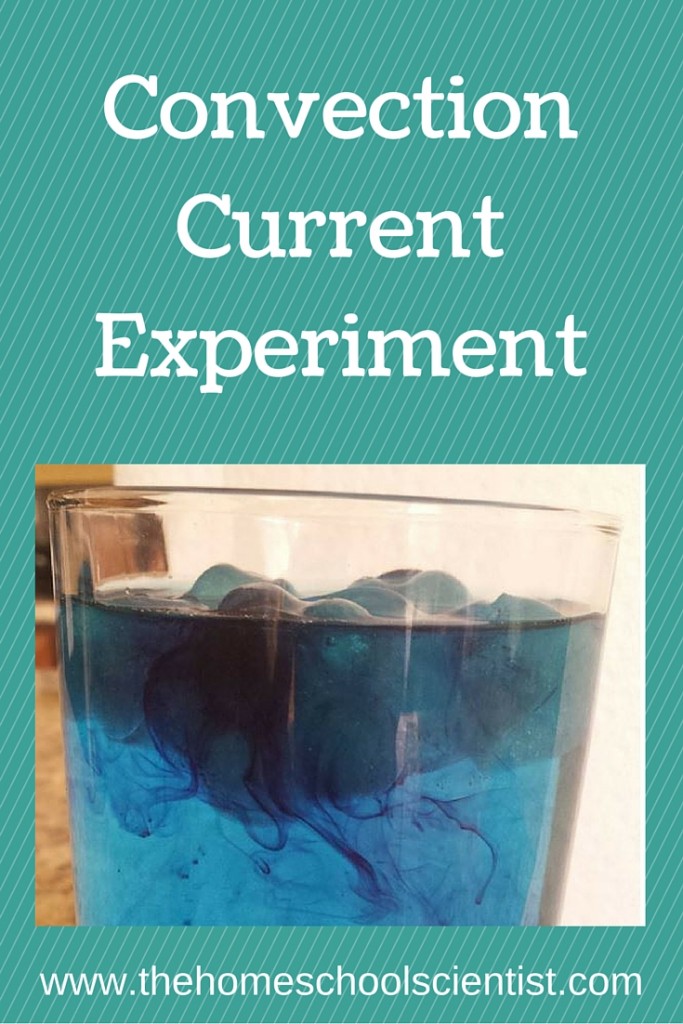
- ice cube tray
- food coloring
- clear drinking glass or jar
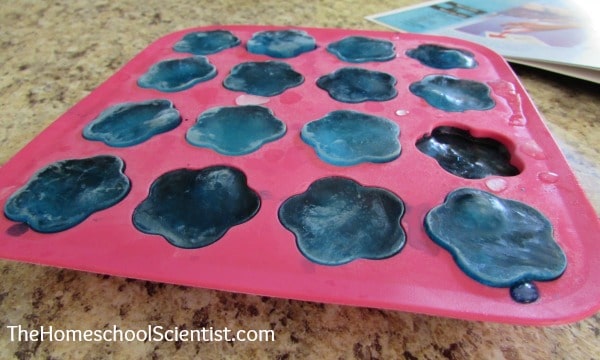
- Put the ice cube tray in the freezer until frozen solid.
- Fill a clear glass with warm water.
- Add one ice cube to the glass of water.
- Observe what happens.
What’s Happening?
The warm water will melt the ice cube, but the resulting water will be very cold. This cold, dense water will sink to the bottom of the glass. You can see this happening because the melted water from the ice cube will be whatever color you made your ice cube.
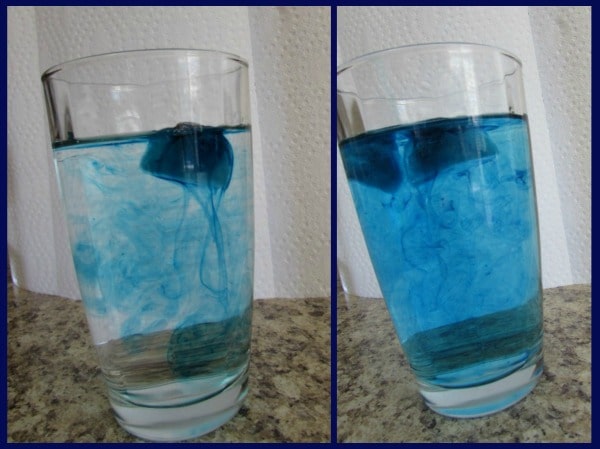
Try this simple experiment at home!
- Skip to primary navigation
- Skip to main content
- Skip to primary sidebar
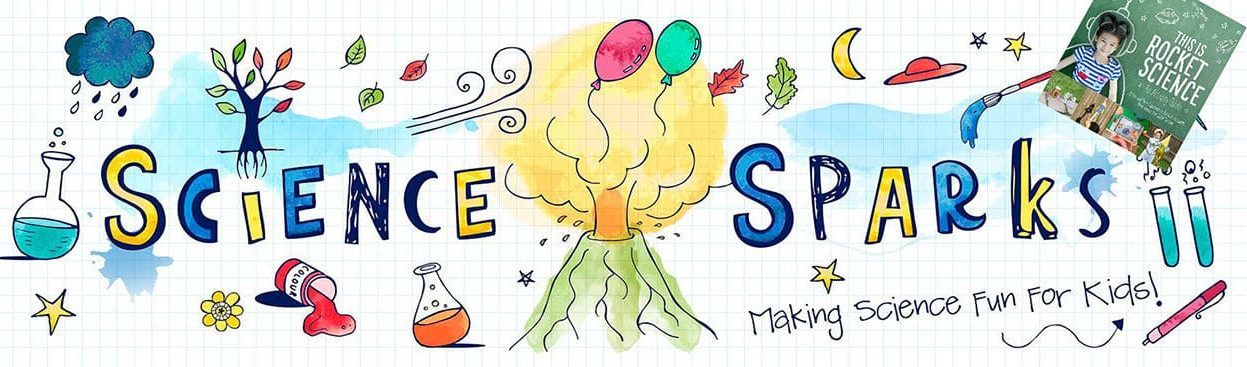
- FREE Experiments
- Kitchen Science
- Climate Change
- Egg Experiments
- Fairy Tale Science
- Edible Science
- Human Health
- Inspirational Women
- Forces and Motion
- Science Fair Projects
- STEM Challenges
- Science Sparks Books
- Contact Science Sparks
- Science Resources for Home and School
Convection Currents Made Easy
June 13, 2022 By Emma Vanstone Leave a Comment
When part of a liquid or gas is heated, it expands and becomes less dense. The warmer, less dense liquid rises upwards, and the cooler liquid falls to take its place. This cycle of a liquid or gas rising and falling is called a convection current .
We set up a very simple convection current demonstration using hot and cold water with food colouring to show the movement of warm water through cold water.
Convection Current Demonstration
You’ll need.
Tall glass or vase
A smaller glass or cup
Food colouring
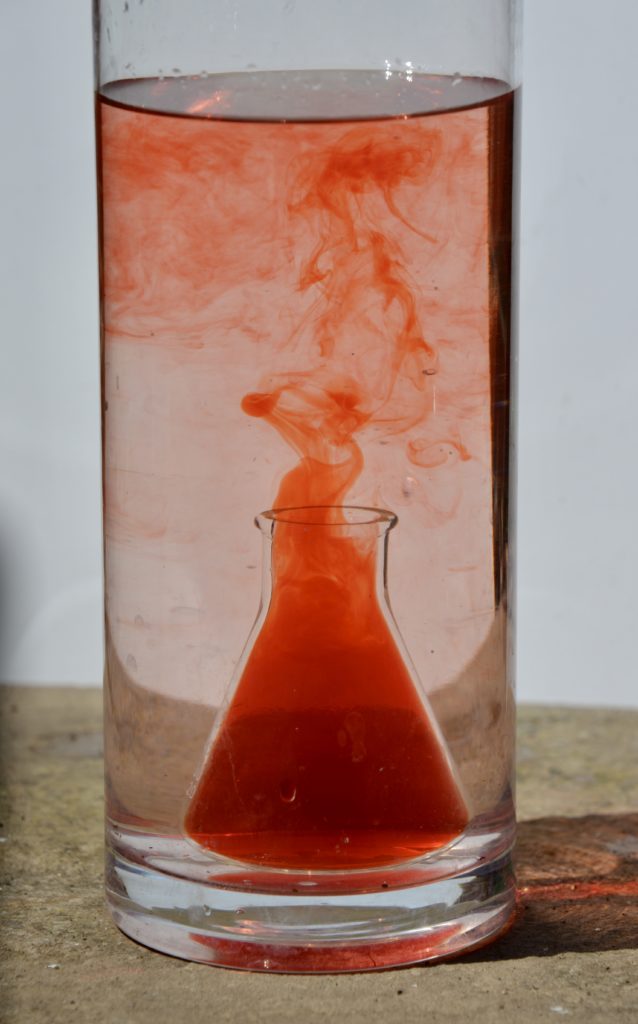
Instructions
Fill the tall glass or jar with cold water.
Fill the smaller container with hot ( but not boiling water ) and add a few drops of food colouring.
Carefully place the small container into the container with the cold water.
Watch what happens to the warmer, coloured water.
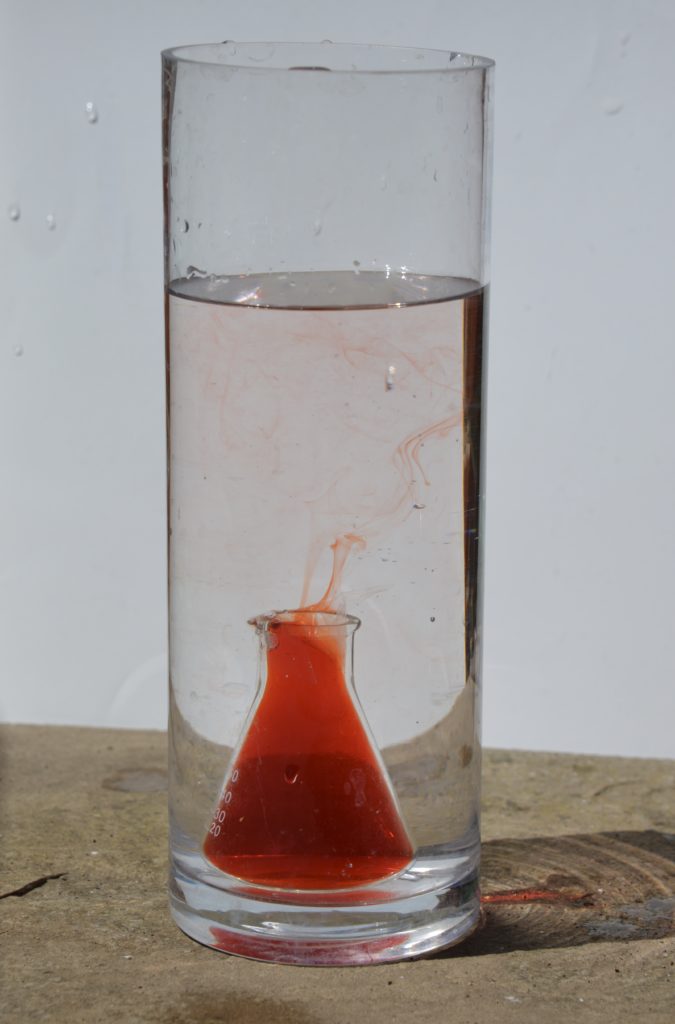
The hot, coloured water rises upwards and collects at the top of the cold water. It then cools and sinks downwards. Eventually, all the water will be at the same temperature.
How does convection work?
Particles of warm water move more quickly and spread out. They rise upwards through denser, cooler water, which sinks to the bottom, where it warms up. Eventually, all the liquid is at the same temperature.
Warm water rises because when liquids and gases are heated, they expand. This means they take up more room but have the same mass, so their density is less than when they are cool. Substances with lower densities float on substances with higher densities.
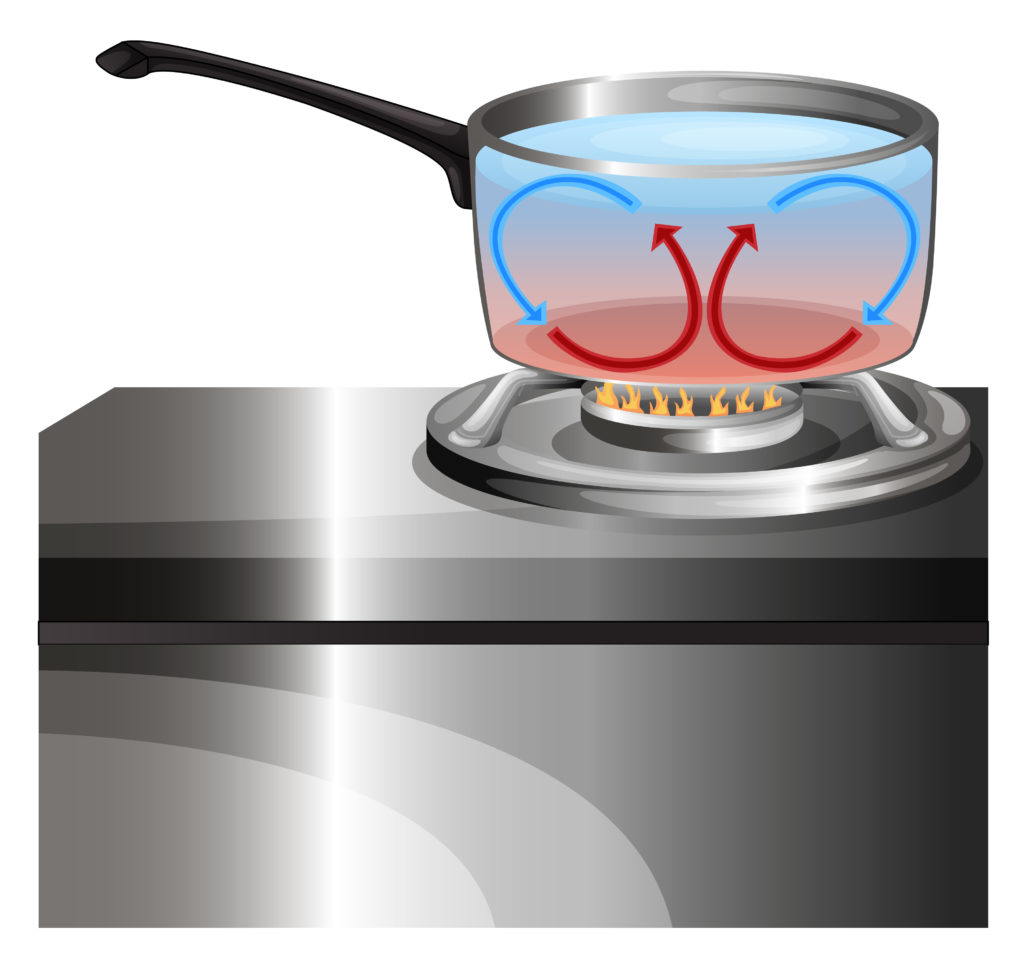
A hot air balloon is another example of convection currents in action.
Ask an adult to help with this activity
Extra Challenge
Repeat the activity with cold water instead of hot and watch what happens to the coloured water.
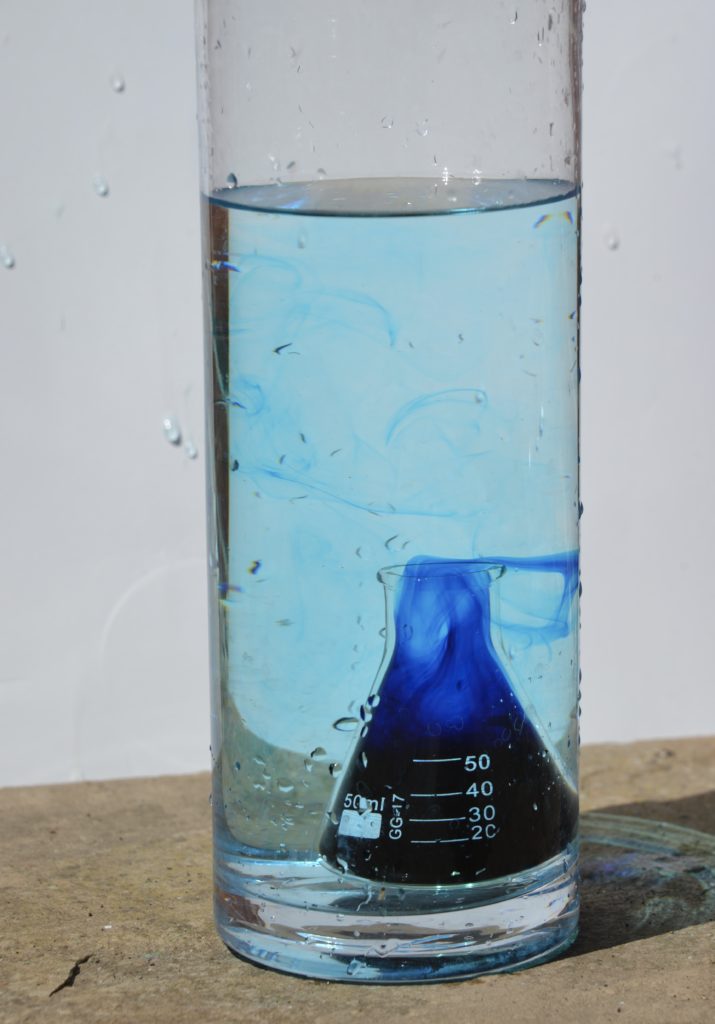
You can see that the cold water isn’t moving upwards like the warm water.
How is heat transferred?
Heat can be transferred in three different ways.
- Conduction.
- Radiation .
Heat convection occurs when warmer molecules of a liquid or gas move from a warmer to a cooler area, taking the heat with them.
Water being heated in a pan is an example of convection. This is the type of heat transfer we demonstrated above.
Another way to demonstrate convection is with a spinning convection snake .
Conduction of heat occurs when vibrating particles pass any extra vibrational energy to nearby particles. The more energy the particles have, the hotter the object gets. An example of this type of heat transfer is when metal pans are heated on a hob. Heat travels through the pan. If the pan handle is also metal, it will get hot, too. This is why metal pans often have plastic or wooden coverings on their handles. Plastic and wood are not good conductors of heat.
Radiation of heat is when heat is radiated to the surrounding area by heat waves. Particles are not involved in this kind of heat transfer.
The heat from the sun travelling through space is an example of heat transfer by radiation. Waves transfer this type of heat.
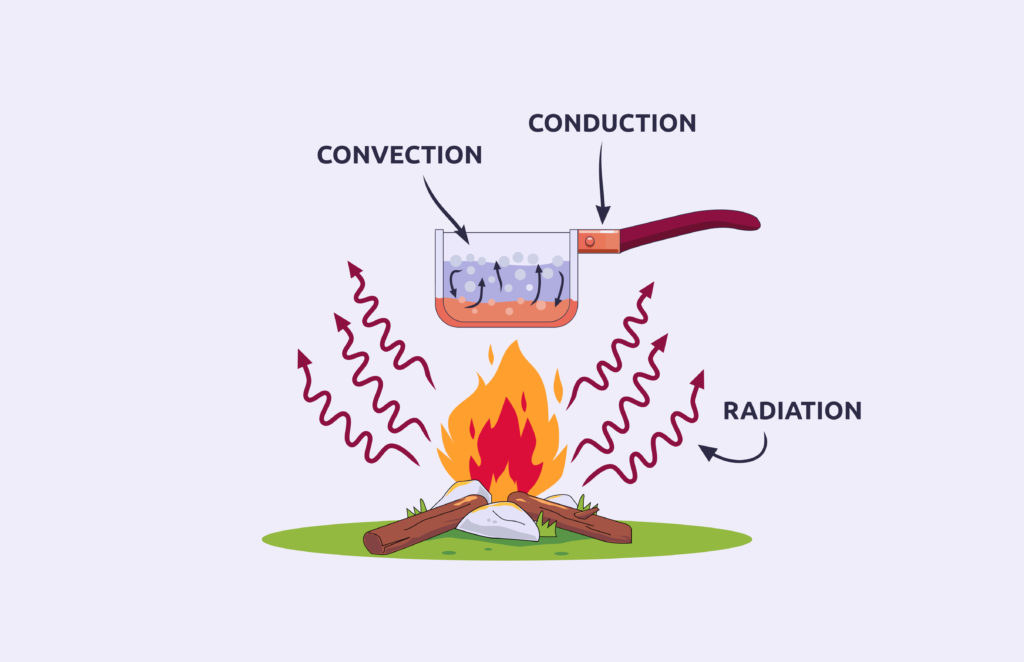
The campfire and pan example above shows all three kinds of heat transfer.
Heat travels by radiation from the campfire to the metal pan. Heat travels through the pan’s metal by conduction to warm the lower layers of water. The water is then heated by convection as the less dense warmer water rises through the cooler water, creating a convection current !
Remember – heat is only transferred if there is a temperature difference.
Science concepts
- Heat transfer
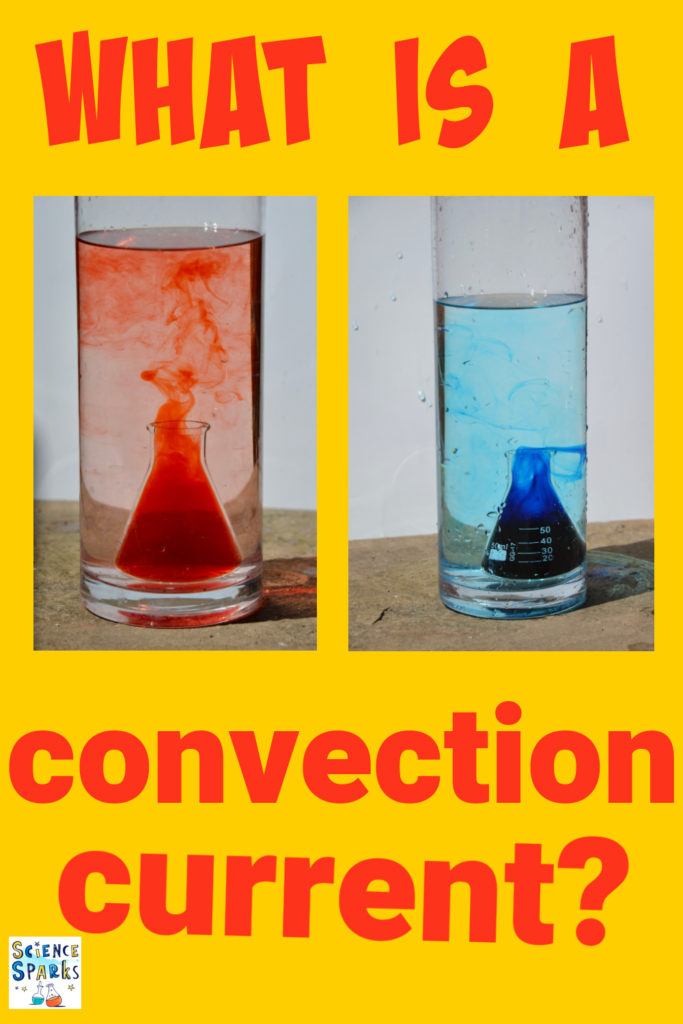
Last Updated on April 20, 2024 by Emma Vanstone
Safety Notice
Science Sparks ( Wild Sparks Enterprises Ltd ) are not liable for the actions of activity of any person who uses the information in this resource or in any of the suggested further resources. Science Sparks assume no liability with regard to injuries or damage to property that may occur as a result of using the information and carrying out the practical activities contained in this resource or in any of the suggested further resources.
These activities are designed to be carried out by children working with a parent, guardian or other appropriate adult. The adult involved is fully responsible for ensuring that the activities are carried out safely.
Reader Interactions
Leave a reply cancel reply.
Your email address will not be published. Required fields are marked *
Cool Science Experiments Headquarters
Making Science Fun, Easy to Teach and Exciting to Learn!
Science Experiments
Convection Science Experiment – How Heat Moves through Liquid
Can heat cause movement? With a few drops of food coloring, cooking oil, and a candle you can find out! In this simple yet exciting science experiment, kids can explore the concepts of density and convection as they watch convection currents in motion!
A demonstration video, printable instructions, and a supplies list are included as well as an easy to understand scientific explanation of how this experiment works.
Note: Because this experiment uses a fire component, adult supervision is required.
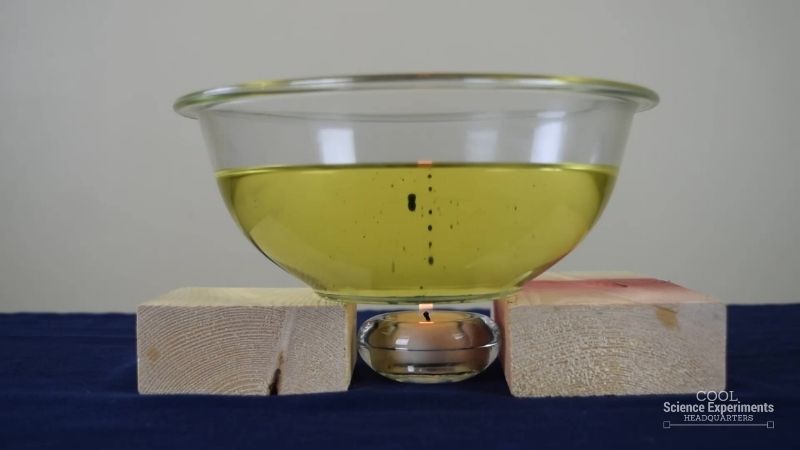
JUMP TO SECTION: Instructions | Video Tutorial | How it Works | Purchase Lab Kit
Supplies Needed
- Large heat safe glass bowl
- Cooking Oil
- Food Coloring
- Two 2×4 blocks
- Match or Lighter
Convection Science Lab Kit – Only $5
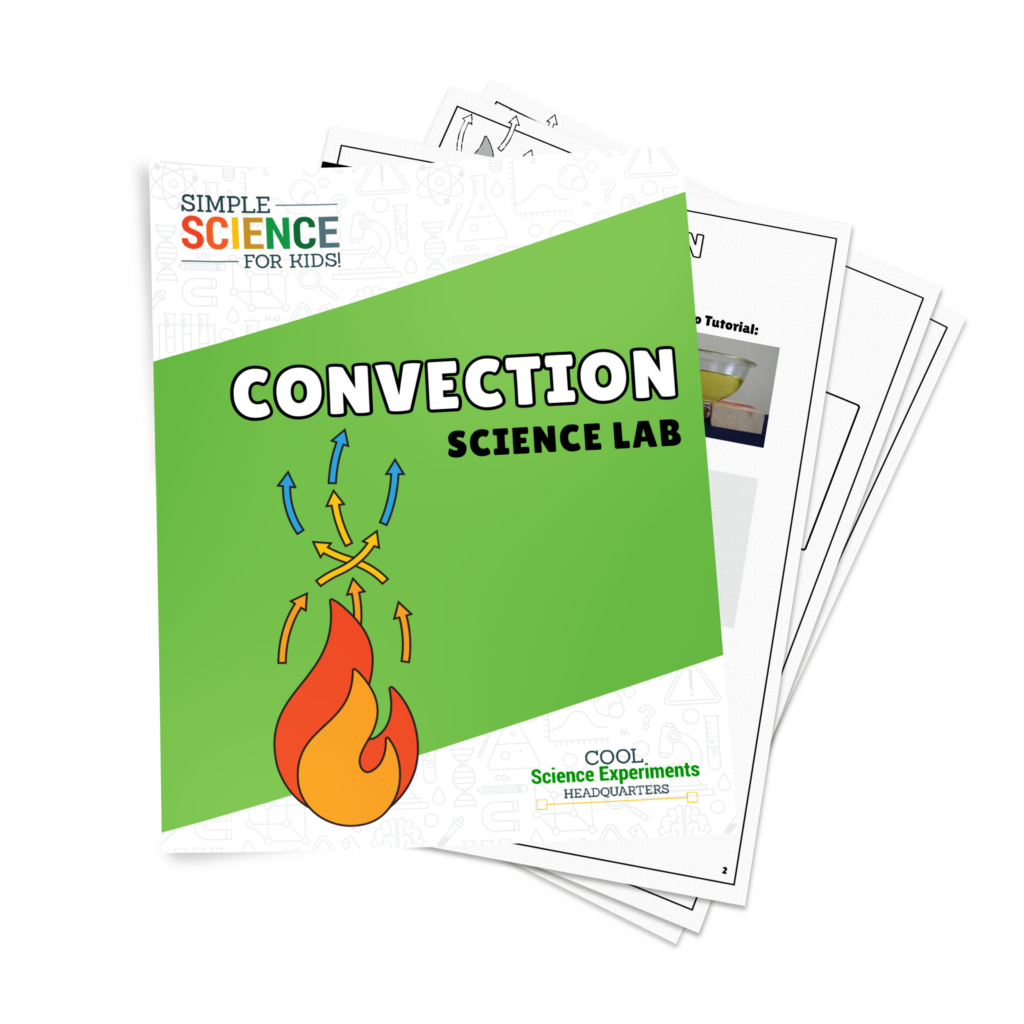
Use our easy Convection Science Lab Kit to grab your students’ attention without the stress of planning!
It’s everything you need to make science easy for teachers and fun for students — using inexpensive materials you probably already have in your storage closet!
Convection Science Experiment Instructions

Step 1 – Begin by filling a large glass bowl with cooking oil.

Step 2 – Next, add between 5-10 drops of food coloring into the oil. Take a moment to make some observations. What happens to the food coloring? Does it mix with the oil?
Helpful Tip: Place the drops near the center of the bowl.
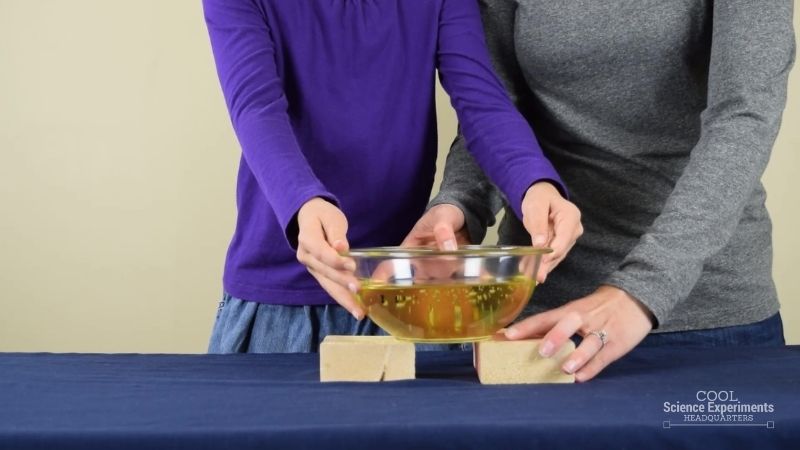
Step 3 – Prop the bowl up off the table using two 2×4 blocks. Position the blocks so there is a space between them to put a candle.
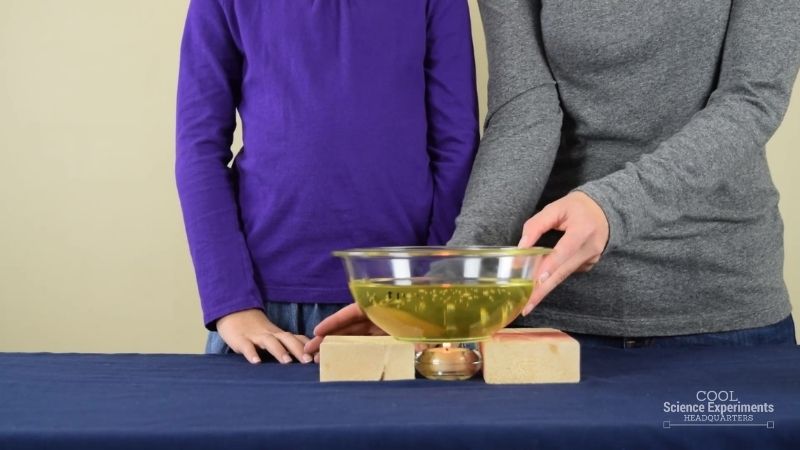
Step 4 – Light a candle and carefully place it under the bowl. The flame of the candle should touch the bottom of the glass bowl.
Step 5 – Look through the side of the glass bowl and watch carefully to observe what happens. Write down what happens. Helpful Tip: It will likely take 5 minutes before you see anything happen to the liquid/food coloring.
Do you know why the food coloring moves around in the oil? Find out the answer in the how does this experiment work section below.
Video Tutorial
How Does the Science Experiment Work
Heat can move in three ways: conduction, convection, and radiation. In this experiment, heat is transferred by means of convection. Convection is the transfer of heat by the movement of currents within a fluid.
In our experiment, the oil at the bottom of the bowl was heated by the candle. The particles of oil at the bottom of the pot began to move faster and further apart. As a result, these oil particles became less dense than the rest of the oil particles in the bowl, so these heated, less dense oil particles began to rise. (Less dense fluids rise and more dense fluids sink). The surrounding, cooler oil particles flow in to take its place. This flow creates a circular motion known as a convection current . A convection current is caused by the rising and sinking of heated and cooled fluids.
You can see evidence of the convection current if you look at the food coloring in the bowl. Notice bubbles of food coloring rise from the center of the bowl, drift to edges of the bowl, and sink back to the bottom.
Convection currents are all around us and responsible for heating many things! Our homes are heated in the winter through convection currents. The troposphere of the atmosphere (the layer closest to Earth) is heated through convection currents. The mantle inside of Earth is heated through convection currents, which causes Earth’s crust to drift in a process called continental drift.
I hope you enjoyed the experiment. Here are some printable instructions:
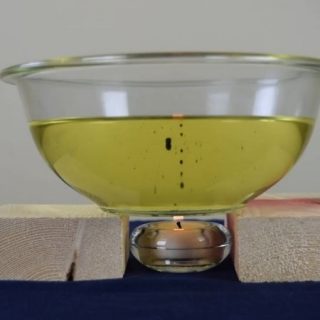
Convection Science Experiment
- Two 2×4 blocks
Instructions
- Begin by filling a large glass bowl with cooking oil.
- Next, add between 5-10 drops of food coloring into the oil. Helpful Tip: Place the drops near the center of the bowl.
- Prop the bowl up off the table using two 2×4 blocks.
- Light a candle and carefully place it under the bowl. The flame of the candle should touch the bottom of the glass bowl.
- Look through the side of the glass bowl and watch carefully to observe what happens. Helpful Tip: It will likely take 5 minutes before you see anything happen to the liquid/food coloring.
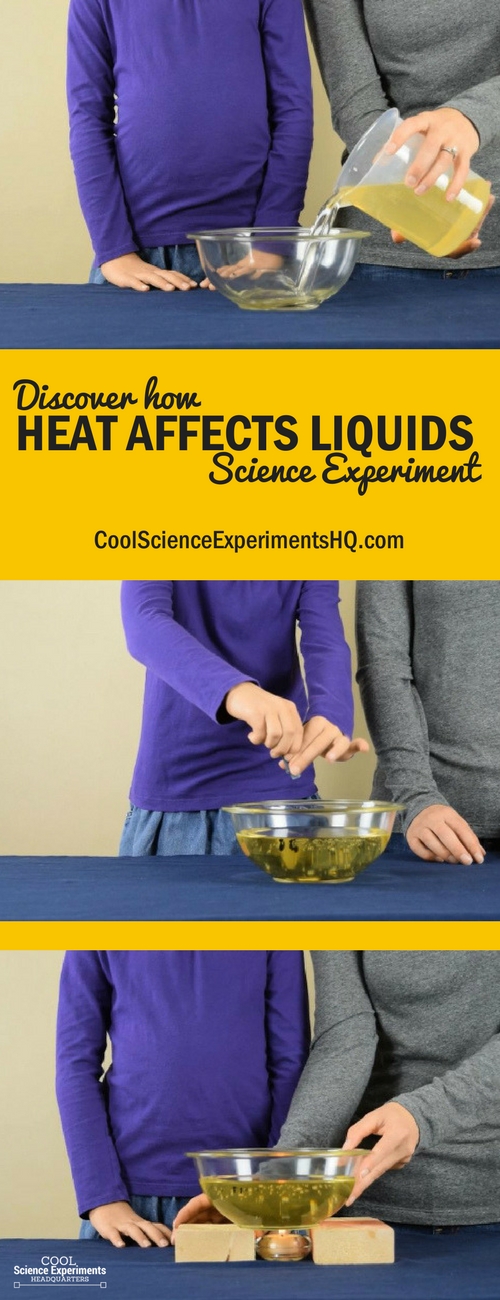
Reader Interactions
Leave a reply cancel reply.
Your email address will not be published. Required fields are marked *
Save my name, email, and website in this browser for the next time I comment.
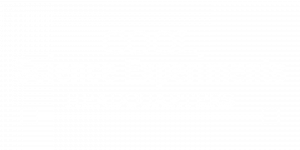
- Privacy Policy
- Disclosure Policy
Copyright © 2024 · Cool Science Experiments HQ
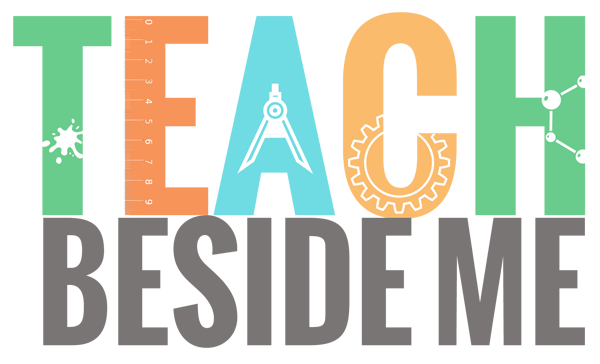
Colored Convection Currents Science Experiment
This post may contain affiliate links.
We are recently tried out a fun and simple experiment called colored convection currents. Have you heard of a convection current before?
Learn all about it here today! Then go and teach it to someone else. 🙂

What Are Convection Currents?
Convection is the movement within a liquid (or air) when the hotter and less dense liquid rises, and the colder, denser liquid sinks due to gravity. These movements create the circulation patterns in our atmosphere through air and water. The warm water or air rises and allows cooler air (or water) to go underneath.
This results in a transfer of heat. Heat can be transferred by convection when there is a big difference in temperature between the two different liquids.
The same thing happens in the movement of air. If you have ever watched the weather channel you can hear them talking about cool air and warm air moving across the earth. I am sure you also have heard that hot air rises? Well you can watch that happen in this experiment.

How to Do the Convection Current Experiment
If you take two bottles of water colored with food coloring , one with cold water and one with very warm water, you can create a current that is really fun to watch! The current will look completely different depending on whether the warm is on top or the cold is on top.

We tried both convection currents at the same time with four different bottles. I made the cold water blue and the warm water red- so you could see the heat rising and mixing. Place a notecard or playing card on top of the bottle that you are going to flip over to hold in the water. There may be a few drops of spilled water, so be prepared for that!

To see both currents at once, leave warm on the bottom for one and cold on the bottom for the other. Once the bottles are on top, pull out the card and you will see the water with the warm on the bottom quickly rise and mix into the cold water. For the one with the cold water on the bottom, it barely mixes!
Try this convection current experiment sometime . It is SO fun & easy. Experiment with different colors, too!
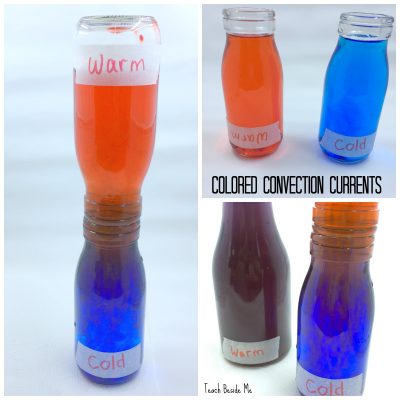
Try More Fun Science Experiments:
The Science of Air
States of Matter: Solids, Liquids and Gasses
Density Experiment
Former school teacher turned homeschool mom of 4 kids. Loves creating awesome hands-on creative learning ideas to make learning engaging and memorable for all kids!
Similar Posts
Rainbow woven lantern.

Winter Craft ~ Watercolor and Salt Snowflakes
Trail guide to learning curriculum, perler bead tic tac toe.

Doubles Math Puzzle Printable

Gravity Experiment: Gravity Spinner Toy
One comment.
This is very engaging and nice thanks for this
Leave a Reply Cancel reply
You must be logged in to post a comment.
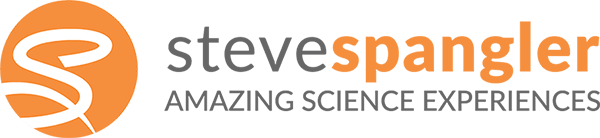
Colorful Convection Currents
Invisible convection currents are revealed using a very colorful demonstration.
Print this Experiment
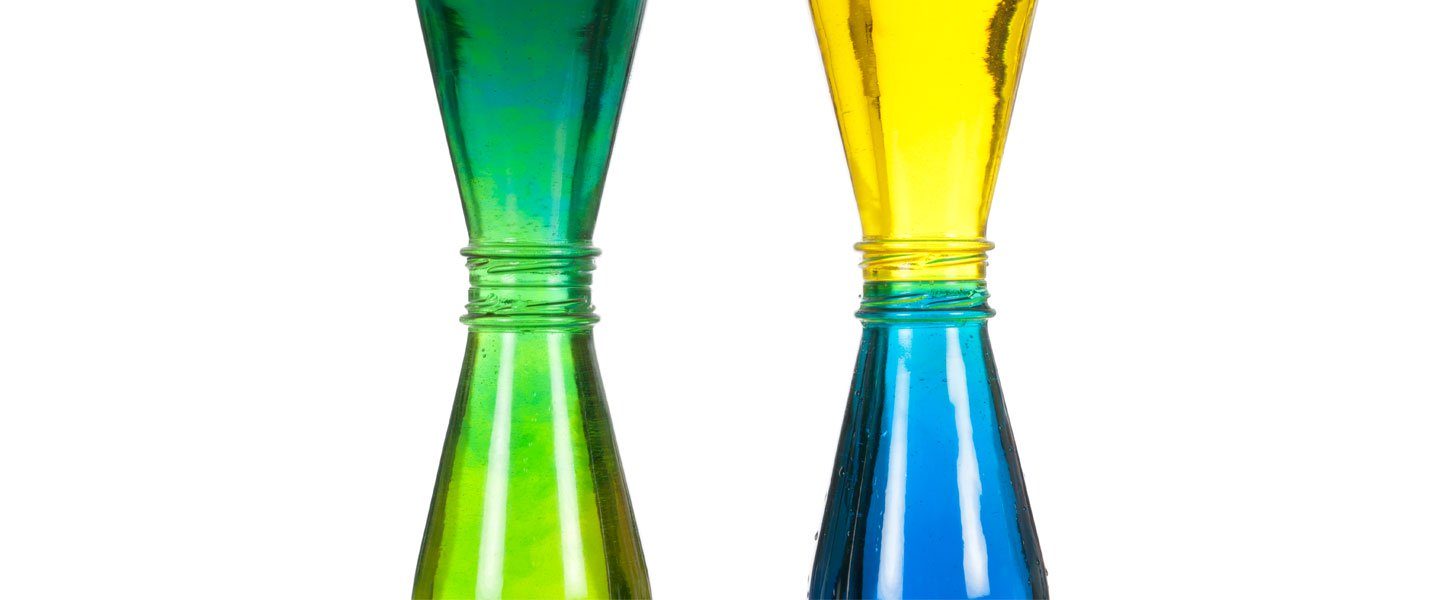
Cool, crisp, clean mountain air has long been an important reason why people move to Colorado, USA. Unfortunately, the air in the city of Denver isn’t quite as clean as it has been. By the 1970s, pollution over the city had a name—the “brown cloud.” Denver’s location at the foot of the Rocky Mountains makes it prone to temperature inversions in which warm air higher in the atmosphere traps cooler air near the ground. This condition prevents pollutants from escaping into the atmosphere. However, the phenomenon of temperature inversion is not unique to Denver, as evidenced by numerous reports of similar brown cloud sightings over major cities throughout the world. This demonstration provides a great illustration of what’s really happening in the atmosphere as hot and cold air masses meet.
Experiment Videos
Here's What You'll Need
Four empty, identical bottles (browse the juice aisle at a grocery store to find bottles similar to those shown. as a general rule, the opening of the bottle should be at least an inch [25 mm] in diameter.), access to hot and cold water, food coloring (yellow and blue) or fizzers coloring tablets, 3 x 5 inch (8 x 13 cm) card or an old playing card, masking tape, paper towels, adult supervision, let's try it.
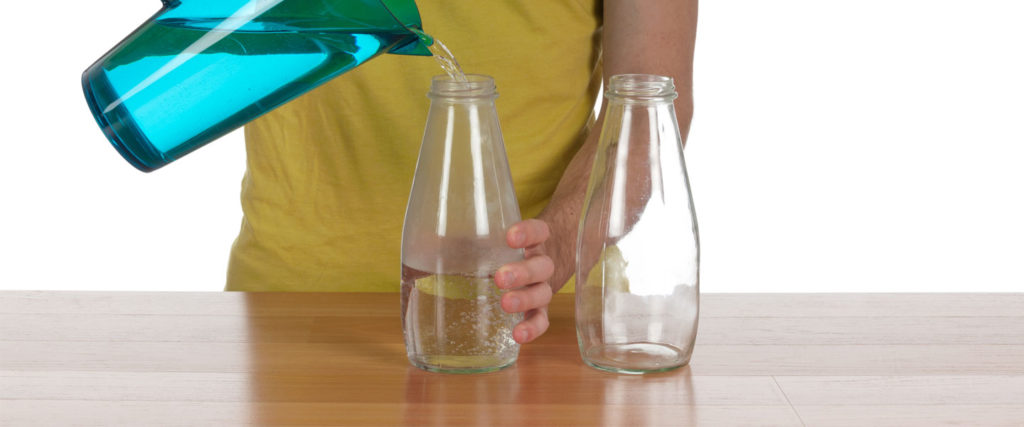
Fill two of the bottles with hot water from the tap and the other two bottles with cold water. Use masking tape and a pen to label the bottles “HOT” and “COLD.”
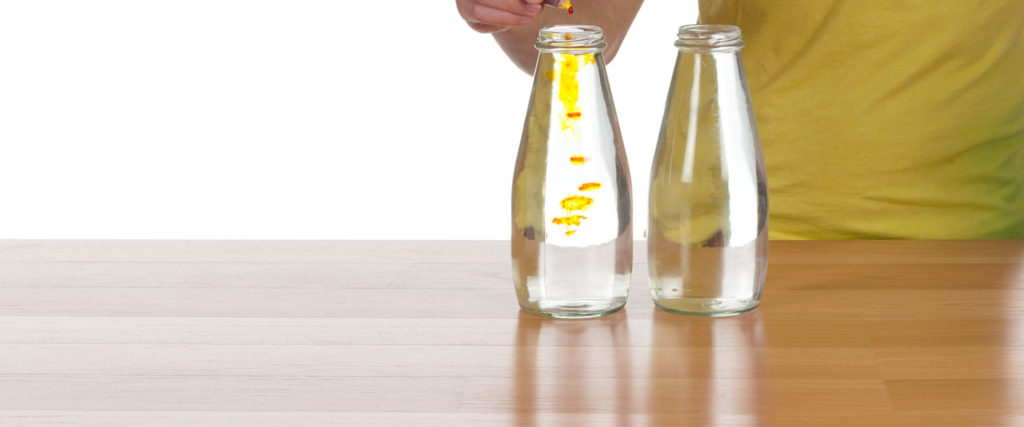
Use yellow food coloring in the warm water and blue food coloring in the cold water. Each bottle must be filled to the brim with water.
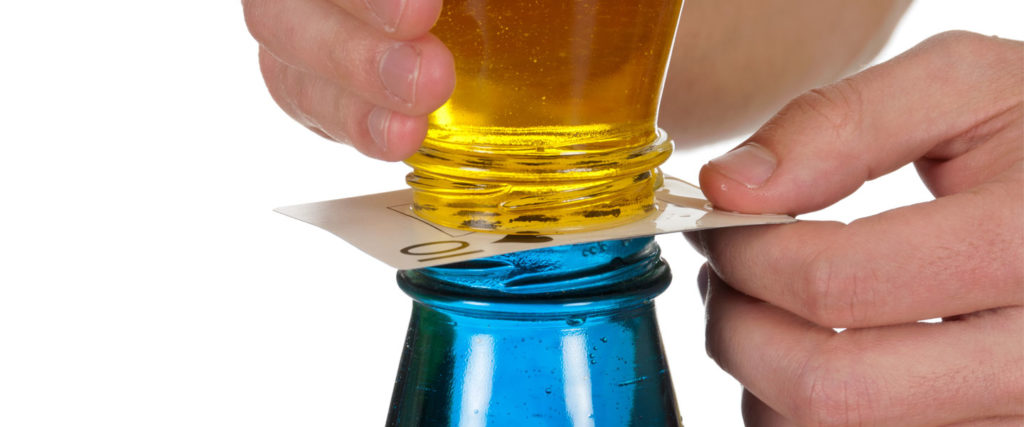
Your first observation will be what happens when the bottle filled with hot water sits on top of the bottle filled with cold water. To do this, place the card over the mouth of a hot water bottle (the yellow water). This can be a little tricky, but with practice you’ll get it. Hold the card in place as you turn the bottle upside down and place it on top of a cold water bottle (the blue water). The two bottles should be positioned so that they are mouth-to-mouth with the card separating the two liquids. (Have paper towels close by in case everything doesn’t go exactly as planned.)
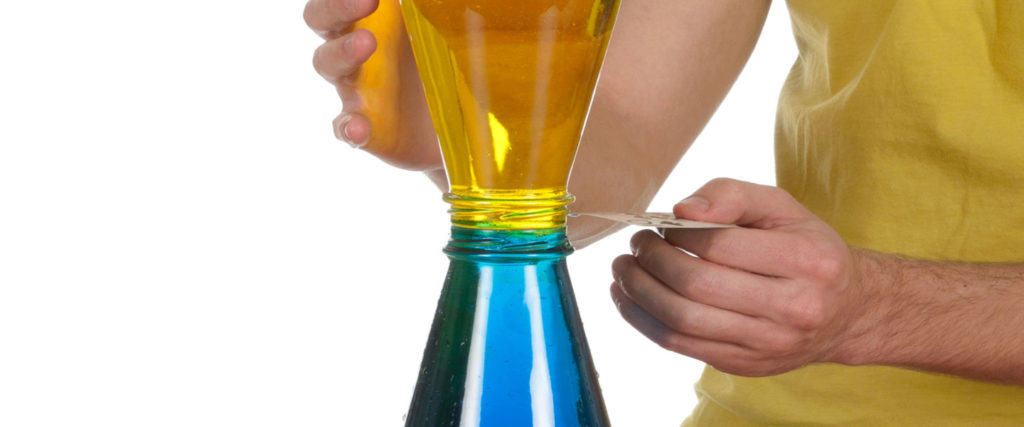
Carefully slide the card out from between the two bottles. Make sure you are holding the top bottle as you remove the card. Watch what happens to the colored liquids in the two bottles with the card removed.
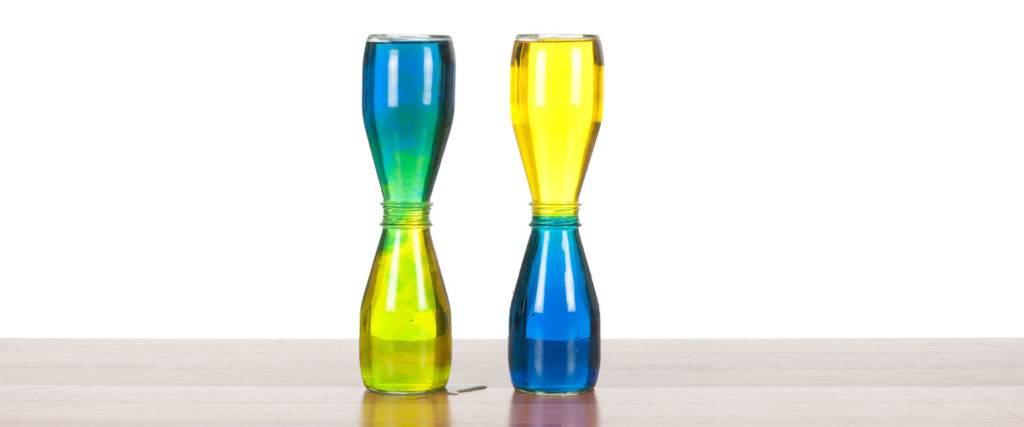
The second part of the experiment is similar to the first. This time, you need to place the cold water (blue) on top of the hot water (yellow). Repeat Steps 3 and 4 and carefully remove the card. Watch what happens this time.
How Does It Work
Hot air balloons rise because warm air in a balloon is lighter and less dense than cold air. Similarly, warm water is lighter or less dense than cold water. When the bottle of warm water is placed on top of the cold water, the more dense cold water stays in the bottom bottle and the less dense warm water is confined to the top bottle. However, when the cold water bottle sits on top of the warm water bottle, the less dense warm water rises into the top bottle and the cold water sinks into the bottom bottle. The movement of the water is clearly seen as the yellow and blue food colorings mix, creating a green liquid.
The movement of the warm and cold water inside the bottles is referred to as a convection current. In our daily life, warm currents of water occur in oceans. The warm Gulf Stream moves northward along the American Eastern Seaboard and crosses the Atlantic Ocean all the way to Europe. Convection currents in the atmosphere are responsible for the formation of thunderstorms as warm and cold air masses collide.
Although the colored liquids that mix are more interesting to watch, the other set of warm and cold water bottles helps to illustrate an important phenomenon that can occur in the atmosphere in cooler months. During daylight hours, the Sun heats the surface of the Earth and the layer of air closest to the Earth warms. This warm air rises and mixes with other atmospheric gases. When the Sun goes down, less dense warm air higher in the atmosphere blankets the colder air closer to the surface. Because the colder air is more dense than the warm air, the colder air is trapped close to the Earth and the atmospheric gases do not mix. This is referred to as a temperature inversion .
Real-World Application
What are the results of a temperature inversion in the atmosphere? Air pollution is much more noticeable during a temperature inversion since pollutants such as car exhaust and particulates are trapped in the layer of cool air close to the surface. As a result, state agencies in many parts of the country oxygenate automobile fuels during winter months with additives like MTBE in an attempt to reduce the harmful effects of trapped pollution. It is this trapped pollution that causes the brown cloud effect. Wind or precipitation can often alleviate the brown cloud by stirring and breaking up the layer of warm air that traps the cold air and pollution near the surface of the Earth.
Related Experiments
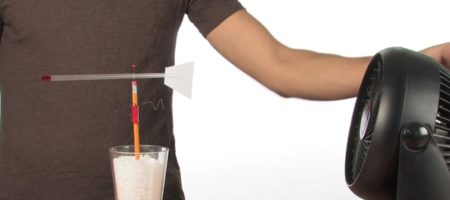
Do it Yourself Weather Vane
Early weathermen and women didn’t use Doppler radar or computers to forecast the weather, they relied on tools that were much more simple. Take, for […]
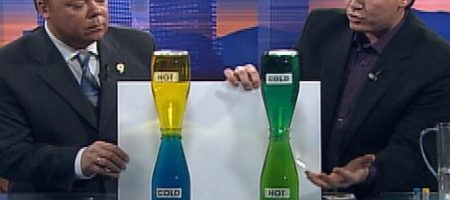
Denver's Brown Cloud
Cool, crisp, clean mountain air has long been an important reason why people move to Colorado. Unfortunately, the air in the city of Denver isn't […]
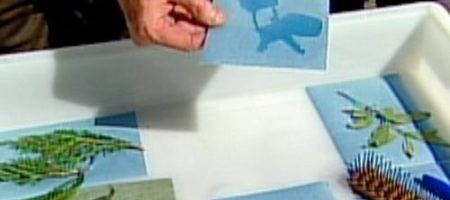
Nature Sun Sensitive Paper
Make amazing white on blue prints with your favorite objects! Just place leaves, flowers, shells, or whatever you choose on the photosensitive paper and place […]
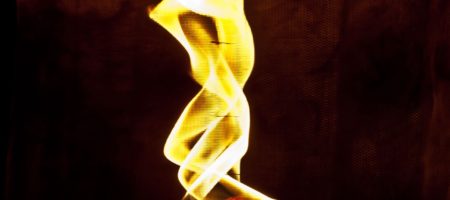
Fire Tornado
When we picture a tornado, most of us imagine a whirling column of air poking down from the clouds. But this tornado-like effect is not […]
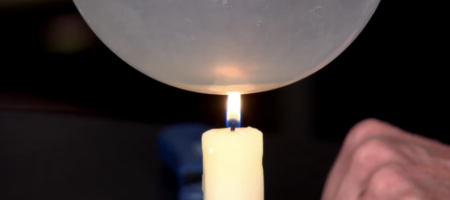
Fireproof Balloon
Common sense tells you that it’s impossible to boil water in a paper bag, but this classic parlor trick was a favorite of Victorian magicians. […]
Browse more experiments by concept:
Modeling How Air Moves
In this activity, students use models to observe that air is a fluid that flows due to temperature-driven density differences.
Learning Objectives
- Students will understand that air is a fluid and behaves in ways we expect of other fluids.
- Students will understand that temperature-driven density changes will produce movement in fluids.
- Students will understand that temperature differences cause convection.
Part 1. Air is a fluid
For a class demonstration:
- Baking soda
- 1/4 c. measuring cup
- 500 ml beaker or a glass jar of similar size
- Candle (a small votive candle is ideal)
- Strip of poster board or cardboard that is about 12" by 3" (old file folders work well)
Part 2. Heat causes fluids to move
For the class:
- Pitchers or jugs of room-temperature water
- An electric teakettle, hot plate and pot, or coffee maker to heat water(Hot tap water is usually not hot enough.)
For each team of students:
- Clear plastic plant saucer or platter that is8 to 10 inches wide and at least an inch deep (Note: Do not use saucers with concentric raised rings on the inside bottom; radial ridges are okay.)
- Blue and red food coloring
- Small containers for food coloring such as small cups
- Eye dropper or pipette
- Three disposable cups used for hot beverages
- Bucket to collect used water if a sink is not available
Part 3. Temperature-driven density differences
For each team of students or for a class demonstration:
- A clear plastic shoebox-sized container
- Red food coloring
- Ice cubes made with blue food coloring and water
- Colored pencils (red and blue)
- Index cards or paper
Preparation
- Gather supplies. Note that Part 1works best as a class demonstration for safety reasons; however, Part 2 and Part 3 can be done as a class demonstration by students working in groups of 3 to 4. Only one set of materials is needed for a class demonstration.If performed in small groups, each group will need the materials listed.
- Parts 2and 3 require room temperature water. Leave water overnight or use a thermometer to ensure that the water is around68 to 72 degrees Fahrenheit.
- Prepare ice cubes made with blue food coloring and water at least a day before Part 3.
Part 1: Air is a fluid
Introduce fluids.
Make a ramp for the carbon dioxide by folding a piece of cardboard.
- Discuss the physical properties of fluids with students. Be sure to include the idea that fluids can be poured.
- Ask students "Can you name a fluid?" (Students will likely name liquids like water, milk, and soda.)
- Provide more detail about fluids. Tell students that a fluid is anything that would spill or float away if it weren't in a container. If you can stir it up with a spoon or blow it through a straw, it's a fluid.
- Relying on students' prior understanding of solids, liquids, and gases, ask students whether solids are fluids (generally, they are not). Ask students whether gases are fluids (which is the focus of this activity).
- Explain that, in this demonstration, students will observe whether it's possible to ‘pour’ a gas like a fluid.
- Tells students that this demonstration uses carbon dioxide gas. Because it is denser than air, carbon dioxide should flow down a ramp if it is a fluid. Show students the poster board or cardboard folded lengthwise, which is the ramp (see illustration at right).
- Put about a tablespoon of baking soda in the glass jar or beaker and add approximately 1/4 cup of vinegar. Instruct students to observe the bubbles in the jar. Explain that the vinegar and baking soda are reacting, which is filling the jar with carbon dioxide gas. Note that the carbon dioxide gas is invisible.
- Tell students that carbon dioxide gas, without any oxygen present, will extinguish a fire.(That's why carbon dioxide is used in fire extinguishers.)
- Light a match and hold it in the jar. The flame should go out. (You may need to tip the jar a bit to get the match into the carbon dioxide without burning your finger.)
Do the experiment and observe the results.
When the fizzing subsides, hold the ramp at an angle so that one end is near the candle flame and the other end is slightly higher. Take care to keep the cardboard ramp out of the flame.
- Empty the jar and set up the experiment (as shown in the illustration at the right) with a lit candle below the ramp and the jar at the upper end of the ramp.
- Explain to students that you will again make carbon dioxide in the jar, but this time you will "pour" it down the ramp and see if it will extinguish the candle.
- Add a tablespoon of baking soda and a 1/4 cup of vinegar to the jar. When the fizzing subsides, hold the ramp at an angle so that one end is near the candle flame and the other end is slightly higher.
- "Pour" the gas from the beaker or jar down the funnel. The flame will go out in a second or two.
- Have students explain how the flame was extinguished. (Answer:There was no more oxygen available for the flame, so the flame went out. Pure carbon dioxide, which is a gas, is denser than air, so it flows like a liquid from the jar or beaker along the ramp. Since the carbon dioxide molecules are heavier, these molecules force out the oxygen molecules. The fire cannot continue without oxygen.
Part 2: Heat causes fluids to move
- Tell the class that they will conduct an experiment to observe how air moves when it's heated.
- Tell students that in this experiment water is used to simulate air in the atmosphere. Water and air move similarly because they are both fluids.
- Show students what their experiment set-up should look like (see image at right). Ask students "What do you think will happen to the food coloring when it's dropped near the heat source (cup of hot water) or when it's dropped further from the heat source?"
Place three disposable cups upside down on a piece of paper.
Experiment set-up for Part 2
- Place the plastic plant saucer or plastic platter (here after referred to as a plate) on top of the cups. The cups should be near the outer edges of the plate and evenly spaced.
- Fill the plate three-quarters full with cool water. To make certain the water is still, let it sit before the experiment. Be careful not to bump the desk or table during the experiment.
- Using a dropper, slowly release a small amount of food coloring at the bottom of the plate of water.
- Slowly remove the dropper, taking care not to stir the water.
- Observe and draw what happens as the drop sits on the plate.
- Students should start each trial with a clean plate of water. (If your classroom doesn't have a sink, you may wish to place dump buckets around the classroom.)
- Each group will need one cup of hot water, filled almost to the top. The cup of hot water should be placed under the center of the plate.
- Ask students to draw what happens during each trial from the top view.The drawings should show the movement of the colored water and its relationship to the hot water (heat source).
Place a drop of food coloring on the bottom of the plate in the center, over the cup of hot water. Take care not to stir the water. | |
Place a drop of food coloring on the bottom of the plate about halfway between the center and the side. Take care not to stir the water. | |
Place two drops of food coloring on the bottom of the plate:one halfway between the center and side of the saucer, the other in the center. Take care not to stir the water. |
Observations and Questions
- Have students write explanatory captions for the drawings they made of each trial.
- Have students repeat the exercise making drawings from the side of the plate.
- Ask students: How does heat affect the food coloring? (Answer: The dye moved more when placed near the heat source.)
- (Advanced Question) If students have learned about heat transfer, ask them to explain what type of heat transfer is taking place.( Answer:Convection is occurring because we can observe the movement of the colored water within the liquid. Convection transmits heat through the movement of molecules.)
Part 3: Temperature-driven density differences
- Introduction:
- To connect Part 2 and Part 3, discuss radiation and convection. The transfer of heat from the water in the cup to the plate in Part 2 is radiation. Convection , another method of heat transfer, is the movement of water in the plate during Part 2. (For additional information on radiation and convection, refer to the background section of this activity.)
- Show students what their experiment set up will look like for Part 3(a shoebox of water, blue ice cubes, and red food coloring). Tell students that this experiment is more like the atmosphere compared to Part 2 because there is a larger thickness/volume of fluid. Remind students that water is representing the same movement found in the air.
- Ask students "From what you have learned in Part 2, will the cold (blue) water rise or sink in comparison to the warm (red) water? Why?
- Provide each group with a plastic container 2/3 full of room temperature water.Instruct students not to move the container or table so that the water becomes completely still.
- Provide each group with a blue ice cube to put at one end of their container (alternatively, use a drop of blue coloring on ice).For best results, add the ice cube with as little disturbance to the water as possible.
- Put two drops of red food coloring at the other end of each container. (For dramatic effect, heat the red food coloring in warm water.).Take care not to stir the water as you add the food coloring.
Have students observe the long sides of the container to see where the blue and red food coloring travel. Example:
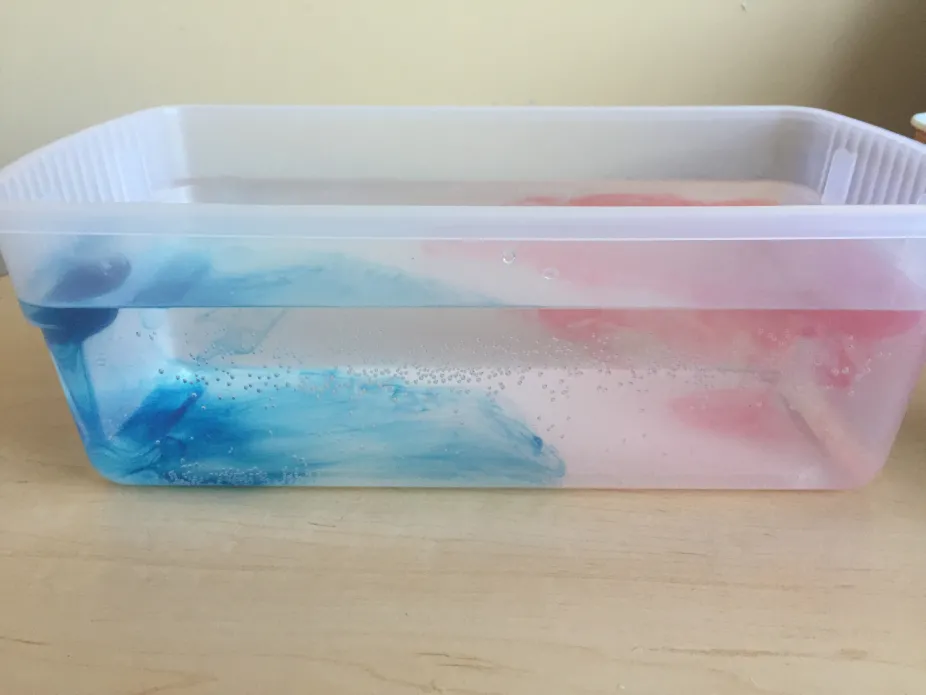
- Ask students to draw a picture that describes their observations and then compare their pictures with pictures made by other groups.
- Did similar things happen to the food coloring in different groups? ( Answer: Results should be similar.)
- What happened to the blue, cold food coloring? ( Answer: It sunk. )
- What happened to the red, warm food coloring? ( Answer: The red, warm water rose towards the surface of the water and spread out above the blue, cold water.)
- What was the difference between the blue and red water? ( Answer: Temperature difference. If you have discussed density, students should know that there is also a density difference.)
- Do warm fluids (e.g. water or air) rise or sink compared to cold fluids? Explain why. (Answer: The temperature difference causes movement of water at different levels because less dense, warm water rises while the more dense cold water sinks.)
- (Advanced Question) Using knowledge from Parts 1 and 2, how could you apply this idea of warm, water rising and cold, water sinking to the air in the atmosphere since both are fluids?
- Ask students to imagine a situation similar to Part 2, except this time, a cup of ice water is substituted for the cup of hot water.How would the circulation look? (Cold water should flow along the bottom of the saucer until it nears the warmer sides. Then it will rise up. As it reaches the top, the water will cool and sink, drawing water from the sides in toward the center.)
A fluid is any substance that flows. Fluids include liquids, gases, and some solids like glacial ice. Fluids take the shape of a container because they do not have a specific shape and include common substances like water, shampoo, sunscreen, honey, and air. Air is a mixture of gases that flows and takes the form of its container, so it is a fluid.
Fluids flow due to different densities. The chemical composition of fluids influences density. For example, fresh water is less dense than salt water; therefore, salt water is heavier and sinks below the freshwater. Another example (as seen in Part 1) is that carbon dioxide is heavier (denser) than air, allowing carbon dioxide to force air out of the way and extinguish the fire. The fire cannot continue without a supply of oxygen, such as the oxygen found in air.
Temperature changes can also affect the density of a fluid. Adding heat to a fluid increases the motion of the molecules, which then spread further apart. Warm fluids are less dense and rise while cold fluids are denser and sink. The circulation of rising and sinking air is called convection.
Convection is the transfer of heat by the movement of a heated material. In the atmosphere, on a hot summer day, the surface of the Earth is heated by energy from the Sun. Then, the warmed Earth surface warms air near the ground. The warm air rises, while cooler air sinks towards the ground. That cooler air is then warmed by the Earth's surface and rises, continuing the convection pattern. Convection plays a key role in the formation of clouds and even thunderstorms. There is a limit of how far the warm air can rise in the atmosphere because air temperatures decrease with increasing altitude .
The continual cycling due to temperature-driven density, convection currents, are found in many places and on many scales in the atmosphere, ocean, and even in the Earth's interior. Smaller convection currents can be found in a cup of hot cocoa or a fish tank. Convective motions in the atmosphere are responsible for the redistribution of heat from the warm equatorial regions to higher latitudes and from the surface upward.
The main three processes of heat transfer include radiation, conduction, and convection.
Consider what happens to the water in a pot as it is heated over an open camp stove.In this example, radiation transfers heat from the burner to the pot. ( Additional background information on radiation can be found here .) Convection moves heat and water in the pot. The water at the bottom of the pot heats up first and expands. Since the warmed water has a lower density than the water around it, it rises up through the cooler, dense water. At the top of the pot, the water cools, increasing its density, which causes it to sink back down to the bottom. This movement distributes heat within the pot.
Related Resources
- Radiation and Albedo Activity
- How Clouds Form
- Atmospheric Circulation
- Virtual Balloon Activity
Convection Currents in Science, What They Are and How They Work
Three-shots/Pixabay
- Chemical Laws
- Periodic Table
- Projects & Experiments
- Scientific Method
- Biochemistry
- Physical Chemistry
- Medical Chemistry
- Chemistry In Everyday Life
- Famous Chemists
- Activities for Kids
- Abbreviations & Acronyms
- Weather & Climate
- Ph.D., Biomedical Sciences, University of Tennessee at Knoxville
- B.A., Physics and Mathematics, Hastings College
Convection currents are flowing fluid that is moving because there is a temperature or density difference within the material.
Because particles within a solid are fixed in place, convection currents are seen only in gases and liquids. A temperature difference leads to an energy transfer from an area of higher energy to one of lower energy.
Convection is a heat transfer process. When currents are produced, matter is moved from one location to another. So this is also a mass transfer process.
Convection that occurs naturally is called natural convection or free convection . If a fluid is circulated using a fan or a pump, it's called forced convection . The cell formed by convection currents is called a convection cell or Bénard cell .
Why They Form
A temperature difference causes particles to move, creating a current. In gases and plasma, a temperature difference also leads to regions of higher and lower density, where atoms and molecules move to fill in areas of low pressure.
In short, hot fluids rise while cold fluids sink. Unless an energy source is present (e.g., sunlight, heat), convection currents continue only until a uniform temperature is reached.
Scientists analyze the forces acting on a fluid to categorize and understand convection. These forces may include:
- Surface tension
- Concentration differences
- Electromagnetic fields
- Bond formation between molecules
Convection currents can be modeled and described using convection- diffusion equations, which are scalar transport equations.
Examples of Convection Currents and Energy Scale
- You can observe convection currents in water boiling in a pot. Simply add a few peas or bits of paper to trace the current flow. The heat source at the bottom of the pan heats the water, giving it more energy and causing the molecules to move faster. The temperature change also affects the density of the water. As water rises toward the surface, some of it has enough energy to escape as vapor. Evaporation cools the surface enough to make some molecules sink back toward the bottom of the pan again.
- A simple example of convection currents is warm air rising toward the ceiling or attic of a house. Warm air is less dense than cool air, so it rises.
- Wind is an example of a convection current. Sunlight or reflected light radiates heat, setting up a temperature difference that causes the air to move. Shady or moist areas are cooler, or able to absorb heat, adding to the effect. Convection currents are part of what drives global circulation of the Earth's atmosphere.
- Combustion generates convection currents. The exception is that combustion in a zero-gravity environment lacks buoyancy, so hot gases don't naturally rise, allowing fresh oxygen to feed the flame. The minimal convection in zero-g causes many flames to smother themselves in their own combustion products.
- Atmospheric and oceanic circulation are the large-scale movement of air and water (the hydrosphere), respectively. The two processes work in conjunction with each other. Convection currents in the air and sea lead to weather .
- Magma in the Earth's mantle moves in convection currents. The hot core heats the material above it, causing it to rise toward the crust, where it cools. The heat comes from the intense pressure on the rock, combined with the energy released from natural radioactive decay of elements. The magma can't continue to rise, so it moves horizontally and sinks back down.
- The stack effect or chimney effect describes convection currents moving gases through chimneys or flues. The buoyancy of air inside and outside of a building is always different due to temperature and humidity differences. Increasing the height of a building or stack increases the magnitude of the effect. This is the principle on which cooling towers are based.
- Convection currents are evident in the sun. The granules seen in the sun's photosphere are the tops of convection cells. In the case of the sun and other stars, the fluid is plasma rather than a liquid or gas.
- A to Z Chemistry Dictionary
- 10 Types of Energy With Examples
- Examples of Solids, Liquids, and Gases
- Flame Temperatures Table for Different Fuels
- A Scientific Way to Define Heat Energy
- Convection and Weather
- How Ocean Currents Work
- Does Blowing on Hot Food Really Make It Cooler?
- What Are the Bubbles in Boiling Water?
- The Chemistry of Weather: Condensation and Evaporation
- Understanding What Fluid Dynamics is
- Sun Facts: What You Need to Know
- Air Pressure and How It Affects the Weather
- Is Fire a Gas, Liquid, or Solid?
- What Is Conduction?
- Winds and the Pressure Gradient Force
Sciencing_Icons_Science SCIENCE
Sciencing_icons_biology biology, sciencing_icons_cells cells, sciencing_icons_molecular molecular, sciencing_icons_microorganisms microorganisms, sciencing_icons_genetics genetics, sciencing_icons_human body human body, sciencing_icons_ecology ecology, sciencing_icons_chemistry chemistry, sciencing_icons_atomic & molecular structure atomic & molecular structure, sciencing_icons_bonds bonds, sciencing_icons_reactions reactions, sciencing_icons_stoichiometry stoichiometry, sciencing_icons_solutions solutions, sciencing_icons_acids & bases acids & bases, sciencing_icons_thermodynamics thermodynamics, sciencing_icons_organic chemistry organic chemistry, sciencing_icons_physics physics, sciencing_icons_fundamentals-physics fundamentals, sciencing_icons_electronics electronics, sciencing_icons_waves waves, sciencing_icons_energy energy, sciencing_icons_fluid fluid, sciencing_icons_astronomy astronomy, sciencing_icons_geology geology, sciencing_icons_fundamentals-geology fundamentals, sciencing_icons_minerals & rocks minerals & rocks, sciencing_icons_earth scructure earth structure, sciencing_icons_fossils fossils, sciencing_icons_natural disasters natural disasters, sciencing_icons_nature nature, sciencing_icons_ecosystems ecosystems, sciencing_icons_environment environment, sciencing_icons_insects insects, sciencing_icons_plants & mushrooms plants & mushrooms, sciencing_icons_animals animals, sciencing_icons_math math, sciencing_icons_arithmetic arithmetic, sciencing_icons_addition & subtraction addition & subtraction, sciencing_icons_multiplication & division multiplication & division, sciencing_icons_decimals decimals, sciencing_icons_fractions fractions, sciencing_icons_conversions conversions, sciencing_icons_algebra algebra, sciencing_icons_working with units working with units, sciencing_icons_equations & expressions equations & expressions, sciencing_icons_ratios & proportions ratios & proportions, sciencing_icons_inequalities inequalities, sciencing_icons_exponents & logarithms exponents & logarithms, sciencing_icons_factorization factorization, sciencing_icons_functions functions, sciencing_icons_linear equations linear equations, sciencing_icons_graphs graphs, sciencing_icons_quadratics quadratics, sciencing_icons_polynomials polynomials, sciencing_icons_geometry geometry, sciencing_icons_fundamentals-geometry fundamentals, sciencing_icons_cartesian cartesian, sciencing_icons_circles circles, sciencing_icons_solids solids, sciencing_icons_trigonometry trigonometry, sciencing_icons_probability-statistics probability & statistics, sciencing_icons_mean-median-mode mean/median/mode, sciencing_icons_independent-dependent variables independent/dependent variables, sciencing_icons_deviation deviation, sciencing_icons_correlation correlation, sciencing_icons_sampling sampling, sciencing_icons_distributions distributions, sciencing_icons_probability probability, sciencing_icons_calculus calculus, sciencing_icons_differentiation-integration differentiation/integration, sciencing_icons_application application, sciencing_icons_projects projects, sciencing_icons_news news.
- Share Tweet Email Print
- Home ⋅
- Science Fair Project Ideas for Kids, Middle & High School Students ⋅
Convection Experiments for Kids
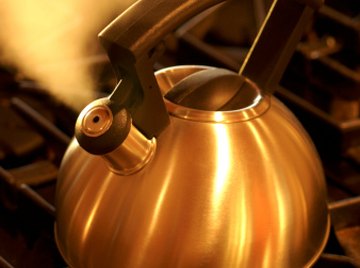
How to Make a 3D Model of a Typhoon
Convection is the cycle of transferring heat. It is a fascinating topic to tackle when attempting scientific experiments with kids, because it’s something that occurs in liquid and the air on a daily basis. Convection is also something that can be tested and understood without using expensive laboratory equipment and tools.
Convection Snake
To perform the convection snake experiment, you will need a piece of paper and scissors. Using the scissors, cut the piece of paper into a spiral shape that is 6 cm long. Attach one end of a 15-cm piece of yarn to the middle of the spiral using clear tape. Take a table lamp and hang the paper spiral about 10 cm above the table lamp. You will notice that the heat from the lamp causes the spiral to twirl. This is due to a convection current. The energy from the lamp's light source warms up the air above it. Because hot air is lighter than cool air, as the air heats up and the hot air rises above the lamp, colder air moves where the warmer air was previously.
Convection Currents Experiment: Materials
If you watch the news, you see weather forecasters talking about natural weather phenomena such as El Nino and La Nina. El Nino and La Nina are caused by convection, because convection currents form when warm and cold air come together in the atmosphere. This creates warm water currents in the ocean. To create your own convection current, you will need four plastic soda bottles that are the same size, two different colors of food coloring, warm and cold water and an index card.
Convection Currents Experiment: Procedure and Results
Completely fill two of the soda bottles with cold water and the other two bottles with warm water. Use the food coloring to color the cold water one color and the warm water the other color. Put your index card over the top of one of the bottles of warm water. Holding the index card in place, inverse the bottle and put it on top of one of the bottles of cold water. Slide the index card from in between the bottles. You will see that the cold water, which is heavier, stays in the bottom bottle and the warm water stays in the top bottle. However, if you perform the experiment placing the bottle of cold water on top and the bottle of warm water on the bottom, the warm water would rise to the top and the cold water would move to the bottom bottle.

Boiling Water
A simple illustration of convection is a pot or kettle of boiling water. When you boil water to make beverages or cook meals, though you start off with cool water, as the water is warmed by an external heating source, it begins to expand. As the hotter water rises, colder water in other parts of the pot or kettle moves in to replace the warmer water. As this process continues, the water gets hot and the convection currents cause the liquid to move in a circular fashion. Eventually, the action becomes very strong and the water begins to boil.
Related Articles
Food coloring experiments, science projects: how hot & cold water changes a balloon, how to make a 3d model of a hurricane, thermal energy science experiments for kids, cell respiration lab ideas, science project egg experiments, how to turn a glass of water with red dye back into..., how to mix calcium chloride and water, convection currents in volcanoes, what happens when you add a drop of food coloring to..., science projects using dawn dishwashing liquid, cool science project ideas for k-4th grade, magic science tricks for kids, how to make a water filter as a science experiment, how to make a hurricane for a science project.
- Weather Questions: What is Convection?
- Ozemail: Convection Snake
About the Author
Mike Jones is an Atlanta native who has been writing professionally since 2000. He has written a number of entertainment, health and how-to articles for online publications such as eHow and Answerbag. He holds a master's degree in journalism from Regent University.
Photo Credits
Thinkstock/Comstock/Getty Images
Find Your Next Great Science Fair Project! GO
- Recipes for PLAY
- Letter of the week
- Kindergarten
- First Grade
- My Father's World
- Sensory Play
- Indoor Play
- Outdoor Play
- Imaginative Play
- Small World Play
August 1, 2013
Making convection currents, observing convection - weather for kids.
.jpg)
How to Make Convection Currents
- blue ice cubes (made by adding a few drops of food coloring to the ice tray before freezing)
- red food coloring
- plastic container
6 comments:
love finding neat science ideas. Thanks for sharing. I pinned this post.
This looks a good fun practical way to show kids science - thank you for sharing.
Thank you for sharing. can't wait to do this - with my kids...
I've done this experiment in the past using four cups of water underneath the bin. Two cups of cold water on one end and two cups of "hot" water on the other end. The food coloring drops are added to the appropriate temperature end of the bin. The students are mesmerized by the swirling currents. I look forward to trying your method with the blue ice cubes. Thanks for sharing.
Hi if we take a small styrofoam piece(around 4 inch square) will it work. Mine is not working.
Thanks Allison for sharing this! What a fun way to teach weather currents... I am going to share this on my Children's Corner post this week, thank you! Marissa @ Reading List
Thank you for visiting and taking the time to comment. I love reading your feedback. Have a blessed day!
Search This Site
Browse Learn Play Imagine
Popular posts, you may pin my images, grab my badge.
ekunji – Key of Knowledge
- Key of Wonders
- Chutney & Sauce Recipe
- Rice Recipes
- Vegetable Recipes
- Roti / Paratha Recipes
- Raita Recipes
- Dessert Recipes
- Snacks Recipes
- Vrat Recipes
- Salad Recipes
- Pickle Recipes
- Festival Recipes
- Beverage Recipes
- Ice Cream Recipes
- Curry Recipes
- Cake Recipes
- Cookies Recipes
- Pizza Recipes
- Bread Recipes
- Muffin Recipes
- Key of Technology
- Key of Professionalism
- Key of Health
- Arts and Crafts
- Beauty Tips
- Science Projects For Kids
- Key of Indian Politics
- Ekunji Store
Convection Currents in Water – Science Experiment
- 26. Dec. 2014
- No Comments
When your kid comes to know first time about convection currents in water, it is difficult for you to demonstrate it. Today I will show you a very easy science experiment to demonstrate convection currents in water.
Apparatus Required for Convection Currents in Water Experiment :
- Tripod stand
- Colored paper
- A paper punch machine
Convection Currents in Water : Video
Steps for Convection Currents in Water Experiment :
1. First of all fill the flask with water.
2. Take out circular bits of colored paper with the help of paper punch.
3. Drop some paper bits in flask and immerse them properly in water.
4. Put the flask over burner and heat for 5 minutes.
5. As the water heated, you will see the movement in paper bits due to convection currents in water.
Why Did This Happen?
When you put flask on flame, then the water at the bottom of the flask starts to heat. Hot water is always lighter than the cold water. Hence, the water starts to flow upward in the flask and the cold water of the upper surface starts to move down. These are called convection currents in water.
Your paper bits also starts to move up and down with these convection currents. This movement of paper bits continues till the entire water in the flask reached at same temperature.
This is the easiest experiment to show and demonstrate convection currents in water to your kids.
- Subscribe Sameer Goyal at ekunji to get easy science projects and craft ideas for kids.
- Subscribe Sameer Goyal at Youtube channel for more science projects and craft ideas videos.
- Join Sameer Goyal at Facebook
- Join Sameer Goyal at Pinterest
- Join Sameer Goyal at Twitter
- Join Sameer Goyal at Google+
Try this easy science experiment to demonstrate convection currents in water and write me your feedback in comment area below.
Leave a Reply Cancel reply
Your email address will not be published. Required fields are marked *
Save my name, email, and website in this browser for the next time I comment.
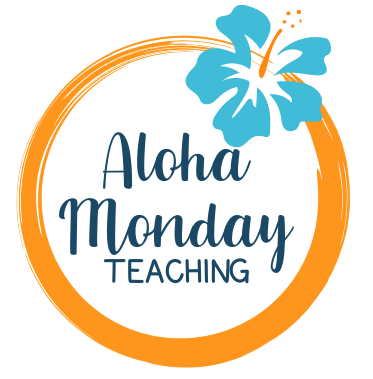
Aloha Monday Teaching
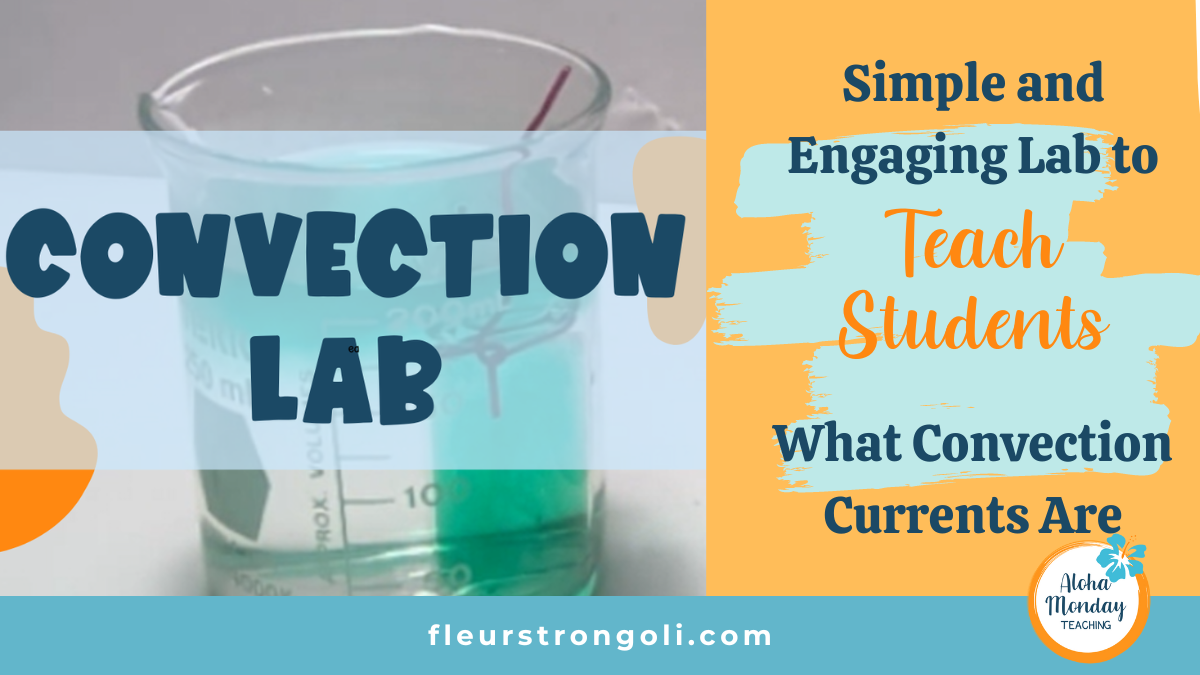
3 Engaging and Simple Ways to Have Convection Current Explained
Are you looking for help to have convection current explained to your students? Do you need some ideas for simple convection currents experiments?
Let’s talk about a lab that demonstrates how convection currents work!
In this post
We are going to go over convection currents and when we teach them in Science class. I will also share one of my favorite labs I like to do in my classes to show my students how convection currents work.
Get the Convection Lab here.
And remember to get your free guide 5 Daily Must-Do Routines to Run Your Classroom Like a Pro !
When to teach convection currents
Convection currents come up a lot in Science class, and it can be supported by these two NGSS standards:
MS-ESS2-1. Develop a model to describe the cycling of Earth’s materials and the flow of energy that drives this process.
MS-ESS2-6. Develop and use a model to describe how unequal heating and rotation of the Earth cause patterns of atmospheric and oceanic circulation that determine regional climates.
When we are teaching our students about the air pressure and winds, ocean currents, or Earth’s interior, we have an opportunity to teach them about convection currents.
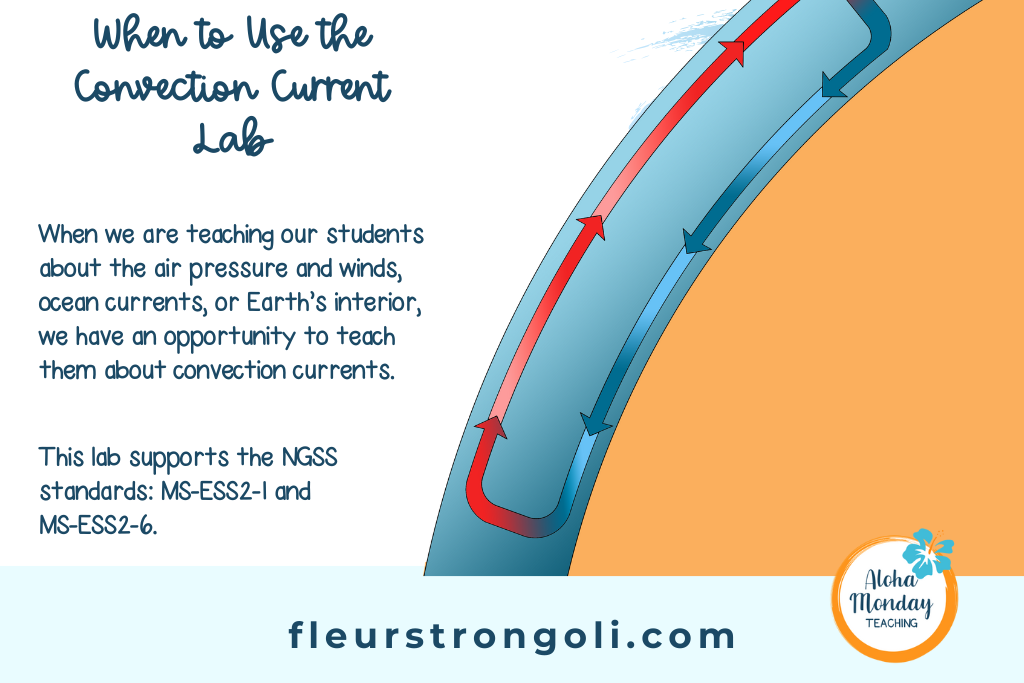
Convection is how heat transfers through liquid or gas. Hotter material will rise because it is less dense, while the cooler material sinks because it is more dense.
We see this in the atmosphere when we teach students about air pressure and winds. Cooler air from the poles sinks and moves toward the warmer air at the equator. The warm air at the equator rises and moves towards the poles. This causes the convection currents and cells that form global winds.
In the ocean, cold (and salty) water at the poles sinks to form deep ocean currents. This moves around the ocean floor, forming the global conveyor belt. When the water warms near the equator, it rises.
And in the mantle, cooler rock in the lithosphere sinks while hotter rock near the core rises. We teach this to show how matter is moving in Earth’s interior and how rocks and minerals are formed.
Convection Lab
There are many labs you can do with your students. This is one I enjoy doing with my students to show them how heat is transferred through liquid. First, I start with a demo using a paper plate (cut up in spiral pattern) and a hot plate. I use the heat from the hot plate to show the heat transferring through gas and spinning the spiral plate.
Then we do this lab. I like to do this toward the beginning of the year during our weather and climate unit for two reasons. First, they gain an understanding of convection currents. And second, I always refer to it since it is now their background knowledge.
Click here to get the convection lab in my TpT store. You’ll get the Power Point, lab sheet, and a lesson plan with a shopping list.
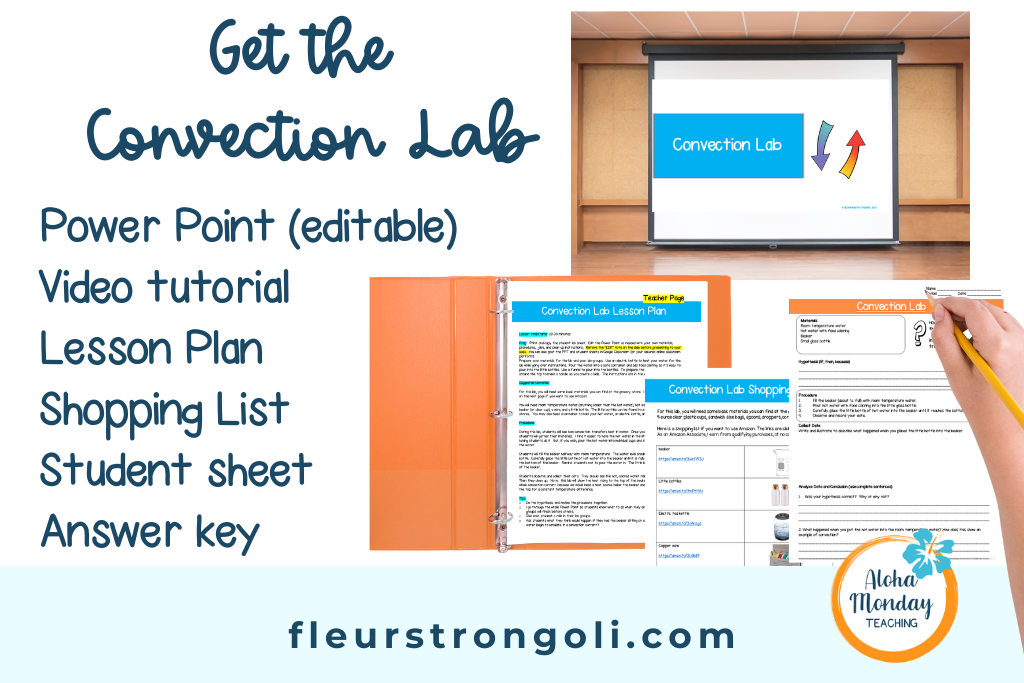
Lab Materials
The materials you need include a beaker, a small glass bottle, wire, hot water, room temperature water, and food coloring. I use an electric tea kettle to heat up my water. You may also want a cup to hold the hot water and a funnel to pour the hot water into the small glass bottle.
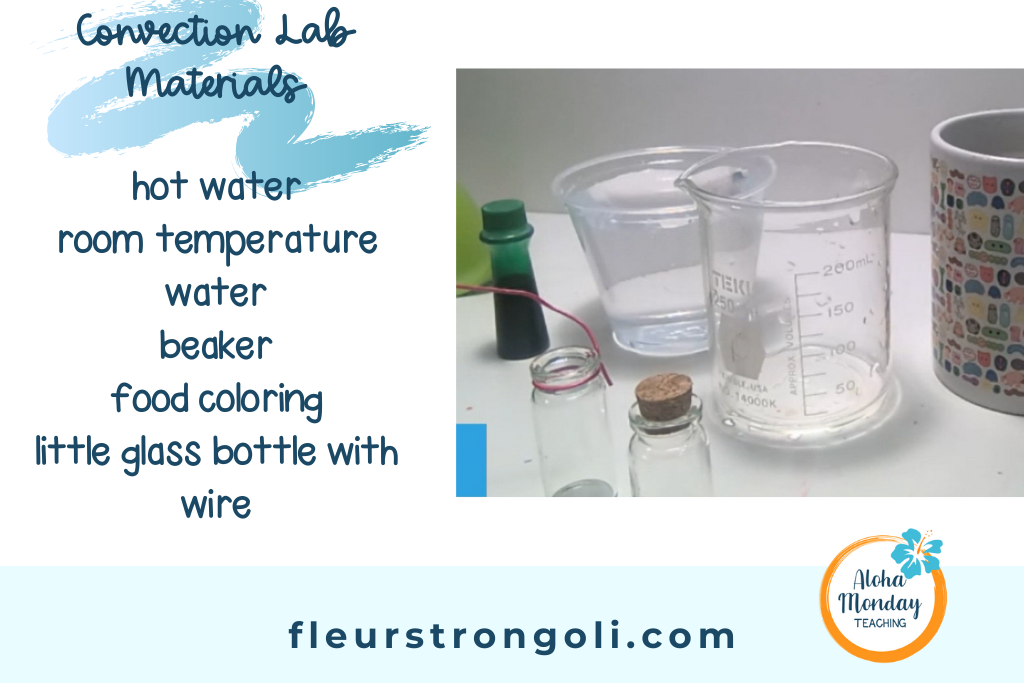
Lab Preparation
To prepare for the lab, heat the water just before students gather materials. Add food coloring to the hot water. Wrap wire around the small glass bottle to create a ladle. Place all of the materials on a tray.
Conducting the Lab with Students
I use a PowerPoint for each lab I do with students. This gives them a visual of each part of the lab along with directions for conducting the lab and cleaning up. Read more about managing labs in this blog post.
Students will fill the beaker with room temperature (or cooler) water. Then they will slowly lower the glass bottle into the beaker until it is submerged. They should not pour it in. The glass bottle should sit at the bottom of the beaker.
Students should see convection taking place. The hot, colored water will rise out of the glass bottle into the rest of the water. They might even see it sink when it reaches the top of the beaker.
Wrapping Up the Lab
When students are finished with the lab, it’s important for them to clean up and to analyze their results or data.
For this lab, I like to ask questions such as:
Was your hypothesis correct? Explain.
How does this model convection currents?
How do you think convection currents move in the (atmosphere, earth’s mantle, ocean)?
Teaching convection currents is ongoing and fun! This lab will really help students understand how convection currents work. We went over when we would teach convection currents and how to use this lab in your classroom. You can get the Convection Lab in my TpT store.
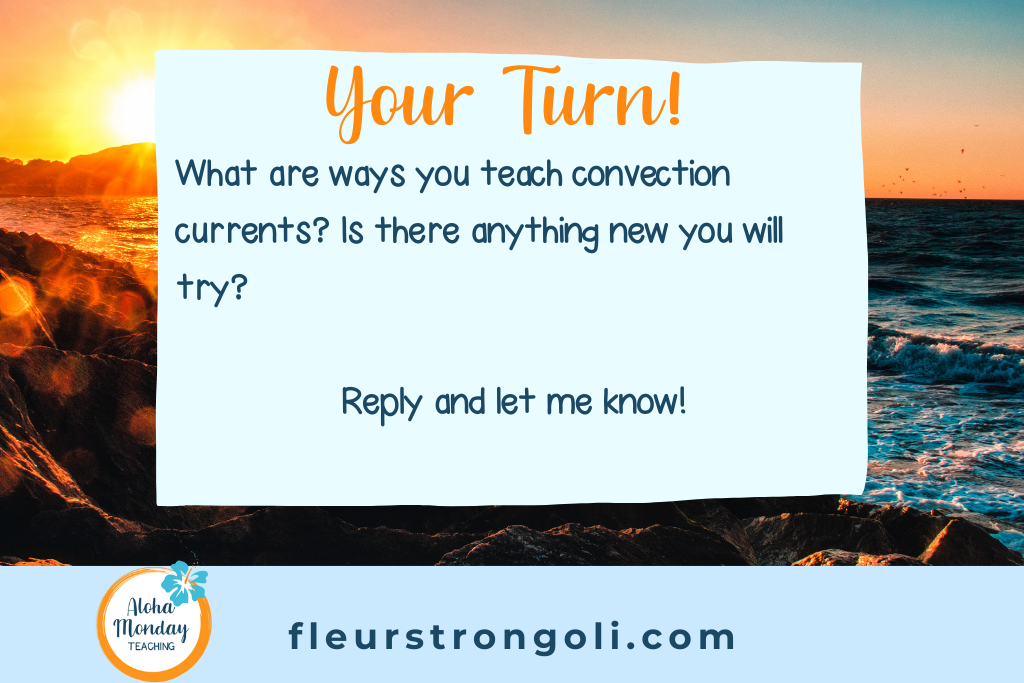
What are ways you teach convection currents? Is there anything new you will try? Reply and let me know!
Read More/Links
Convection Lab on TpT
What is a Science Lab and How Do I Manage It Effectively?
Similar posts.
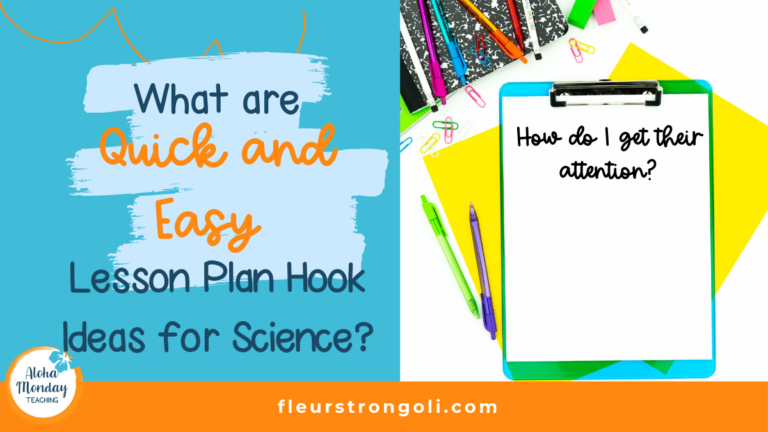
What are Quick and Easy Lesson Plan Hook Ideas for Science?
What do you think about lesson plan hook ideas? Let’s try this activity. Look at this image of two separate classrooms. What do you observe? One classroom is full of students who are bored, not engaged. The other classroom has students participating and asking questions. Why is this? Quite possibly, the teacher in the…
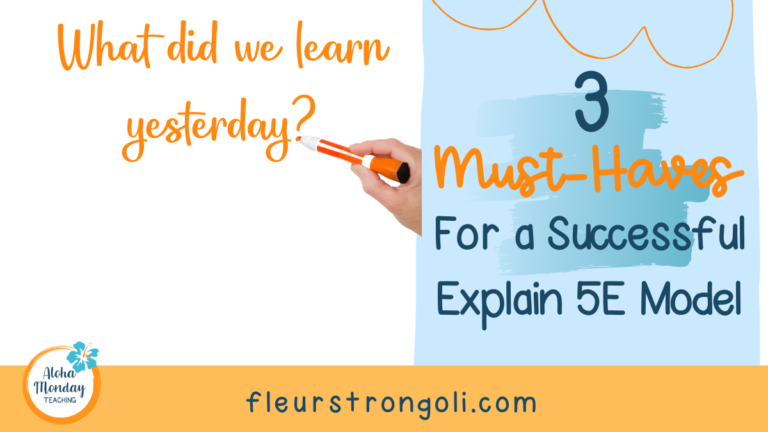
3 Must-Haves for a Successful Explain 5E Model
We’ve engaged and explored, now it’s time to Explain 5E Model. This is your time to shine and project your best teaching practices with direct instruction. So, what are the components to successfully implement the Explain step? In This Post Explain is the guided instruction piece of the 5E Model. We will look at what…
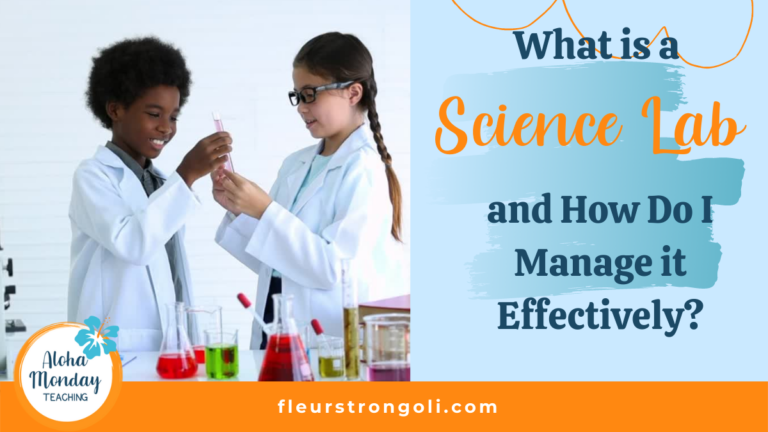
Teaching middle school science is a lot of fun, and kids get excited when most of them finally get the answer to their question, “What is a science lab?” As teachers we need to make sure we are managing it so students are safe, engaged, and learning. Oh, and so we are not stressed too. In…
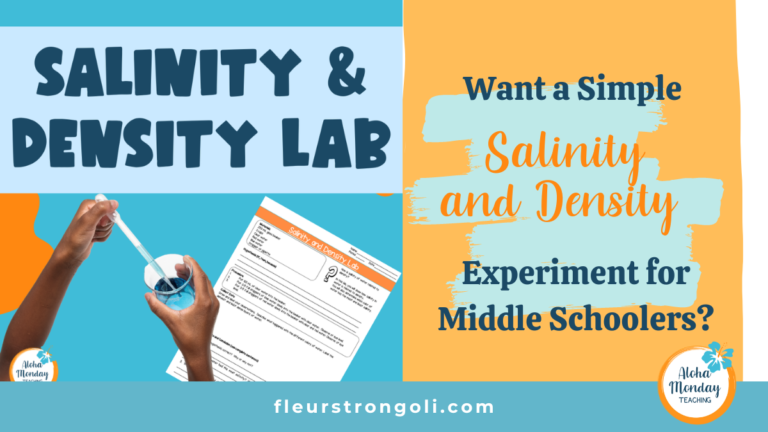
Want a Simple Salinity and Density Experiment for Middle Schoolers?
Here is a salinity and density experiment that demonstrates how differences in salinity are related to density without having to go to the poles to show students how salinity and density create deep ocean currents. . This lab also gets students guessing which water has the greatest salinity. In this post We will go over salinity…
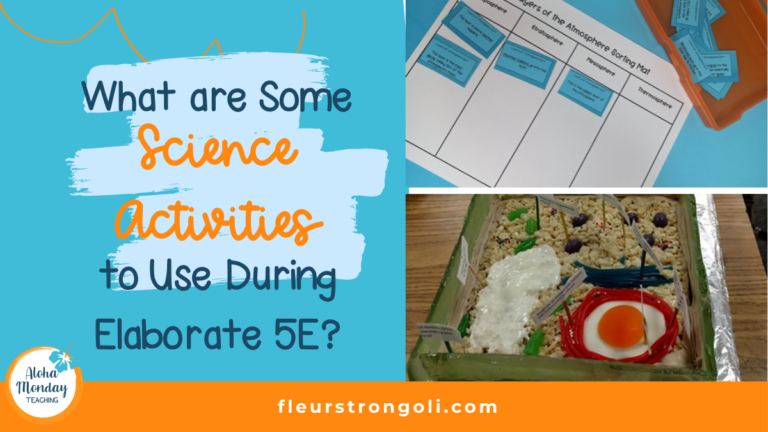
What are Some Science Activities to Use During Elaborate 5E?
What are some science activities you can assign that are meaningful, engaging, and grow students’ brains? This is what Elaborate is all about! It’s time to have students practice and expand on what you taught them in Explain. In This Post We will discuss what Elaborate is in a 5E unit. I will share different…
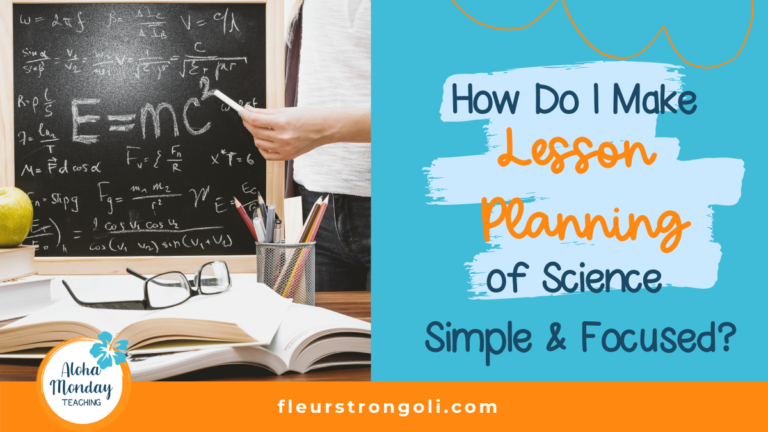
How Do I Make Lesson Planning of Science Simple and Focused?
Why is lesson planning of science such an important thing to do? Think back to your college days. Some of you may have just graduated and others, like me, feel like college was in another lifetime. But I bet you can remember writing those detailed lesson plans for your education classes. Some school districts…
Leave a Reply Cancel reply
Your email address will not be published. Required fields are marked *
Save my name, email, and website in this browser for the next time I comment.
Heat transfer - CCEA Convection
Learn about how heat transfer occurs.
Part of Physics (Single Science) Energy
Heat can be transferred in liquids or gases by convection close convection The transfer of heat energy through a moving liquid or gas. .
Liquids and gases are fluids because they can be made to flow.
The particles close particle A general term for a small piece of matter. For example, protons, neutrons, electrons, atoms, ions or molecules. in these fluids can move from place to place.
Convection occurs when particles with a lot of heat energy in a liquid or gas move and take the place of particles with less heat energy.
Convection in a liquid
Convection in a liquid can be seen by putting a crystal of potassium permanganate in a beaker of water and gently heating it with a Bunsen flame.
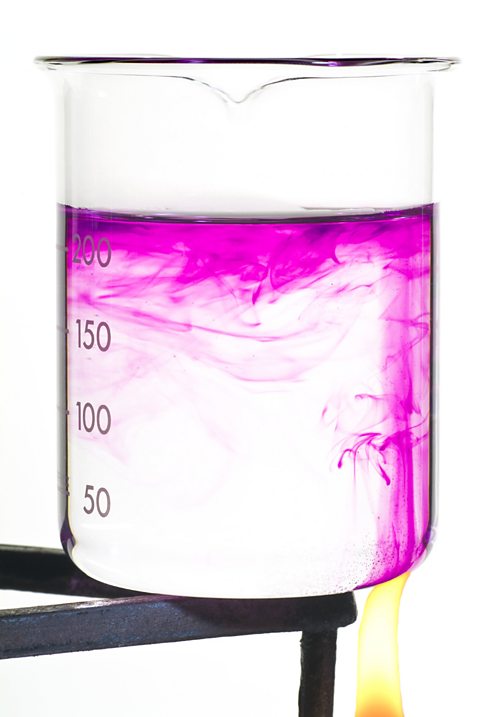
- Air close to the radiator is heated.
- It is replaced by the cooler, denser air which surrounds it.
- This air is in turn heated, expands becomes less dense and rises.
- The process continues, a convection current is set up and heat is transferred through the air and hence through the room.
Convection currents enable hot air balloons to rise, and also explain why it is often hotter in houses upstairs rather than downstairs.
Most of our winds are caused by convection currents occurring on a big scale in air.
Many ocean currents are also due to convection currents.
More guides on this topic
- Energy forms - CCEA
- Energy resources - CCEA
- Work, power and efficiency - CCEA
- Kinetic and gravitational potential energy - CCEA
Related links
- BBC Science
- School Science
- Revision Buddies Subscription
- Heat Introduction Classification
Convection Currents
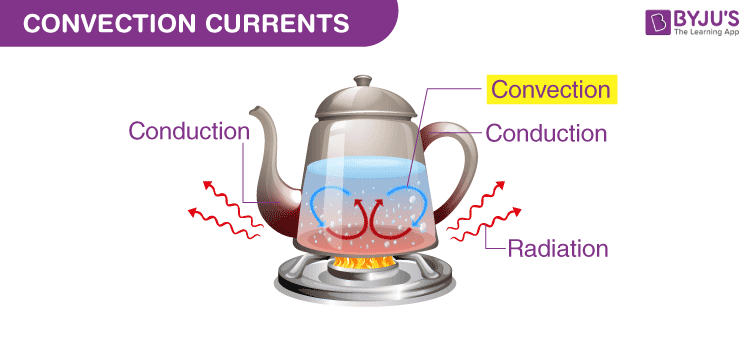
Convection currents tend to move fluid or gas particles from one place to another. These are created as a result of the differences occurring within the densities and temperature of a specific gas or fluid. Convection is one of the forms of heat transfer, of which the other two are radiation and conduction. The convection process only happens in fluids, i.e. in liquids and gases. This happens due to the reason that molecules within liquids or gases are free to move.
The heat energy can be transferred by the process of convection by the difference occurring in temperature between the two parts of the fluid. Due to this temperature difference, hot fluids tend to rise, whereas cold fluids tend to sink. This creates a current within the fluid called a convection current.
The mantle within the earth’s surface flows due to convection currents. These currents are mainly caused by a very hot material present in the deepest part of the mantle, which rises upwards, then cools, sinks, again and again, repeating the same process of heating and rising.
Hence, convection current is defined as “a process of continuous heating up of liquids or gases by the process called convection. “
Read More: Heat Transfer by Convection
Convection Currents- Examples
- Boiling of Water – When boiling water on a stove or while making tea, or while boiling an egg. The temperature of molecules within those liquids increases, and they slowly begin to move at a rapid rate. These molecules get charged up, thus producing kinetic energy . These hot water molecules residing near the heat source tend to become less dense. They rise above cooler dense molecules. As these hotter molecules rise, they tend to cool down and begin to sink, replacing cooler molecules. These movements occurring within this boiling water what the convection currents.
- Campfires – The hotness which we feel around a campfire is all that convection currents heating up your hand. Heat comes from various kinds of heat transfers, such as radiation. But while placing your hand above a campfire, a lot of convection currents rises towards you.
- Changes in the weather- The cool air and breeze occurring near to a beach are all the effects of convection currents. The weather changes on a daily basis are also affected by these currents.
- Convection currents occurring in the ocean – The Oceanic currents are also the convection currents. These are caused due to the difference in the water density and the temperature occurring in different parts of the ocean.
- Convection currents are present in the air – A good example of convection current is the warm air that rises towards the ceiling in your house. The process happens as the warm air is said to be less dense than that of the colder air. Another good example of convection current is wind. The wind is mainly caused when the reflected radiation of the light from the sun heats up the air, thus displacing the cooler air.
Watch the video and learn about convection currents
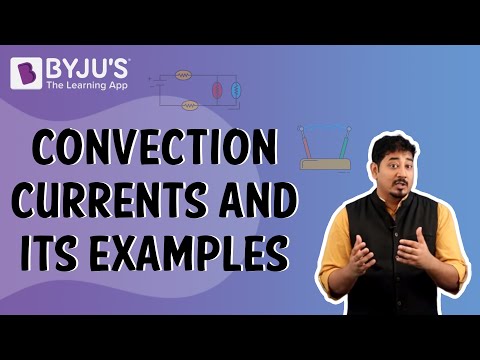
Put your understanding of this concept to test by answering a few MCQs. Click ‘Start Quiz’ to begin!
Select the correct answer and click on the “Finish” button Check your score and answers at the end of the quiz
Visit BYJU’S for all Physics related queries and study materials
Your result is as below
Request OTP on Voice Call
PHYSICS Related Links | |
Leave a Comment Cancel reply
Your Mobile number and Email id will not be published. Required fields are marked *
Post My Comment

thank you i like it

Register with BYJU'S & Download Free PDFs
Register with byju's & watch live videos.
Thank you for visiting nature.com. You are using a browser version with limited support for CSS. To obtain the best experience, we recommend you use a more up to date browser (or turn off compatibility mode in Internet Explorer). In the meantime, to ensure continued support, we are displaying the site without styles and JavaScript.
- View all journals
- Explore content
- About the journal
- Publish with us
- Sign up for alerts
- Open access
- Published: 19 June 2024
Free convection in a square wavy porous cavity with partly magnetic field: a numerical investigation
- Amirmohammad Mirzaei 1 ,
- Bahram Jalili 2 ,
- Payam Jalili 2 &
- Davood Domiri Ganji 3
Scientific Reports volume 14 , Article number: 14152 ( 2024 ) Cite this article
130 Accesses
Metrics details
- Fluid dynamics
- Mechanical engineering
Natural convection in a square porous cavity with a partial magnetic field is investigated in this work. The magnetic field enters a part of the left wall horizontally. The horizontal walls of the cavity are thermally insulated. The wave vertical wall on the right side is at a low temperature, while the left wall is at a high temperature. The Brinkman-Forchheimer-extended Darcy equation of motion is utilized in the construction of the fluid flow model for the porous media. The Finite Element Method (FEM) was used to solve the problem’s governing equations, and the current study was validated by comparing it to earlier research. On streamlines, isotherms, and Nusselt numbers, changes in the partial magnetic field length, Hartmann number, Rayleigh number, Darcy number, and number of wall waves have been examined. This paper will show that the magnetic field negatively impacts heat transmission. This suggests that the magnetic field can control heat transfer and fluid movement. Additionally, it was shown that heat transfer improved when the number of wall waves increased.
Similar content being viewed by others
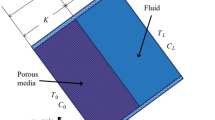
Investigating double-diffusive natural convection in a sloped dual-layered homogenous porous-fluid square cavity
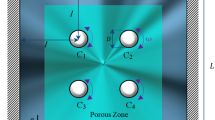
Numerical investigation of transient mixed convection of nanofluid in a cavity with non-Darcy porous inner block and rotating cylinders with harmonic motion
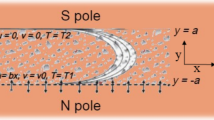
An investigation into a semi-porous channel's forced convection of nano fluid in the presence of a magnetic field as a result of heat radiation
Introduction.
Natural convection is vital in various engineering applications, including heat transfer in electronic devices, energy-efficient building design, and thermal management in industrial processes. To design and optimize such systems, it is important to understand and predict the fluid flow and heat transfer properties in complex geometries 1 , 2 , 3 , 4 , 5 , 6 .
Several studies have focused on the influences of magnetic fields on heat transfer and fluid flow in square wavy cavities. Researchers have examined the influence of different parameters, for instance, magnetic field strength, orientation, and boundary conditions, on the convective heat transfer process. Sreedevi et al. 7 examined the behavior of nanofluid in a square cavity considering magnetic field and thermal radiation. The finite difference approach was used to solve the governing equations. They found that the maximum average value of the Nusselt number can be obtained with higher values of the Rayleigh number. To study the natural convection in a cavity where an inclined oval heater is exposed to a magnetic field, Dogonchi et al. 8 performed a numerical investigation using the CVFEM method. According to their findings, improving the Hartmann number reduced the average Nusselt at a constant Rayleigh number. Li et al.’s research 9 concentrated on convective heat transfer in an alumina water nanofluid-filled square cavity that was angled toward the horizon. In addition to convective heat transfer, they also considered radiative heat transfer. The study also analyzed entropy production. A hot circular baffle was placed inside the cavity under a fixed horizontal magnetic field. They understood that with the increase of the Rayleigh number from 103 to 106, the pace of heat transfer improved 4.5 times, and the entropy produced also increased with the development of the Rayleigh number. Furthermore, as the magnetic field strength (Ha) increased, there was a notable decrease in both the average Nusselt number (Nu) and the amount of entropy generated. Specifically, the Nu decreased by 45%, while the generated entropy diminished by 35%. In order to study the entropy generation and heat transfer in a porous corrugated cavity filled with an integrated nanofluid under the influence of natural convection, Dogonchi et al. 10 conducted a numerical study using the finite element method (FEM). This study shows that raising the Rayleigh number (Ra), and Darcy number (Da) is necessary to raise the thermal-natural convection rate. The results showed that complete irreversibility is established with Ra and Da, while it is reduced with Ha. Also, this analysis provides valuable insights into entropy production and convective heat transfer characteristics in such complex systems. Jalili et al. 11 , 12 , 13 , 14 , 15 , 16 , 17 did several works using the FEM method in this field. Mahmoudi 18 conducted theoretical and numerical research to investigate natural convection heat transfer in a porous cavity exposed to a magnetic field. This study used the scale analysis method to create a correlation to predict the heat transfer. A numerical study was also conducted using the Lattice Boltzmann method to validate the theoretical findings. The numerical simulations’ results were consistent with the theoretical predictions, indicating the proposed correlation’s correctness and reliability. This research contributes to a better understanding of heat transfer in porous cavities with magnetic field effects. Izadi et al. 19 examined the influence of two non-constant magnetic sources on the natural convection of a magnetic nanofluid in a porous medium. They discovered that as the intensity ratio between the two magnetic sources grew along with a rise in the Rayleigh (Ra) numbers, the Nusselt number initially dropped. This suggests that the convection rate decreases when the magnetic field strength becomes more asymmetric. It highlights the complex interaction between magnetic fields and natural convection in porous media and provides further insights into the behavior of such systems. Massoudi et al. 20 studied the physical properties of natural convection and radiation heat transfer in a nonagon cavity with a variable magnetic field length in a porous medium with nanofluid exposed to a uniform magnetic field. In order to find out how metal foam and rotation angle affected the natural convection of nanofluids in a hollow exposed to a magnetic field, Qi et al. 21 conducted an experiment. Their research demonstrated that a horizontal magnetic field lessens the cavity’s heat transfer, but a vertical direction improves it. The horizontal magnetic field with more force brings down the number of Nusselt, and the vertical magnetic field with higher strength enhances heat transfer, resulting in a larger Nusselt number. The numerical examination of natural convection in an F-shaped cavity with a horizontal periodic magnetic field containing non-Newtonian silver nanofluid in a porous medium was conducted by AK Hussein et al. 22 . It was noticed that heat transfer improves with increasing solid volume fraction (φ), Darcy number (Da), and Rayleigh number (Ra). Izadi et al. 23 numerically studied nanofluid’s natural convection in a porous medium influenced by a nonuniform magnetic field. Buoyancy forces, Lorentz forces, and magnetism influence the hybrid nanofluid. They found that in a porous medium at high Da, the Nusselt number falls as the porosity coefficient rises, but the Nusselt number is unaffected by the porosity coefficient of the porous medium at low and high values of Da and Ra, respectively. At low Rayleigh numbers, with the change of porosity and permeability coefficient, natural convection heat transfer change is not noticeable. Hashemi et al. 24 studied the natural convection of micropolar copper–water nanofluid in a porous chamber that produces heat. Their investigation focused on the thermal and dynamic characteristics of the nanofluid in a square chamber, where heat is produced in both the fluid and solid phases of the porous medium. They employed the Galerkin finite element method with a nonuniform structured grid to solve the governing equations and the Darcy model to simulate the flow dynamics. Finally, they showed the effect of various dimensionless parameters on velocity, temperature, and rotation. Fenghua Li et al. 25 explored the flow behavior of hybrid nanomaterials inside a permeable cavity under the influence of magnetic force. They considered the effects of permeability and external force in the Navier–Stokes equations to simulate the free convection of hybrid nanomaterials. They also evaluated the effect of various factors such as Darcy number, Hartmann number, and radiation on the fluid flow. The steady-state flow of magnetized nanofluid in the wavy cavity with radiation was examined by Nong et al. 26 . The control volume finite element (CVFE) technique is used to estimate numerical modeling. The behavior of streamlines, average Nusselt number, and isotherms was shown to be affected by magnetic force, Rayleigh number, porosity coefficient, and shape factors of nanoparticles according to them. The natural convection of a micropolar fluid was examined by Nikita et al. 27 . They reviewed their study in the wave cavity. They discussed how the flow patterns, temperature fields, and average Nusselt number in the hot corrugated wall were affected by various characteristics, including the Rayleigh number, Prandtl number, wave number, and vortex viscosity parameter. Their work is based on partial differential equations constructed in non-dimensional variables and solved with second-order precision using a finite difference approach. Mahmoud et al. 28 focused on investigating fluid flow and heat transfer in a porous media when subjected to a magnetic field. They investigated the effect of different parameters such as Darcy number, porosity parameter, radiation parameter, and Richardson number on heat transfer and flow characteristics. They applied numerical techniques and the Galerkin weighted residual finite element approach to solve the governing equations. According to their findings, there is a positive correlation between these factors and heat transfer, while an increase in the slant angle of the magnetic field causes a slight increase in velocity. In an effort to improve heat transfer, Tusi et al. 29 examined the natural motion of a water fluid containing nanocopper particles in a square cavity that was partially filled with porous media. They utilized the Darcy-Brinkman-Forchheimer relationship for fluid flow via porous media and the two-phase mixture model for simulating nanofluid flow. Additionally, they looked into how fluid flow and heat transfer were affected by the concentration of nanoparticles, Rayleigh and Darcy numbers, and the thickness ratio of the porous layer. Geridonmez et al. 30 , 31 , 32 investigated the mathematical analysis of natural convection flow in a square cavity that is exposed to a constant magnetic field. The RBF method for spatial derivatives and the backward Euler method for time derivatives are used to discretize the governing dimensionless equations. The findings demonstrate that the Lorentz force significantly reduces fluid flow and heat transfer in the affected area. In summary, this study provides significant findings regarding the aforementioned aspects. Convective heat transfer is decreased, and fluid flows more slowly as the influence area grows because of the increase in Lorentz force. As they concluded, the applied magnetic field is capable of controlling fluid flow and heat transfer. In an effort to enhance heat transfer, Several investigations were carried out to examine a cavity under various circumstances when a magnetic field was present 33 , 34 , 35 , 36 , 37 , 38 , 39 , 40 , 41 , 42 , 43 , 44 , 45 , 46 , 47 . Alsabery et al. 48 , 49 , 50 did several works in the field of heat transfer inside the porous cavity.
This paper explores the natural convection in a square, wavy, porous cavity with a partial magnetic field. Our objective is to analyze the fluid flow patterns and heat transfer behavior within the cavity through numerical simulations and mathematical modeling. This study supports existing knowledge by providing information on the influence of external factors, such as magnetic fields and porous media, on natural convection phenomena.
Problem formulation
A cavity with porous material and a partially imposed magnetic field is being considered for a laminar, incompressible natural convection flow. The configuration of the problem can be seen in Fig. 1 . The cavity’s top and bottom walls are thermally insulated, while the left wall is the heat source (T = T h ), and the right wavy wall is the cold boundary (T = T c ). The cavity’s length (L) and height (H) are in unity. The values of the parameters related to the right wall are: a = 0.9, b = 0.1, and \(k{\prime}\) is the wave number. The porous material inside the cavity is uniform and has isotropic properties. The fluid and porous material reach a local thermal equilibrium. The current analysis does not account for the effects of radiation effects, viscous dissipation, Joule heating, or the induced magnetic field.
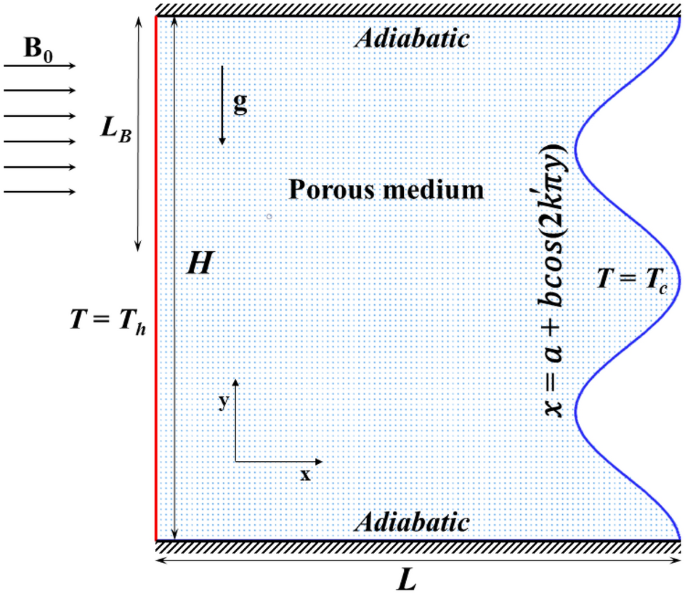
Definition of problem geometry.
Darcy’s law alone may not be sufficient in various scenarios, such as when dealing with more porous materials, high velocities, or high Reynolds number effects. In 1901, Forchheimer introduced the concept of a quadratic drag term (also known as the inertial or Forchheimer term) to Darcy’s law. Later, Brinkmann further expanded on the model to incorporate larger porosity and account for viscous effects. It’s worth mentioning that this study excludes certain factors, such as induced magnetic fields and Joule heating.
Brinkman-Forchheimer-extended Darcy’s model is utilized in this study to analyze the porous material within the cavity. The problem’s configuration involves a laminar, incompressible natural convection flow with a partially applied magnetic field. This study’s governing equations are the continuity, momentum, and energy expressed in the u-v-p-T form. These nonlinear equations capture the complex dynamics of the system and provide insights into the fluid flow, pressure distribution, and temperature distribution within the porous material-filled cavity, as follows 31 :
In which \(\mu \) corresponds to the dynamic viscosity of the fluid, the effective dynamic viscosity is \({\mu }_{e}\) , the fluid’s density is \({\rho }_{f}\) , the norm of the velocity vector \(\sqrt{({u}^{2}+{v}^{2})}\) is \(\left|\mathbf{u}\right|\) , \(g\) is the gravitational acceleration, the thermal expansion coefficient is \(\beta \) , the pressure is defined by \(p\) , the porosity of a porous medium is measured by \({\epsilon }_{p}\) , the magnitude of the applied magnetic field is referred to as \({B}_{0}\) , the fluid’s electrical conductivity is defined as σ, the effective thermal diffusivity is \({{\alpha }}_{e}=\frac{{k}_{e}}{{({\rho }_{f}{c}_{p})}_{f}}\) , the effective thermal conductivity is \({k}_{e}={\epsilon }_{p}{k}_{f}+(1-{\epsilon }_{p}){k}_{s}\) , the specific heat at constant pressure is \({c}_{p}\) , the coefficient of form is \({c}_{F}=\frac{1.75(1-{\epsilon }_{p})}{{d}_{p}{{\epsilon }_{p}}^{3}}\) , \(K=\frac{{{d}_{p}}^{2}{{\epsilon }_{p}}^{3}}{150{(1-{\epsilon }_{p})}^{2}}\) refers to the permeability of the porous medium, \({d}_{p}\) is the size of a solid particle in a porous medium, and the heat capacity ratio \({\upsigma }_{h}=\frac{{\epsilon }_{p}{({\rho }_{f}{c}_{p})}_{f} +(1-{\epsilon }_{p}){({\rho }_{f}{c}_{p})}_{s}}{{({\rho }_{f}{c}_{p})}_{f}}\) , is considered one in this model. Additionally, the fluid \((f)\) and solid \((s)\) are assumed to have the same thermal conductivity and thermal diffusivity. That means, \({k}_{e}={k}_{f}={k}_{s}\) and \({\alpha }_{e}={\alpha }_{f}=\alpha \) . In addition, \({\mu }_{e}=\mu \) is assumed. Air fluid was used for the present work. The physical properties of air are given in Table 1 .
The boundary conditions are outlined below:
The dimensionless quantities are defined below:
With the help of non-dimensional quantities, they are replaced in the original Eqs. ( 1 )-( 4 ), and assuming the elimination of prime symbols, the following dimensionless equations are obtained.
where, \({c}_{g}=\frac{ 1.75}{\sqrt{150}{{\epsilon }_{p}}^{3/2}}\) . The dimensionless parameters Prandtl, Darcy, Rayleigh, and Hartmann numbers are as follows:
where \(\Delta T={T}_{h}-{T}_{c}\) . By setting the stream function \(\psi \) as \(u=\partial \psi /\partial y, v=-\partial \psi /\partial x\) , it eliminates the continuity equation due to satisfying the continuity condition, and the pressure term is removed by using the vorticity definition \(\omega =\nabla \times \mathbf{u}\) in the momentum equations. Non-dimension equations are deduced from the effects of stream and vorticity functions:
In the case of the incoming magnetic field, \({\delta }_{B}\) , is defined as:
The reduced boundary conditions are as follows:
The average Nusselt number for the warm wall is determined as follows:
Method of solution and numerical results
The Eqs. ( 12 )-( 14 ) have been solved using the Finite Element Method with boundary conditions (16). The reliability of this method is a result of its high strength and flexibility. Once the initial meshing is done, the solution continues continuously. To achieve acceptable accuracy, it may also alter the mesh structure. This process continues until the convergence condition is met. The convergence condition for the present work is to reach 10 –5 accuracy. The Finite Element Method (FEM) operates on a fundamental principle: breaking down a complex problem domain into discrete sub-regions that are called finite elements. Each of these elements possesses a distinct geometry and is characterized mathematically through a set of equations that describe the behavior of the system within that particular section. This approach simplifies the solution of complex governing equations, which would be arduous or nearly impossible to solve manually. Shape functions are used in FEM to interpolate element-level solutions based on nodal values. In this study, FEM serves as a numerical tool for solving the governing equations related to fluid flow and heat transfer due to natural convection of a square wavy cavity with a magnetic field and porous media. To improve understanding of the numerical solution technique, Fig. 2 displays the flowchart of the numerical method. Through FEM, a more precise and detailed solution for the natural convection of a square wavy cavity with a magnetic field and porous media can be obtained. Figure 3 shows a good match between the streamlines and isotherm lines compared to the work of Geridonmez et al. 31 .
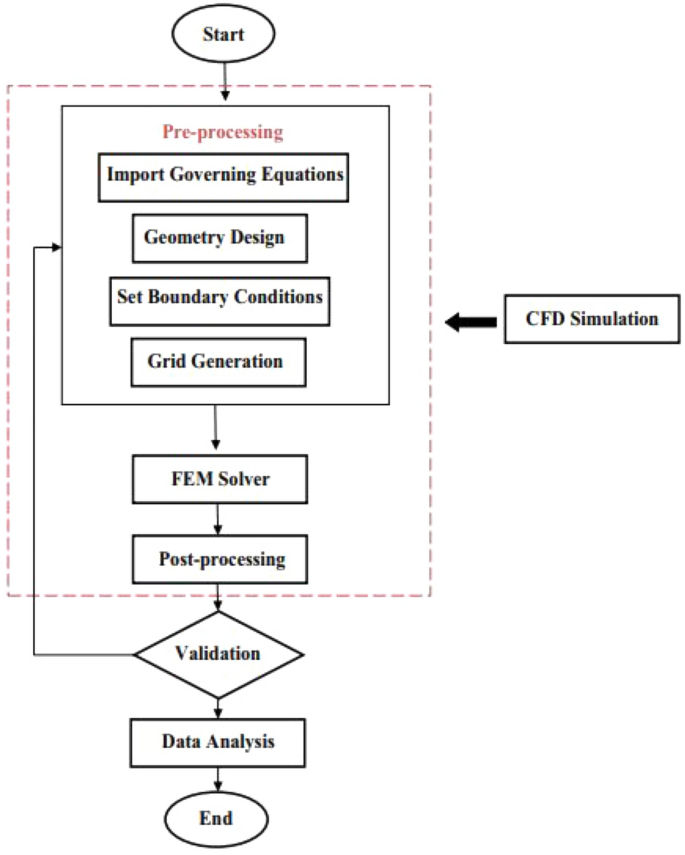
The flowchart of the numerical method.

Comparison of streamlines (on the left) and isotherm lines (on the right) at Ra = 10 5 , Da = 0.01, ϵ p = 0.9, Ha = 50, L B = 0.7.
In order to obtain a mesh-independent solution, mesh independence is investigated. The grid-independent solution was examined by providing a solution for natural convection in a square cavity with one side having a cosine wave with a magnetic field applied to a portion of the square side. Five of the grids have been tested. As can be seen in Table 2 , with the number of cells 3006, the solution becomes independent and reaches an accuracy of 10 –5 . The optimal mesh is shown in Fig. 4 .
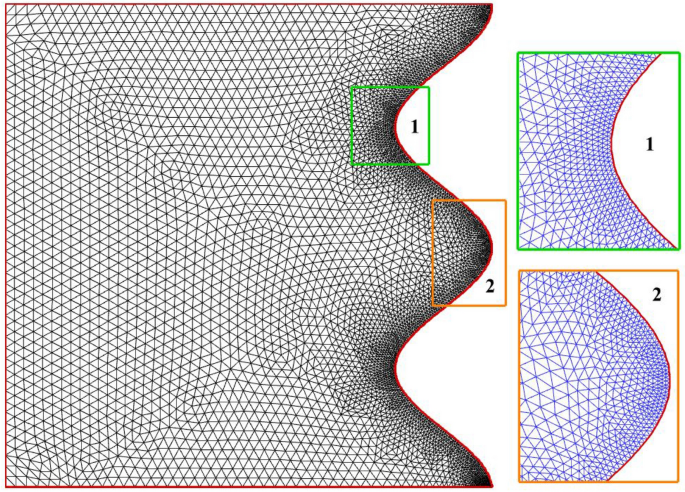
Grid of geometry.
Results and discussions
Figure 5 shows the impact of the entranced magnetic field length on the left wall. As the magnetic field length increases, the main vortex is compressed towards the lower left nook of the cavity, but the upper part of the vortex is stretched towards the upper right nook of the cavity because of the increased Lorentz force area introduced by the magnetic field. The vortex’s strength decreases with the magnetic field’s length. On the other hand, the buoyancy force slows down. The temperature difference in the isotherms decreases. In addition, the isotherm lines tend to flatten, indicating the magnetic force’s inhibition of natural convection.
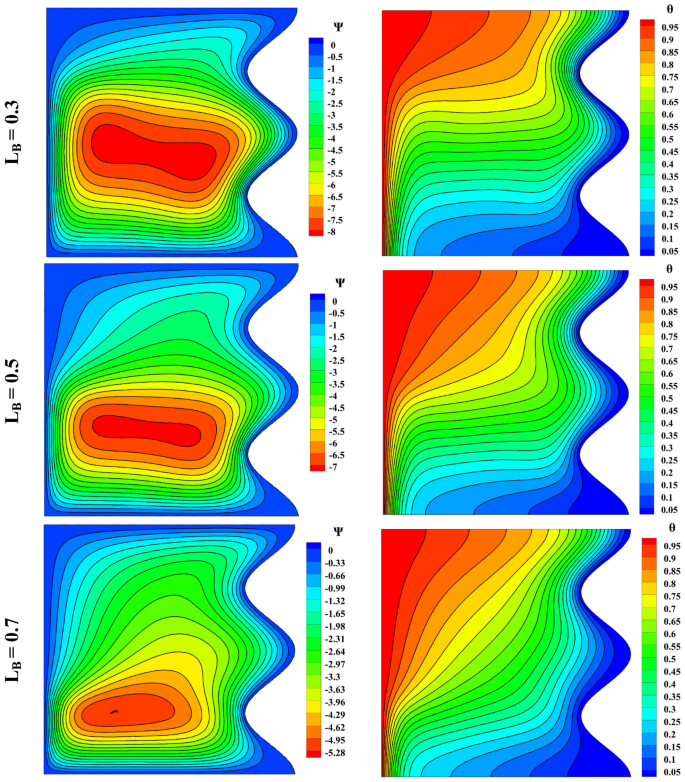
Impact of magnetic field length on streamlines and isotherm lines with Ra = 10 5 , Da = 0.01, ϵ p = 0.9, Ha = 50, \(k{\prime}\) = 2; from top to bottom Nu = 5.127, 4.245, 3.247, respectively.
The velocity and temperature distribution based on the variable magnetic field length can be seen in Fig. 6 . It is concluded that by raising the magnetic field length, the velocity decreases, and the temperature increases from the bottom of the cavity to its center, but this process is the opposite for the upper half. The maximum velocity u belongs to L B = 0.5.
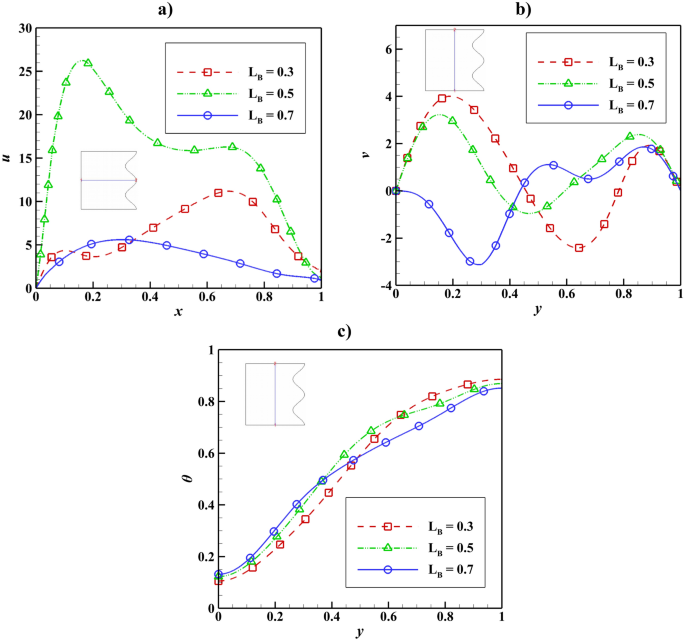
Velocity and temperature distribution based on magnetic field length change with Ra = 10 5 , Da = 0.01, ϵ p = 0.9, Ha = 50, \(k{\prime}\) = 2; ( a ) u at y = 0.5, ( b ) v at x = 0.5, ( c ) θ at x = 0.5.
Figure 7 shows the effect of the Rayleigh number on streamlines and isothermal lines. L B = 0.5, as defined, means that the magnetic field affects the left wall of the cavity from the top to the center. Therefore, the streamlines show the natural convection at the bottom of the cavity, while the upper part of the cavity shows the lagging impact of the Lorentz force. Because of the dominance of Lorentz force over the buoyancy force, the second vortex is seen in the streamlines at Ra = 10 4 , while it is not observed at Ra = 10 5 or 10 6 due to the high buoyancy force. The isotherm lines are approximately 90 degrees to the upper wall for Ra = 10 4 , 10 5 . With the increase of Rayleigh, the isotherm lines tend to bend more from the flat state, and heat penetrates more in the upper half.
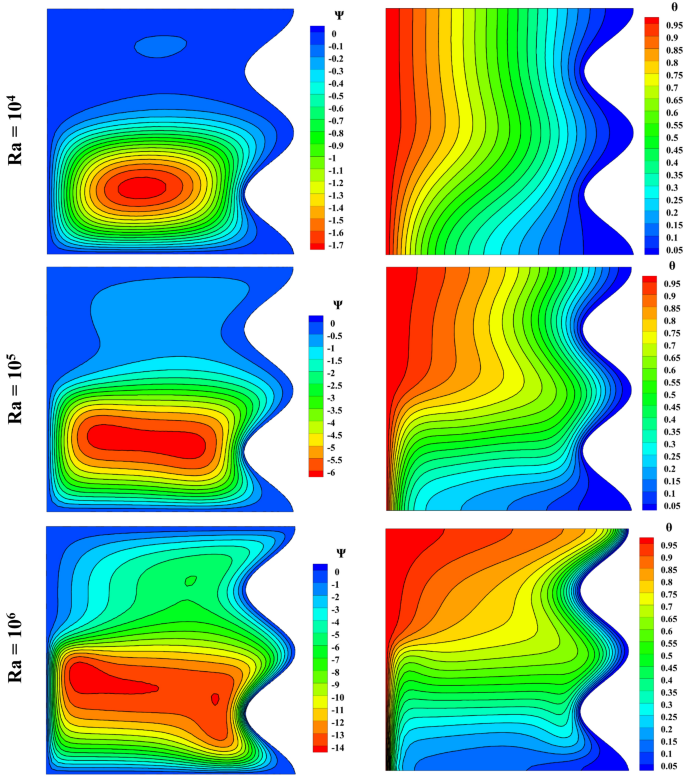
Influence of Rayleigh number on streamlines and isotherm lines with Da = 0.01, ϵ p = 0.9, Ha = 100, L B = 0.5, \(k{\prime}\) = 2; from top to bottom Nu = 1.465, 3.6, 8.944, respectively.
The velocity and temperature distribution based on the Rayleigh number change can be seen in Fig. 8 . Raising the Rayleigh number lowered the temperature in the cavity’s lower region while increasing the maximum velocity, but this trend changes for the upper part of the cavity, and the temperature increases.

Velocity and temperature distribution based on Rayleigh number change with Da = 0.01, ϵ p = 0.9, Ha = 100, L B = 0.5, \(k{\prime}\) = 2; ( a ) u at y = 0.5, ( b ) v at x = 0.5, ( c ) θ at x = 0.5.
Figure 9 shows the effect of the Hartmann number on streamlines and isothermal lines. In case there’s no magnetic field due to the non-existence of Lorentz force delay, the streamlines show natural convection in all parts of the cavity. With the augmentation of the Hartmann number, the vortex tends to the lower side of the cavity. Also, the increase in Hartmann reduces the strength of the vortex.
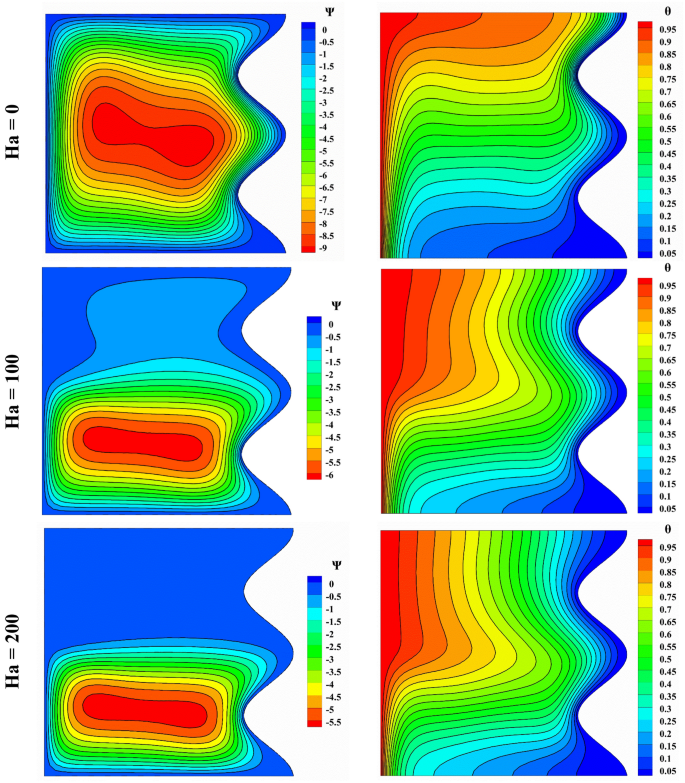
Impact of Hartmann number on streamlines and isotherm lines with Da = 0.01, ϵ p = 0.9, Ra = 10 5 , L B = 0.5, \(k{\prime}\) = 2; from top to bottom Nu = 5.973, 3.6, 3.342, respectively.
The isotherm lines are almost perpendicular to the top wall for Ra = 10 5 , 10 6 . As the Rayleigh increases, the isotherm lines tend to bend less from the flat state, and heat penetrates less in the upper half, indicating that the magnetic field was controlling the heat transfer and fluid flow.
The velocity and temperature distribution due to the Hartmann number change is shown in Fig. 10 . In the absence of a magnetic field, u has the lowest value, and with the augmentation of the Hartmann number, u and v decrease. With increasing the Hartmann number, the temperature trend for the cavity’s lower half increases, but this trend is reversed for the upper half, and the temperature decreases.

Velocity and temperature distribution based on Hartmann number change with Da = 0.01, ϵ p = 0.9, Ra = 10 5 , L B = 0.5, \(k{\prime}\) = 2; ( a ) u at y = 0.5, ( b ) v at x = 0.5, ( c ) θ at x = 0.5.
The influence of Darcy number on streamlines and isotherm lines was examined, and it is shown as a contour in Fig. 11 . At the Darcy number close to zero, the vorticity tends to the center, and the isothermal lines are almost parallel to each other because the Lorentz force loses its effect at a low Darcy number. With the increase of Darcy, the penetration of heat increases, and as a result, the fluid moves at a higher speed, and the vortex is transferred downwards.

Impact of Darcy number on streamlines and isotherm lines with Ra = 10 5 , ϵ p = 0.9, Ha = 50, L B = 0.5, \(k{\prime}\) = 2; from top to bottom Nu = 1.234, 2.404, 4.245, respectively.
The velocity and temperature distribution due to the Darcy number change can be seen in Fig. 12 . With increasing the Darcy number, the magnitude of u and v increase, and it can be said that the temperature also has an increasing trend, and in Darcy 10 –4 , the temperature changes are insignificant.

Velocity and temperature distribution based on Darcy number change with Ra = 10 5 , Ha = 50, ϵ p = 0.9, L B = 0.5, \(k{\prime}\) = 2; ( a ) u at y = 0.5, ( b ) v at x = 0.5, ( c ) θ at x = 0.5.
The impact of wave number on streamlines and isotherm lines was studied, and the outcomes are shown in Fig. 13 . As the wave number increases, the eddy tends to rise less, and the fluid moves with a higher partial velocity. The number of waves has no noticeable effect on isothermal lines. Nonetheless, there is a certain increase in the Nusselt number as the wave number rises. The behavior of velocity in horizontal and vertical, as well as temperature distribution in the vertical case, is shown. See Fig. 14 for better understanding.

Impact of wave number on streamlines and isotherm lines with Ra = 10 5 , Da = 0.01, ϵ p = 0.9, Ha = 50, L B = 0.5; from top to bottom Nu = 3.978, 4.093, 4.245, 4.243, respectively.
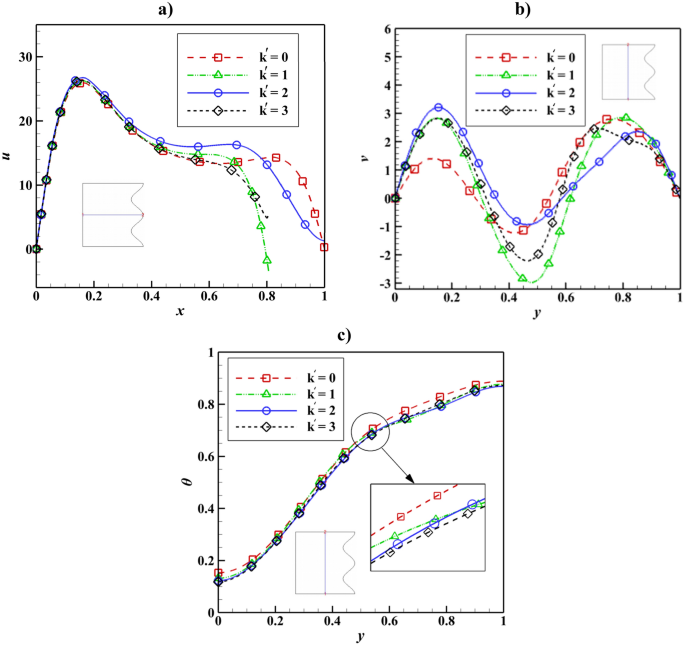
Velocity and temperature distribution based on Wave number change with Ra = 10 5 , Ha = 50, Da = 0.01, ϵ p = 0.9, L B = 0.5; a) u at y = 0.5, b) v at x = 0.5, c) θ at x = 0.5.
Average Nusselt is influenced by magnetic field length, Rayleigh, Hartmann, and Darcy number, as shown in Fig. 15 . The average Nusselt is proportional to the Rayleigh and Darcy numbers and increases with the increase of Rayleigh and Darcy. At the same time, it has an inverse relationship with the Hartmann number and the length of the magnetic field. Figures 16 and 17 show the simultaneous effect of magnetic field length, Rayleigh number, and Hartmann and Darcy numbers on the average Nusselt. The average Nusselt number reached its highest point when there was no magnetic field, and the Rayleigh and Darcy numbers were high.
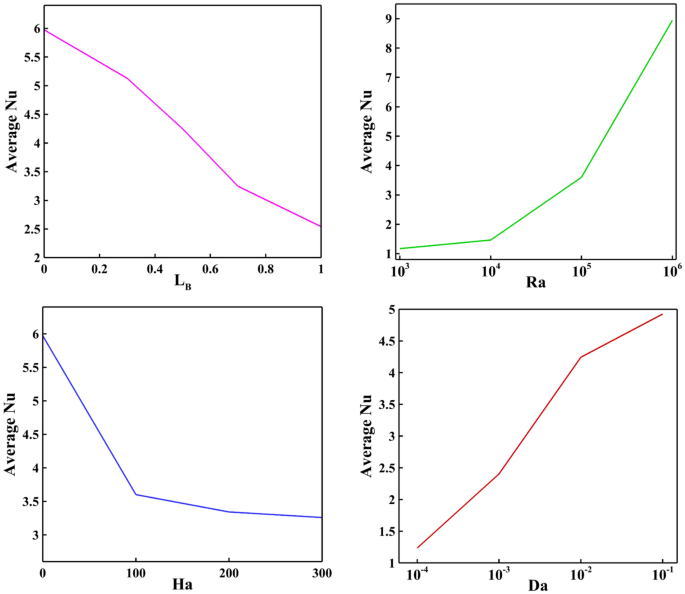
Impact of magnetic field length, Rayleigh, Hartmann, and Darcy numbers on Average Nusselt with Ra = 10 5 , Da = 0.01, ϵ p = 0.9, Ha = 50, L B = 0.5, \(k {\prime}\) = 2.
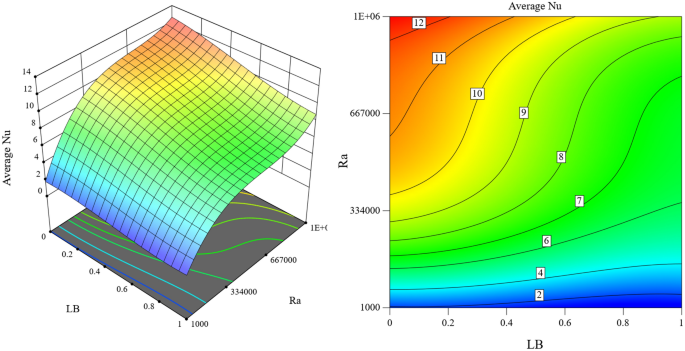
Simultaneous effect of magnetic field length and Rayleigh number on average Nusselt number with Da = 0.01, ϵ p = 0.9, Ha = 50, \(k\) = 2.
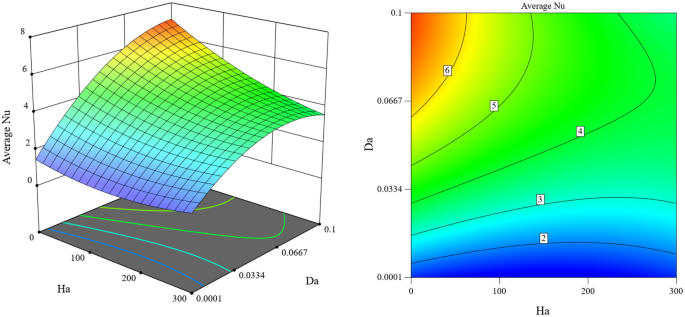
Simultaneous impact of Hartmann and Darcy numbers on average Nusselt number with Ra = 10 5 , ϵ p = 0.9, L B = 0.5, \(k\) = 2.
In summary, the set of average Nusselt number and maximum psi value is given in Table 3 .
This paper presents the results of a numerical study on natural convection in a square wavy cavity in the presence of a partial magnetic field under effective factors such as magnetic field length (L B ), Rayleigh number (Ra), Hartmann number (Ha), Darcy number (Da) and wave number ( \(k{\prime}\) ). The effective parameters were investigated in L B = 0.3–0.7, Ra = 10 4 –10 6 , Da = 0.0001–0.01, \(k{\prime}\) = 0–3 intervals. In addition, the distribution of temperature, average Nusselt, streamlines, and isotherm lines were shown for the cavity. The most important results obtained are as follows:
As the magnetic field’s length increases, heat transfer decreases. As the length of the magnetic field increases from 0.3 to 0.7, the Nusselt number decreases by 36.6%, and the value of maximum psi decreases by 34%.
As the Rayleigh number increases, the Nusselt number also increases. In fact, with the increase of the Rayleigh number, the buoyancy force increases, and the buoyancy force increases the heat transfer and fluid velocity. As the Rayleigh number increases from 10 4 to 10 6 , the Nusselt number increases more than five times, and the value of maximum psi increases more than seven times.
In the lower Rayleigh, the second vortex is formed due to the dominance of the Lorentz force over the buoyancy force.
The effect of Lorentz force on fluid flow and heat transfer increases at a high Rayleigh number.
Nusselt number decreases with increasing Hartmann number. As the Hartmann number increases, the Lorentz force increases. Lorentz force reduces heat transfer and fluid velocity. As the Hartmann number increases from 0 to 200, the Nusselt number decreases by 44%, and the value of maximum psi decreases by 38.8%.
By increasing the permeability in the porous medium, heat transfer is improved. As the Darcy number increases from 0.0001 to 0.01, the Nusselt number increases more than two times, and the value of maximum psi increases more than nine times.
At low Darcy numbers, the magnetic field becomes ineffective.
As the wave number of the right wall increases, the Nusselt number increases due to the increase of the heat transfer area. As the wave number increases from 0 to 2, the Nusselt number increases by 6.71%, and the value of maximum psi increases by 1.3%.
Data availability
The datasets used and/or analysed during the current study available from the corresponding author on reasonable request.
Abbreviations
Prandtl number
Rayleigh number
Hartmann number
Darcy number
Specific heat, J.kg -1 .K -1
Thermal conductivity, W.m −1 .K −1
Permeability,m 2
Temperature, K
Gravitational acceleration
Length of the cavity, m
Height of the cavity, m
Wave number
Magnetic field length
Average Nusselt number
Velocity, m.s -1
Dimensionless velocity
Dimensionless time
Pressure, pa
Dimensionless pressure
Magnitude of the magnetic field, N.A -1 .m 2
Cartesian coordinates,m
Dimensionless cartesian coordinates
Dimensionless temperature
Density, kg.m -3
Dynamic viscosity, Pa.S
Thermal expansion coefficient, K -1
Thermal diffusivity, m 2 .s
Dimensionless stream function
Baïri, A., Esther Z. P., & García De María, J. M.. A review on natural convection in enclosures for engineering applications. The particular case of the parallelogrammic diode cavity. Appl. Thermal Eng. 63 (1), 304–322 (2014).
Asghar, Z., Muhammad, W. S. K., Amjad, A. P., Mustafa, M. R., Sankaralingam, L., & Mohammad, I. A. On non-Newtonian fluid flow generated via complex metachronal waves of cilia with magnetic, hall, and porous effects. Phys. Fluids 35 (9) (2023).
Umavathi, J. C., & Mahanthesh, B. Study of multilayer flow of two immiscible nanofluids in a duct with viscous dissipation. Phys. Fluids 35 (9) (2023).
Parmar, D., Rathish Kumar, B. V., Krishna Murthy, S. V., & Sumant, K. Numerical study of entropy generation in magneto-convective flow of nanofluid in porous enclosure using fractional order non-Darcian model. Phys. Fluids 35 (9) (2023).
Rath, C., & Anita, N. A numerical study on MHD Cu-Al2O3/H2O hybrid nanofluid with Hall current and cross-diffusion effect. Phys. Fluids 35 (10) (2023).
Rahimi, A., Ali, D. S., Abbas K., & Emad, H. M. A comprehensive review on natural convection flow and heat transfer: the most practical geometries for engineering applications. Int. J. Numer. Methods Heat Fluid Flow 29 (3), 834–877 (2019).
Sreedevi, P., & Sudarsana Reddy, P. Effect of magnetic field and thermal radiation on natural convection in a square cavity filled with TiO2 nanoparticles using Tiwari-Das nanofluid model. Alexandria Eng. J. 61 (2), 1529–1541 (2022).
Dogonchi, A. S., Armaghani, T., Chamkha, Ali J. & Ganji, D. D. Natural convection analysis in a cavity with an inclined elliptical heater subject to shape factor of nanoparticles and magnetic field. Arabian J. Sci. Eng. 44 (9), 7919–7931. https://doi.org/10.1007/s13369-019-03956-x (2019).
Article CAS Google Scholar
Li, Z., Ahmed, K. H., Obai, Y., Masoud, A., & Shizhe, F. Natural convection and entropy generation of a nanofluid around a circular baffle inside an inclined square cavity under thermal radiation and magnetic field effects. Int. Commun. Heat Mass Transf. 116 , 104650 (2020).
Sattar Dogonchi, A., Tayebi, T., Karimi, N., Chamkha, A. J. & Alhumade, H. Thermal-natural convection and entropy production behavior of hybrid nanoliquid flow under the effects of magnetic field through a porous wavy cavity embodies three circular cylinders. J. Taiwan Inst. Chem. Eng. 124 , 162–173. https://doi.org/10.1016/j.jtice.2021.04.033 (2021).
Jalili, P., Afifi, M. D., Jalili, B., Mirzaei, A. M. & Ganji, D. D. Numerical study and comparison of two-dimensional ferrofluid flow in semi-porous channel under magnetic field. Int. J. Eng. 36 (11), 2087–2101. https://doi.org/10.5829/IJE.2023.36.11B.13 (2023).
Mirzaei, A., Jalili, P., Afifi, M. D., Jalili, B. & Ganji, D. D. Convection heat transfer of MHD fluid flow in the circular cavity with various obstacles: Finite element approach. Int. J. Thermofluids 20 , 100522. https://doi.org/10.1016/j.ijft.2023.100522 (2023).
Article Google Scholar
Jalili, P., Mohammad, D. A., Amirmohammad, M., Bahram, J., & Davood, D.G. Study of ferrofluid flow with Lorentz force in the porous channel in the presence of transversely magnetic field. Int. J. Eng. (2023).
Dehghan, A., et al . The effects of thermal radiation, thermal conductivity, and variable viscosity on ferrofluid in porous medium under magnetic field. World J. Eng . (2024).
Jalili, P. et al. Analytical and numerical investigation of thermal distribution for hybrid nanofluid through an oblique artery with mild stenosis. SN Appl. Sci. 5 (4), 95 (2023).
Jalili, P., et al . Thermal analysis of transverse fluid flow in a gradient porous media with the exponentially boundary conditions. Mod. Phys. Lett. B 2350229 (2023).
Bahmani, M. et al. Effect of variations hollow of octagon porous media on heat and mass transfer. Int. J. Thermofluids 21 , 100576 (2024).
Mahmoudi, A. A scale analysis for natural convection in a porous media in the presence of a magnetic field. J. Taiwan Inst. Chem. Eng. 95 , 21–31 (2019).
Izadi, M., Rasul, M., Amin, A. D. & Hasan, S. Natural convection of a magnetizable hybrid nanofluid inside a porous enclosure subjected to two variable magnetic fields. Int. J. Mech. Sci. 151 , 154–169 (2019).
Massoudi, M. D., Mohamed, B. B. H., & Mohammed, A. A. Free convection and thermal radiation of nanofluid inside nonagon inclined cavity containing a porous medium influenced by magnetic field with variable direction in the presence of uniform heat generation/absorption. Int. J. Numer. Methods Heat Fluid Flow 31 (3), 933–958 (2021).
Qi, C. et al. Effects of rotation angle and metal foam on natural convection of nanofluids in a cavity under an adjustable magnetic field. Int. Commun. Heat Mass Transfer 109 , 104349 (2019).
Hussein, A. K. et al. Natural convection in F-shaped cavity filled with Ag-water non-Newtonian nanofluid saturated with a porous medium and subjected to a horizontal periodic magnetic field. Korean J. Chem. Eng. 39 (4), 887–901 (2022).
Izadi, M. et al. LTNE modeling of Magneto-Ferro natural convection inside a porous enclosure exposed to nonuniform magnetic field. Phys. A Stat. Mech. Appl. 535 , 122394 (2019).
Article MathSciNet CAS Google Scholar
Hashemi, H., Zafar, N. & Mehryan, S. A. M. Cu-water micropolar nanofluid natural convection within a porous enclosure with heat generation. J. Mol. Liquids 236 , 48–60 (2017).
Li, F. et al. "Free convection simulation of hybrid nanomaterial in permeable cavity with inclusion of magnetic force. J. Mol. Liquids 335 , 116170 (2021).
Nong, H. et al. Numerical modeling for steady-state nanofluid free convection involving radiation through a wavy cavity with Lorentz forces. J. Mol. Liquids 336 , 116324 (2021).
Gibanov, N. S., Sheremet, M. A. & Pop, I. Natural convection of micropolar fluid in a wavy differentially heated cavity. J. Mol. Liq. 221 , 518–525 (2016).
Mehmood, K., Hussain, S. & Sagheer, M. Numerical simulation of MHD mixed convection in alumina–water nanofluid filled square porous cavity using KKL model: Effects of nonlinear thermal radiation and inclined magnetic field. J. Mol. Liq. 238 , 485–498 (2017).
Toosi, M. H. & Majid, S. Two-phase mixture numerical simulation of natural convection of nanofluid flow in a cavity partially filled with porous media to enhance heat transfer. J. Mol. Liquids 238 , 553–569 (2017).
Geridonmez, Pekmen, B. & Oztop, H. F. MHD natural convection in a cavity in the presence of cross partial magnetic fields and Al2O3-water nanofluid. Comput. Math. Appl. 80 (12), 2796–2810 (2020).
Article MathSciNet Google Scholar
Geridonmez, G., Pekmen, B. & Oztop, H. F. Natural convection in a cavity filled with porous medium under the effect of a partial magnetic field. Int. J. Mech. Sci. 161 , 105077 (2019).
Pekmen Geridonmez, B. & Oztop, H. F. Natural convection in a cavity under partial magnetic field applied from different corners. Int. Commun. Heat Mass Trans. 114 , 104575. https://doi.org/10.1016/j.icheatmasstransfer.2020.104575 (2020).
Selimefendigil, F. & Öztop, H. F. Effects of conductive curved partition and magnetic field on natural convection and entropy generation in an inclined cavity filled with nanofluid. Phys. A 540 , 123004 (2020).
Hussain, S., Shoeibi, S. & Armaghani, T. Impact of magnetic field and entropy generation of Casson fluid on double diffusive natural convection in staggered cavity. Int. Commun. Heat Mass Trans. 127 , 105520 (2021).
Li, Z. et al. Simulation of natural convection of Fe 3 O 4-water ferrofluid in a circular porous cavity in the presence of a magnetic field. Eur. Phys. J. Plus 134 , 1–8 (2019).
Afrand, M. et al. Free convection and entropy generation of a nanofluid in a tilted triangular cavity exposed to a magnetic field with sinusoidal wall temperature distribution considering radiation effects. Int. Commun. Heat Mass Trans. 112 , 104507 (2020).
Saleem, S., et al . Steady laminar natural convection of nanofluid under the impact of magnetic field on two-dimensional cavity with radiation. AIP Adv. 9 (6) (2019).
Belhaj, S. & Ben-Beya, B. Thermal performance analysis of hybrid nanofluid natural convection in a square cavity containing an elliptical obstacle under variable magnetic field. Int. J. Numer. Meth. Heat Fluid Flow 32 (6), 1825–1860 (2022).
Rahman, M. M., Pop, I. & Saghir, M. Z. Steady free convection flow within a titled nanofluid saturated porous cavity in the presence of a sloping magnetic field energized by an exothermic chemical reaction administered by Arrhenius kinetics. Int. J. Heat Mass Transf. 129 , 198–211 (2019).
Mourad, A., Aissa, A., Mebarek-Oudina, F., Al-Kouz, W. & Sahnoun, M. Natural convection of nanoliquid from elliptic cylinder in wavy enclosure under the effect of uniform magnetic field: Numerical investigation. Eur. Phys. J. Plus 136 (4), 429 (2021).
Abderrahmane, A. et al. Investigation of the free convection of nanofluid flow in a wavy porous enclosure subjected to a magnetic field using the Galerkin finite element method. J. Magnetism Mag. Mater. 569 , 170446. https://doi.org/10.1016/j.jmmm.2023.170446 (2023).
Nabwey, H. A. et al. Radiative effects on unsteady MHD natural convection flow in an inclined wavy porous cavity using hybrid nanofluid containing a square obstacle. Alexandria Eng. J. 65 , 921–937. https://doi.org/10.1016/j.aej.2022.10.004 (2023).
Salehi, M., Afshar, S. R., Ali, R. & Chamkha, A. J. Numerical investigation of TiO2-water nanofluid heat transfer in a porous wavy circular chamber with a ┴-shaped heater under magnetic field. Case Stud. Thermal Eng. 49 , 103405. https://doi.org/10.1016/j.csite.2023.103405 (2023).
Abderrahmane, A. et al. Second law analysis of a 3D magnetic buoyancy-driven flow of hybrid nanofluid inside a wavy cubical cavity partially filled with porous layer and non-Newtonian layer. Annals Nuclear Energy 181 , 109511. https://doi.org/10.1016/j.anucene.2022.109511 (2023).
Alqaed, S. et al. Entropy generation of the laminar and mixed flow of alumina/water nanofluid flow in a two-dimensional rectangular enclosure affected by a magnetic field using the lattice Boltzmann method Eng. Anal. Boundary Elements 151 (187), 198. https://doi.org/10.1016/j.enganabound.2023.03.004 (2023).
Jelodari, I. & Nikseresht, A. H. Effects of Lorentz force and induced electrical field on the thermal performance of a magnetic nanofluid-filled cubic cavity. J. Mol. Liq. 252 , 296–310 (2018).
Ahrar, A. J. & Mohammad, H. D. Lattice Boltzmann simulation of a Cu-water nanofluid filled cavity in order to investigate the influence of volume fraction and magnetic field specifications on flow and heat transfer. J. Mol. Liquids 215 , 328–338 (2016).
Alsabery, A. I., Mohebbi, R., Chamkha, A. J. & Hashim, I. Effect of local thermal non-equilibrium model on natural convection in a nanofluid-filled wavy-walled porous cavity containing inner solid cylinder. Chem. Eng. Sci. 201 , 247–263 (2019).
Alsabery, A. I., Tayebi, T., Chamkha, A. J. & Hashim, I. Effect of rotating solid cylinder on entropy generation and convective heat transfer in a wavy porous cavity heated from below. Int. Commun. Heat Mass Trans. 95 , 197–209 (2018).
Alsabery, A. I. et al. Convection heat transfer in enclosures with inner bodies: A review on single and two-phase nanofluid models. Renew. Sustain. Energy Rev. 183 , 113424 (2023).
Download references
Author information
Authors and affiliations.
Department of Mechanical Engineering, Sharif University of Technology, Tehran, Iran
Amirmohammad Mirzaei
Department of Mechanical Engineering, North Tehran Branch, Islamic Azad University, Tehran, Iran
Bahram Jalili & Payam Jalili
Department of Mechanical Engineering, Babol Noshirvani University of Technology, P.O. Box 484, Babol, Iran
Davood Domiri Ganji
You can also search for this author in PubMed Google Scholar
Contributions
A.M. and B.J. wrote the main manuscript text and P.J. and D.D.G. prepared all figures and calculations. All authors reviewed the manuscript.
Corresponding authors
Correspondence to Payam Jalili or Davood Domiri Ganji .
Ethics declarations
Competing interests.
The authors declare no competing interests.
Additional information
Publisher's note.
Springer Nature remains neutral with regard to jurisdictional claims in published maps and institutional affiliations.
Rights and permissions
Open Access This article is licensed under a Creative Commons Attribution 4.0 International License, which permits use, sharing, adaptation, distribution and reproduction in any medium or format, as long as you give appropriate credit to the original author(s) and the source, provide a link to the Creative Commons licence, and indicate if changes were made. The images or other third party material in this article are included in the article's Creative Commons licence, unless indicated otherwise in a credit line to the material. If material is not included in the article's Creative Commons licence and your intended use is not permitted by statutory regulation or exceeds the permitted use, you will need to obtain permission directly from the copyright holder. To view a copy of this licence, visit http://creativecommons.org/licenses/by/4.0/ .
Reprints and permissions
About this article
Cite this article.
Mirzaei, A., Jalili, B., Jalili, P. et al. Free convection in a square wavy porous cavity with partly magnetic field: a numerical investigation. Sci Rep 14 , 14152 (2024). https://doi.org/10.1038/s41598-024-64850-7
Download citation
Received : 26 March 2024
Accepted : 13 June 2024
Published : 19 June 2024
DOI : https://doi.org/10.1038/s41598-024-64850-7
Share this article
Anyone you share the following link with will be able to read this content:
Sorry, a shareable link is not currently available for this article.
Provided by the Springer Nature SharedIt content-sharing initiative
- Partial magnetic field
- Natural convection
- Porous medium
- Square wavy cavity
By submitting a comment you agree to abide by our Terms and Community Guidelines . If you find something abusive or that does not comply with our terms or guidelines please flag it as inappropriate.
Quick links
- Explore articles by subject
- Guide to authors
- Editorial policies
Sign up for the Nature Briefing newsletter — what matters in science, free to your inbox daily.

- About the Hub
- Announcements
- Faculty Experts Guide
- Subscribe to the newsletter
Explore by Topic
- Arts+Culture
- Politics+Society
- Science+Technology
- Student Life
- University News
- Voices+Opinion
- About Hub at Work
- Gazette Archive
- Benefits+Perks
- Health+Well-Being
- Current Issue
- About the Magazine
- Past Issues
- Support Johns Hopkins Magazine
- Subscribe to the Magazine
You are using an outdated browser. Please upgrade your browser to improve your experience.
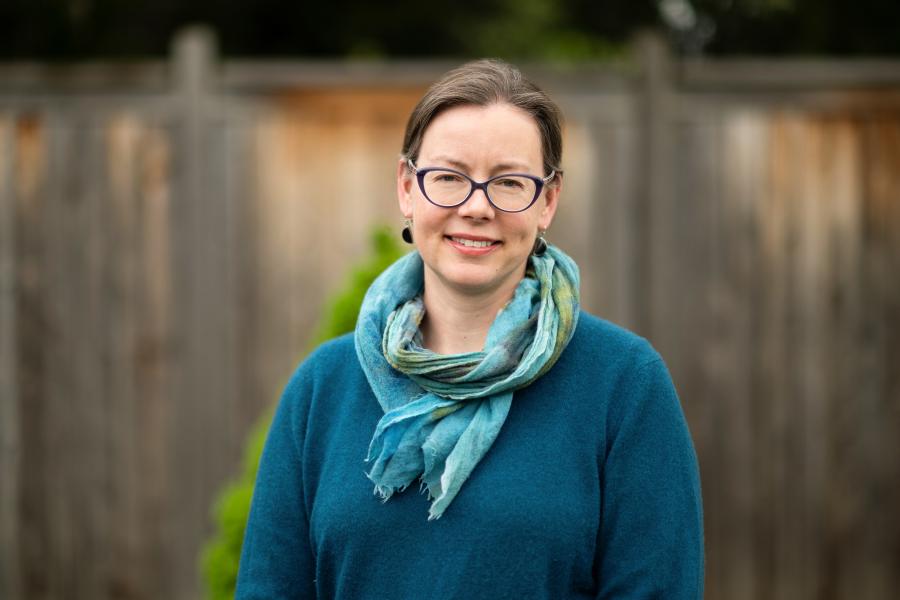
Image caption: Julie Lundquist
Julie Lundquist, leader in sustainable energy research, to join Johns Hopkins faculty
Lundquist, who researches atmospheric dynamics in hopes of making wind energy more efficient, named bloomberg distinguished professor of atmospheric science and wind energy.
By Annika Weder
When she was an undergraduate student studying English literature, Julie Lundquist added a physics major because she enjoyed math and solving physics problems—though she says they didn't excite her the way certain kinds of poetry did. That is, until a seminar during a summer internship at the National Center for Atmospheric Research. There, she was introduced to Milton Van Dyke's An Album of Fluid Motion , a book of photographs illustrating fluid dynamics, such as water tank simulations or smoke moving through a wind tunnel. Lundquist says she was "blown away" by how beautiful it was and pivoted her career to become an atmospheric scientist.
Lundquist studies atmospheric dynamics—the ways in which air flows. Her research uses observational and computational approaches to understand the atmospheric boundary layer, with an emphasis on atmosphere-wind energy interactions. The atmospheric boundary layer is the lowest part of the atmosphere closest to the Earth's surface, where communication between the air and the ground occurs, and temperature changes on the ground can affect air movement in the atmosphere. The turbulent atmospheric boundary layer usually traps pollution so that upper layers of the atmosphere are effectively insulated from the ground. Lundquist is interested in both the atmospheric consequences of wind energy deployment as well as atmospheric impacts on wind energy production.
"I want to solve these big picture problems, and knowing the work that we're doing can make an impact in the world inspires my research," Lundquist says. "Our civilization is moving so fast, and the disparities are so dramatic. If I can do something that helps make electricity more accessible to more people, in a way that doesn't cause harm, that's one step toward addressing energy challenges and global climate change while reducing pollution. And I get to solve some really fun puzzles along the way."
Lundquist, a national leader in research in sustainable energy generation from wind, will join Johns Hopkins University as a Bloomberg Distinguished Professor of Atmospheric Science and Wind Energy on July 1. She will hold primary appointments in the Department of Mechanical Engineering in the Whiting School of Engineering and in the Department of Earth and Planetary Sciences in the Krieger School of Arts and Sciences. Lundquist will also be part of the Sustainable Transformations and Energy Bloomberg Distinguished Professorships (BDP) cluster.
"Julie Lundquist is a pioneering leader in the field of sustainable wind energy generation," says Ray Jayawardhana , Johns Hopkins provost. "Her interdisciplinary approach, combining expertise in atmospheric science and engineering with innovative computational work in modeling atmosphere-wind energy interactions, will add terrific strengths to the Sustainable Transformations and Energy BDP cluster. We are excited to welcome this accomplished scholar to Johns Hopkins University and look forward to the impactful work she will accomplish here."
The BDP clusters are faculty-developed interdisciplinary groups that are recruiting new BDPs and junior faculty members to Johns Hopkins to conduct transformational research in 10 crucial fields. The Sustainable Transformations and Energy cluster unites scientists, engineers, and market and policy experts with interests aligned toward solving critical technological and societal problems arising from the use of unsustainable chemicals and materials, fossil fuels, and other anthropogenic, environmentally harmful substances. The BDPs in this cluster will hold lead roles as part of the Ralph O'Connor Sustainable Energy Institute (ROSEI) , a nexus for sustainable energy-related research and educational programs at Johns Hopkins University.
"Hopkins is a place where big things and big dreams can happen," Lundquist says. "There are so many people with excellent and deep expertise that are all really motivated and driven. The ideas just keep blossoming, and I'm so excited about all of the potential for collaborating with amazing colleagues. A lot of the important challenges in society right now are very interdisciplinary, and I'm looking forward to working with a diverse group of collaborators to get critical work done and make an impact with our work. And becoming a part of the very first research university in the country is awe inspiring as well."
Because air flow can't be seen, it is measured with instruments such as drones, meteorological towers, and lidars, which use beams of light to sense where air is flowing and how it is flowing, important steps to figuring out why it flows the way that it does. Lundquist also uses mathematical models to simulate and predict atmosphere-wind energy interactions.
"The minute details of how wind is flowing make a big difference for wind energy generation," Lundquist explains. "There are differences, for example, between daytime and nighttime wind, between wind moving over land compared to over water, and of course, the weather is highly variable from day to day. But there are reasons for all of this, and we can write equations to then simulate what is happening with airflow."
During the day, and over land, solar radiation heats the ground, and as the ground warms, it causes bubbles that organize into convective plumes to rise up—much like boiling water on a hot stove—making the atmosphere much more turbulent. This turbulence caused by convection is what can make airplane takeoffs very bumpy. At night, the ground cools down and the turbulence dies off, leading to very stable stratified layers in the atmosphere that can lead to fast winds, called low-level jets, which provide much of the wind energy resource.
Much of Lundquist's work has focused on turbulence dissipation rate and its impact on wind energy generation. Convection cells in the atmosphere are circular patterns of rising and falling air driven by density differences in air of different temperatures: denser, colder air sinks while warmer, less dense air rises. Turbulence caused by this convection has big whirls that break down into smaller and smaller whirls, as expressed in a poem by famed scientist L. F. Richardson in 1922: "Big whirls have little whirls that feed on their velocity, and little whirls have lesser whirls and so on to viscosity." The dissipation of turbulence is the process of converting turbulent energy into heat at the smallest scales. The turbulence dissipation rate gives an indication of how quickly turbulence will erode, as well as how far away from the source turbulence will persist. This information is crucial for optimizing wind farms with multiple wind turbines as well as for predicting pollution dispersion and flow in urban areas.
"In clusters of wind turbines, the wake—the very turbulent zone downwind from a turbine—can affect downwind turbines. With strong wakes, the downwind turbines will experience slower and more turbulent flow," Lundquist explains. "This impacts the efficiency in generating electricity and leads to more technical problems and blades that need to be replaced. On the other hand, when the wake turbulence dissipates quickly, a downwind turbine won't feel the impact of its upwind neighbor.
"I'm committed to applied research—I like to address real-world problems and develop solutions that are going to be useful and help people," Lundquist adds. "What I want to understand is how can we design and operate wind farms to more effectively use the energy available to us in the atmosphere in order to provide people with affordable and clean electricity. The goal is to be more intelligent and strategic in how we deploy the resources available so that we can make maximal use of renewably generated electricity. I want to do that in a way that saves money, and without adverse environmental consequences."
Adverse consequences of large-scale deployment of wind energy, Lundquist, her students, and her collaborators have found, are minimal. Simulation studies and field experiments investigating wind turbines built in cropland in the Midwestern U.S. showed that, despite mixing a part of the atmosphere that might not usually be mixed, the atmospheric impact of wind turbines was too small to cause any adverse impacts on the surrounding crops. Temperature changes on the ground occurred only in the immediate vicinity of the wind farms, lasted for just a few hours at night, and were limited to around half of a degree Celsius.
Lundquist says because of the necessary interdisciplinary collaborations required for wind energy generation, one of her favorite parts of her job is her role in bridging the gap between different disciplines.
"I frequently need to translate between different disciplines, like between atmospheric science and engineering," Lundquist says. "For instance, there are differences in how turbulence is studied and represented in the two fields, and it's important that we're communicating clearly. There are a lot of critical issues that we can solve at the interface between atmospheric sciences and engineering. Another important type of interdisciplinarity is to go from science and engineering to the social sciences, to work with law professors to investigate if the law accurately reflects the physics of what happens in the atmosphere, or with economists to document the economic benefits of various choices when constructing wind farms. I enjoy working with colleagues whose areas of expertise are different from mine, because you learn a lot when you talk to people who aren't like you."
Moving forward, Lundquist is excited to explore the potential of offshore wind energy. As there are higher wind speeds offshore than on land, wind farms in large bodies of water have a high capacity for generating electricity. Existing research on offshore wind energy already being done at Johns Hopkins, Lundquist says, coupled with Maryland's investments in offshore wind energy, make Hopkins a very appealing place for her work in this area.
"I'm really excited about offshore wind energy, and that's one of the reasons why I wanted to come to JHU," Lundquist says. "I've noticed my doodles have started having more waves in them recently, thinking about different offshore problems. Offshore wind in the U.S. will be important for our energy transition but has many challenges that I want to help tackle. The interaction between atmosphere and ocean is complex here, with extreme weather, ocean waves, and circulations, and these challenges must be addressed now, as the offshore wind industry is taking off. This is the right time to be working on offshore wind if you, like me, are fascinated by geophysical fluid dynamics problems and want to do work that is going to make an important difference, because we need to reduce our dependence on fossil fuels."
Lundquist comes to Johns Hopkins from the University of Colorado Boulder, where she is a professor in the Department of Atmospheric and Oceanic Sciences and affiliate faculty in the Department of Applied Mathematics. She also holds a joint appointment at the National Renewable Energy Laboratory, which will continue at Hopkins.
Lundquist earned her Bachelor of Arts with a double major in English and physics at Trinity University, and her Master of Science as well as her PhD in astrophysical, planetary, and atmospheric science from the University of Colorado Boulder, along with an Environmental Policy Certificate, having completed graduate courses in environmental law, political science, journalism, and environmental science. She completed postdoctoral research at the Lawrence Livermore National Laboratory before joining the staff there as a physicist.
"Julie Lundquist is tackling some of renewable energy's most complex challenges with vision, originality, and a talent for building productive partnerships and collaborations that draw upon an incredibly wide range of expertise," says Ed Schlesinger , dean of the Whiting School of Engineering. "Her eagerness to pursue bold ideas and her ability to create wholly novel solutions that work at a human level—with a focus on accessibility and improving people's lives—make her a great addition to the Whiting School and the university."
Adds Christopher Celenza , dean of the Krieger School of Arts and Sciences: "Julie Lundquist brings a unique set of skills to our esteemed Department of Earth and Planetary Sciences. Her interdisciplinary experience shows that she is eager to take on big challenges in the field of sustainable energy. I am confident that she will be an asset to her colleagues in the Sustainable Transformations and Energy research cluster and an inspiration to our students."
In addition to her research, Lundquist looks forward to continuing to work with students—mentorship she enjoys and considers her greatest professional accomplishment.
"One of the best pieces of advice I've ever gotten was to always hire people who are smarter than I am—and every time I've had the opportunity to do that, I have," Lundquist says. "My students continually surprise and impress me with their creativity, their approaches, and their determination. In our research, you not only have to spark new ideas, but you have to persist to implement them, pushing through the inevitable disappointments. Working on projects with students, and continuing to collaborate with former students, allows me to enjoy and appreciate their great achievements. That is incredibly rewarding."
As a Bloomberg Distinguished Professor, Lundquist joins an interdisciplinary cohort of scholars working to address major world problems and teach the next generation. The program is backed by support from Bloomberg Philanthropies .
Posted in University News
Tagged sustainability , krieger school , bloomberg distinguished professorships , ralph s. o'connor sustainable energy institute , whiting school
You might also like
News network.
- Johns Hopkins Magazine
- Get Email Updates
- Submit an Announcement
- Submit an Event
- Privacy Statement
- Accessibility
Discover JHU
- About the University
- Schools & Divisions
- Academic Programs
- Plan a Visit
- my.JohnsHopkins.edu
- © 2024 Johns Hopkins University . All rights reserved.
- University Communications
- 3910 Keswick Rd., Suite N2600, Baltimore, MD
- X Facebook LinkedIn YouTube Instagram

IMAGES
VIDEO
COMMENTS
Learn how convection is the transfer of heat by the movement of heated particles into an area of cooler particles. See how to create your own convection current with water and food coloring in a glass.
Learn how convection currents work with a simple experiment using hot and cold water and food colouring. Find out how convection is different from conduction and radiation, and see examples of convection in nature.
Convection is the transfer of heat by the movement of currents within a fluid. In our experiment, the oil at the bottom of the bowl was heated by the candle. ... Convection currents are all around us and responsible for heating many things! Our homes are heated in the winter through convection currents. The troposphere of the atmosphere (the ...
What Are Convection Currents? Convection is the movement within a liquid (or air) when the hotter and less dense liquid rises, and the colder, denser liquid sinks due to gravity. These movements create the circulation patterns in our atmosphere through air and water. The warm water or air rises and allows cooler air (or water) to go underneath.
Invisible convection currents are revealed using a very colorful demonstration. Cool, crisp, clean mountain air has long been an important reason why people move to Colorado, USA. Unfortunately, the air in the city of Denver isn't quite as clean as it has been. By the 1970s, pollution over the city had a name—the "brown cloud.".
Convection Currents w a r m r i s e s c o o l s i n k s Reference: Berkeley UC Museum of Paleontology Source: Rookie Parenting Science Experiment Set-Up Convection currents are heat-driven cycles that occur in the air, ocean, and mantle. They are caused by a difference in temperature, often due to a differing proximity to a heat source. The
Convection Currents with Ice Cubes Experiment (Convection currents demonstration and experiments)Made for parents and teachers Science Kits and morehttps://e...
Ready for another? The wetland rap is gonna blow you away!https://www.youtube.com/watch?v=X33FX8pG-Dc-~-~~-~~~-~~-~-This fun science experiment illustrates c...
Here I model a convection current lab. This goes with several units including heat transfer, weather and climate, and the Uneven Heating of the Earth's Surfa...
This experiment was safety-checked in March 2020. Download colourful convection (ppt, 119 KB) Appears in these Collections. Show me. Filter. Apply filters. ... The purpose of this demonstration is to show the build-up of convection currents of warm air in a room. Classroom Activity 11-14 Convective Heating. Energy and Thermal Physics.
Fill the beaker with water. Put individual crystals of potassium manganate VII on the bottom of the beaker, using the method suggested above. Heat the water gently over the Bunsen burner and observe the motion of the coloured water. For a clear effect, use a small flame and no gauze between Bunsen and beaker (Pyrex beakers do stand this).
The point source of a Mini Maglite projects clear images of convection when used on a small-scale desktop experiment like this. Changing the distance from the point light source to the candle will change the magnification of the image of the convection currents projected. Related Snacks. Pie-Pan Convection. Visualize the spectacular fluid ...
Convection, another method of heat transfer, is the movement of water in the plate during Part 2. (For additional information on radiation and convection, refer to the background section of this activity.) Show students what their experiment set up will look like for Part 3(a shoebox of water, blue ice cubes, and red food coloring).
Convection currents are flowing fluid that is moving because there is a temperature or density difference within the material. Because particles within a solid are fixed in place, convection currents are seen only in gases and liquids. A temperature difference leads to an energy transfer from an area of higher energy to one of lower energy.
To perform the convection snake experiment, you will need a piece of paper and scissors. Using the scissors, cut the piece of paper into a spiral shape that is 6 cm long. Attach one end of a 15-cm piece of yarn to the middle of the spiral using clear tape. Take a table lamp and hang the paper spiral about 10 cm above the table lamp.
This experiment is a very graphic demonstration of convection currents. You need a big cardboard box, transparency, candle and a few incense sticks. Make two...
What do convection currents have to do with the weather? The red water represents a warm air mass, and the blue water represents a cold air mass. A thunderstorm is caused by unstable air. A body of warm air is forced to rise by an approaching cold front forming a strong, persistent updraft of warm moist air.
Steps for Convection Currents in Water Experiment : 1. First of all fill the flask with water. 2. Take out circular bits of colored paper with the help of paper punch. 3. Drop some paper bits in flask and immerse them properly in water. 4. Put the flask over burner and heat for 5 minutes.
Do you need some ideas for simple convection currents experiments? Let's talk about a lab that demonstrates how convection currents work! In this post. ... Convection currents come up a lot in Science class, and it can be supported by these two NGSS standards: MS-ESS2-1. Develop a model to describe the cycling of Earth's materials and the ...
Convection in a liquid. Convection in a liquid can be seen by putting a crystal of potassium permanganate in a beaker of water and gently heating it with a Bunsen flame. A beaker is heated and the ...
Convection currents are present in the air - A good example of convection current is the warm air that rises towards the ceiling in your house. The process happens as the warm air is said to be less dense than that of the colder air. Another good example of convection current is wind. The wind is mainly caused when the reflected radiation of ...
Convection currents are the result of uneven heating of water or air. These currents create some of our wind, weather, and ocean currents.In order to demonst...
In order to find out how metal foam and rotation angle affected the natural convection of nanofluids in a hollow exposed to a magnetic field, Qi et al. 21 conducted an experiment. Their research ...
Much of Lundquist's work has focused on turbulence dissipation rate and its impact on wind energy generation. Convection cells in the atmosphere are circular patterns of rising and falling air driven by density differences in air of different temperatures: denser, colder air sinks while warmer, less dense air rises.
This video demonstrates a convection experiment for GCSE Physics using potassium permanganate in water with a Bunsen burner.Convection can be demonstrated wh...