- View PDF
- Download full issue


Revista Clínica Española (English Edition)
Review doping and sports endocrinology: anabolic-androgenic steroids endocrinología del dopaje y los deportes: andrógenos anabolizantes.
- Previous article in issue
- Next article in issue
Palabras clave
Cited by (0).
Anabolic Steroid use in Sports and in Physical Activity: Overview and Analysis
- October 2018
- Sport Mont Journal 16(3):113-118

- Parthenope University of Naples
Abstract and Figures

Discover the world's research
- 25+ million members
- 160+ million publication pages
- 2.3+ billion citations

- Mustafa M Basree
- Aasems Jacob

- Leandro Almeida de Oliveira
- Nicholas Mayers
- Marcos Gonçalves Santana

- INT J MOL SCI

- Zhang Wenbo
- Rodrigo Cristiano Stechechen

- Ayomide Aribisala Goodness

- Jéssica Miranda da Silva
- Jéssica Rodrigues da Silva
- Adriano Rios da Silva Santana Leite

- A. Esposito
- MOL CELL ENDOCRINOL
- Bradley D Anawalt

- Shehzad Basaria
- Anna Goldman
- PULM PHARMACOL THER

- Ryan Modlinski
- Karl B Fields
- Gen Kanayama
- Harrison G. Pope
- Recruit researchers
- Join for free
- Login Email Tip: Most researchers use their institutional email address as their ResearchGate login Password Forgot password? Keep me logged in Log in or Continue with Google Welcome back! Please log in. Email · Hint Tip: Most researchers use their institutional email address as their ResearchGate login Password Forgot password? Keep me logged in Log in or Continue with Google No account? Sign up
- Search Menu
- Sign in through your institution
- Advance articles
- Author Guidelines
- Open Access
- About Integrative and Comparative Biology
- About the Society for Integrative and Comparative Biology
- Editorial Board
- Advertising and Corporate Services
- Journals Career Network
- Self-Archiving Policy
- Dispatch Dates
- Journals on Oxford Academic
- Books on Oxford Academic
Article Contents
Introduction, implications of studies of humans for studies of nonhuman animals, conclusions, acknowledgments.
- < Previous
Steroid use and human performance: Lessons for integrative biologists
From the symposium “Hormonal Regulation of Whole-Animal Performance: Implications for Selection” presented at the annual meeting of the Society for Integrative and Comparative Biology, January 3–7, 2009, at Boston, Massachusetts.
2 Present address: Department of Biology, University of South Dakota, Vermillion, SD 57069, USA
- Article contents
- Figures & tables
- Supplementary Data
Jerry F. Husak, Duncan J. Irschick, Steroid use and human performance: Lessons for integrative biologists, Integrative and Comparative Biology , Volume 49, Issue 4, October 2009, Pages 354–364, https://doi.org/10.1093/icb/icp015
- Permissions Icon Permissions
While recent studies have begun to address how hormones mediate whole-animal performance traits, the field conspicuously lags behind research conducted on humans. Recent studies of human steroid use have revealed that steroid use increases muscle cross-sectional area and mass, largely due to increases in protein synthesis, and muscle fiber hypertrophy attributable to an increased number of satellite cells and myonuclei per unit area. These biochemical and cellular effects on skeletal muscle morphology translate into increased power and work during weight-lifting and enhanced performance in burst, sprinting activities. However, there are no unequivocal data that human steroid use enhances endurance performance or muscle fatigability or recovery. The effects of steroids on human morphology and performance are in general consistent with results found for nonhuman animals, though there are notable discrepancies. However, some of the discrepancies may be due to a paucity of comparative data on how testosterone affects muscle physiology and subsequent performance across different regions of the body and across vertebrate taxa. Therefore, we advocate more research on the basic relationships among hormones, morphology, and performance. Based on results from human studies, we recommend that integrative biologists interested in studying hormone regulation of performance should take into account training, timing of administration, and dosage administered when designing experiments or field studies. We also argue that more information is needed on the long-term effects of hormone manipulation on performance and fitness.
One of the most widely discussed and controversial arenas of human performance concerns the use of steroid supplements to enhance athletic ability for a variety of sports, ranging from bicycling to baseball. There is strong evidence that human athletes have attempted to enhance their athletic performance using steroids since the 1950s, but whether, and in which sports, steroids are actually effective remains controversial (reviewed by Ryan 1981 ; George 2003 ; Hartgens and Kuipers 2004 ). In general, steroids used by athletes encompass a wide variety of forms of the androgen testosterone (George 2003 ), and most seem to have the classical androgenic and anabolic effects on men, although steroid use by women cannot be ignored (Malarkey et al. 1991 ; Gruber and Pope 2000 ). Alternative forms of testosterone (e.g., testosterone enanthate, methandrostenolone) are typically used by those desiring enhanced performance because ingested or injected testosterone is quickly metabolized into inactive forms (Wilson 1988 ). Thus, studies of humans that we cite involve testosterone derivatives. Early studies of the effects of steroids on human performance, however, had major flaws in design, such as lack of control groups and a double-blind procedure, the presence of confounding factors (e.g., differences in level of exercise and in motivation), and inappropriate statistical techniques (reviewed by Bhasin et al. 2001 ; George 2003 ). These problems left open for many years the question of whether, and in what capacity, steroids actually enhance athletic performance, until more recent studies conclusively showed significant effects of steroids.
The topic of steroid effects on human athletic performance is germane to an emerging field of research investigating hormonal effects on animals’ performance (e.g., sprint speed, endurance capacity, bite-force capacity) (Husak et al. 2009a ), as testosterone may exert general effects on performance across widely divergent vertebrate taxa. Our goal in this review is to interpret the effects of steroids on human performance in this broader context of hormonal effects across a wider range of taxa. We are particularly interested in drawing lessons and potential avenues of research for animal biologists from published research on humans. We have performed a selective review of studies examining how humans' use of steroids affects skeletal muscle physiology and subsequent athletic performance. While studies of performance on nonhumans have dealt extensively with the effects of morphological traits on performance and the impact of performance on individual fitness (Arnold 1983 ; Garland and Losos 1994 ; Irschick and Garland 2001 ; Irschick et al. 2007 , 2008 ; Husak et al. 2009a ), there has been relatively little synthetic discussion of how hormones affect performance in non-human animals. We also point the reader towards several recent reviews of steroid use and performance by humans for details not discussed in our review (Bhasin et al. 2001 ; George 2003 ; Hartgens and Kuipers 2004 ).
General effects of testosterone on the phenotype of males
The development of primary and secondary sexual characteristics is stimulated by testosterone in vertebrate males, and these effects can be either organizational or activational in nature (Norris 1997 ; Hadley 2000 ). Organizational effects tend to occur early in development, and during a critical window of time, thereby resulting in permanent effects. On the other hand, activational effects occur in adults, and the effects are typically temporary (Arnold and Breedlove 1985 ). The hypothalamus stimulates production of gonadotropin-releasing hormone, which in turn stimulates production of luetenizing hormone in the anterior pituitary. Luetenizing hormone then stimulates production of testosterone in the Leydig cells of the testes. Testosterone then circulates throughout the body where it exerts effects on multiple target tissues that have the appropriate receptors or appropriate enzymes (e.g., aromatase or 5α-reductase) to convert testosterone for binding to other types of receptors (Kicman 2008 ). The widespread effects of circulating levels of testosterone on aggression, secondary sexual traits, and growth of skeletal muscle in males of many vertebrate species are well-documented (Marler and Moore 1988 ; Wingfield et al. 1990 ; Ketterson and Nolan 1999 ; Sinervo et al. 2000 ; Ketterson et al. 2001 ; Oliveira 2004 ; Adkins-Regan 2005 ; Hau 2007 ; contributions in this issue). In particular, production of testosterone by males has been linked with the expression of color and behavioral display signals, as well as aggression (Marler and Moore 1988 ; Kimball and Ligon 1999 ; Hews and Quinn 2003 ; Adkins-Regan 2005 ; Cox et al. 2008 ) and increased growth (Fennell and Scanes 1992 ; Borski et al. 1996 ; Cox and John-Alder 2005 ), although this latter effect may depend on specific selective pressures on males (Cox and John-Alder 2005 ).
Effects of testosterone on the physiology of human skeletal muscle
Testosterone has multiple effects on skeletal muscle at the biochemical and cellular levels, but the direct cause-and-effect relationships among these effects are still unclear (Sinha-Hikim 2002 ; Hartgens and Kuipers 2004 ). The studies that we discuss here, and throughout the paper are from experiments or correlative studies conducted on adult individuals such that the effects seen are activational in nature, causing rather rapid changes to the phenotype. Increased testosterone causes increased protein synthesis by muscle cells (Griggs et al. 1989 ; Kadi et al. 1999 ; Hartgens and Kuipers 2004 ), which is necessary for anabolic effects and an increase in lean muscle mass. Sinha-Hikim et al. ( 2002 ) found a dose-dependent increase in the mean number of myonuclei found in skeletal muscle fibers ( vastus lateralis muscle) with testosterone supplementation, as well as in the number of myonuclei per fiber (see also Eriksson et al. 2005 ). This increase was also associated with an increase in the number of satellite cells in the muscle tissue (but see Eriksson et al. 2005 ). Satellite cells are progenitor cells found external to muscle fibers that are incorporated into fibers and promote repair and growth of the muscle (Kadi and Thornell 2000 ; Reimann et al. 2000 ). However, the mechanism by which testosterone causes an increase in the number of satellite cells is unknown and could be due to testosterone (1) promoting cell division of satellite cells, (2) inhibiting apoptosis of satellite cells, or (3) causing differentiation of stem cells into satellite cells (Sinha-Hikim 2002 ). In any case, the functional implications for these findings are clear. More satellite cells likely result in more myonuclei per fiber, which, combined with increased protein synthesis, contribute to increases in muscle growth via an increased number and hypertrophy of muscle fibers (Kadi 2000 ; Kadi and Thornell 2000 ).
Testosterone also appears to cause a dose-dependent increase in the cross-sectional area of muscle fibers, although details about which types of fibers are affected and where in the body this occurs remains equivocal. Testosterone may increase the cross-sectional area of both type I (oxidative “slow twitch”) and type II (glycolytic “fast twitch”) fibers simultaneously after administration (Sinha-Hikim 2002 ; Eriksson et al. 2005 ), but other studies have shown greater increases in type I than in type II fibers (Hartgens et al. 1996 ; Kadi et al. 1999 ; also in growing rats, Ustunelet al. 2003 ), increased size in only type I fibers (Alén et al. 1984 ; Kuipers et al. 1991 , 1993 ), or increased size in only type II fibers (Hartgens et al. 2002 ). These mixed results are intriguing, because they suggest that different parts of the body, and, hence, different performance traits, may be affected differently by elevated testosterone levels. The likely mechanism for these differences is variation in density of receptors within the myonuclei of muscle fibers in different regions of the body (Kadi 2000 ; Kadi et al. 2000 ). An alternative hypothesis is that different types of fiber have differing relationships between the number of internal myonuclei and muscle cross-sectional area during hypertrophy (Bruusgaard et al. 2003 ). That is, some types of fibers may have internal myonuclei that can serve larger “nuclear domains” than can other types of fibers (reviewed by Gundersen and Bruusgaard 2008 ). If either of these hypothesized mechanisms is correct, then circulating levels of testosterone may only explain a portion of inter-individual (or interspecific) variation in performance. Testosterone may also stimulate changes in the proportions of types of fibers in muscles (Holmang et al. 1990 ; Pette and Staron 1997 ), although evidence for this effect in humans is mixed. For example, Sinha-Hikim et al. ( 2002 ) did not observe a change in the proportions of type I and type II fibers after administration of testosterone.
Changes in lower-level traits (e.g., protein synthesis, number of satellite cells, cross sectional area of muscle fibers) after testosterone supplementation, as described above, thus, result in changes at the whole-muscle level and explain many of the classic effects of testosterone that are desired by humans using steroids. That is, increasing testosterone via steroid use increases body weight, lean body mass, as well as cross-sectional area, circumference, and mass of individual muscles (i.e., “body dimensions”); however, there are numerous studies with contradictory results, finding no change in one, or all, of these traits, depending on the drug used, the dose taken, and the duration of use (reviewed by Bhasin et al. 2001 ; Hartgens and Kuipers 2004 ). The finding that testosterone can change muscle physiology and increase whole-muscle size and/or body mass is consistent with results in nonhuman animals. For example, testosterone implants increased size and number of fibers in the sonic muscles of male plainfin midshipman fish ( Porichthys notatus ) (Brantley et al. 1993 ). Similarly, testosterone supplementation increased muscle mass and changed contractile properties of trunk muscles of male grey treefrogs ( Hyla chrysoscelis ) (Girgenrath and Marsh 2003) and of forelimb muscles of male frogs ( Xenopus laevis , Regnier and Herrera 1993 ; Rana pipiens , Sidor and Blackburn 1998 ).
Effects of testosterone on humans’ performance
Whether steroids actually enhance performance of athletes was a subject of great controversy throughout the 1980s and 1990s (Ryan 1981 ; Haupt and Rovere 1984 ; Cowart 1987 ; Wilson 1988 ; Elashoff et al. 1991 ; Strauss and Yesalis 1991 ; Hartgens and Kuipers 2004 ), largely due to flaws in design of early studies (see above). However, the past decade has seen a surge in more carefully designed studies that have convincingly tested whether, all else equal, steroids increase performance. Hartgens and Kuipers ( 2004 ) found that 21 out of 29 studies they reviewed found an increase in humans’ strength after steroid use, with improvements in strength ranging from 5% to 20%. Storer et al. ( 2003 ) found that testosterone caused a dose-dependent increase in maximal voluntary strength of the leg (i.e., amount of weight lifted in a leg press), as well as in leg power (i.e., the rate of force generation). They further tested whether increased muscle strength was due simply to increased muscle mass or to changes in the contractile quality of muscle affected by testosterone, but they found no change in specific tension, or in the amount of force generated per unit volume of muscle. This latter result suggests that, at least for leg-press performance, testosterone increases strength by increasing muscle mass and not by changing contractile properties. Rogerson et al. ( 2007 ) found that supraphysiological doses of testosterone increased maximal voluntary strength during bench presses (see also Giorgi et al. 1999 ) and increased output of work and output of power during cycle sprinting compared to placebo control subjects. Thus, “burst” or “sprint” performance traits appear to be enhanced by increased testosterone, and this is in general agreement with studies of nonhuman animals (John-Alder et al. 1996 , 1997 ; Klukowski et al. 1998 ; Husak et al. 2007 ). For example, experimentally elevated levels of testosterone caused increased sprint speed, relative to sham-implanted individuals, in northern fence lizards ( Sceloporus undulatus ) (Klukowski et al. 1998 ). These findings contrast with results for endurance events, in which no increase in performance has been detected experimentally in humans (reviewed in George 2003 ; Hartgens and Kuipers 2004 ). The finding that endurance by humans is not enhanced by testosterone is unexpected since testosterone may increase hemoglobin concentrations and hematocrit (Alén 1985 , but see Hartgens and Kuipers 2004 ) and exogenous testosterone increases endurance in rats (Tamaki et al. 2001 ) and male side-blotched lizards ( Uta stansburiana ) (Sinervo et al. 2000 ). More studies of the effects of increased testosterone on endurance would help to better clarify these seemingly paradoxical findings. One possibility that might explain species’ differences in endurance is the relative proportion of type I fibers available for enhancement, which likely varies across species (Bonine et al. 2005 ), although this hypothesis needs explicit testing. Steroid use does not seem to consistently enhance recovery time after strenuous exercise (reviewed in Hartgens and Kuipers 2004 ), although it may in non-human animals (Tamaki et al. 2001 ). Storer et al. ( 2003 ) also found no change in fatigability (i.e., the ability of a muscle to persist in performing a task) of muscle during exercise, which is consistent with other studies (George 2003 ).
One of the problems in early studies of steroid effects was that the participants’ history of training and exercise while taking steroids was not taken into account or controlled (Bhasin et al. 2001 ; George 2003 ; Hartgens and Kuipers 2004 ). Recent studies have shown that the presence or absence of exercise training during testosterone supplementation can have a marked impact on how much performance is enhanced, thus complicating results when training is not controlled. Bhasin et al. ( 2001 ) reviewed several examples of such results. They pointed out that testosterone supplementation alone may increase strength from baseline levels, but so will exercise alone with a placebo, such that strength levels with exercise alone are comparable to those with testosterone addition alone (Bhasin et al. 1996 ). Testosterone supplementation while undergoing exercise training typically has the greatest increase in strength compared to exercise only or testosterone only (Bhasin et al. 1996 , 2001 ). These findings are consistent with those of others (reviewed by George 2003 ). Indeed, George ( 2003 ) suggested that steroids will only consistently enhance strength if three conditions are met: (1) steroids are given to individuals who have been training and who continue to train while taking steroids, (2) the experimental subjects have a high protein diet throughout the experiment, and (3) changes in performance are measured by the technique with which the individuals were training while taking steroids. That is, one may, or may not, find a change in bench-press performance if individuals trained with leg presses, and not bench presses, while taking steroids. We note that the confounding effect of training is a rather intuitive finding, but it does point out potential problems in studies of non-human animals, specifically laboratory studies, which we address below.
Given the effects of steroids on physiology and performance of human muscle, what can integrative biologists take away from these findings? We suggest that they can provide some valuable insights into the mechanisms of how hormones might regulate whole-animal performance traits in nonhuman animals. The most obvious lesson is that manipulating the circulating levels of testosterone, or its derivatives, increases overall strength, which has apparent benefits for performance in bursts, such as sprint speed. In contrast, there is little evidence from studies on humans for a positive effect on capacity for endurance, which is counter-intuitive, given the known effect of testosterone on hemoglobin concentrations and hematocrit. However, these same studies of humans also raise a host of issues that merit special consideration by researchers interested in hormonal effects on nonhuman animals, including effect of training, timing of administration, and dosage administered. We also argue that more information is needed on the long-term effects of hormonal manipulation on performance and fitness. Although recent studies suggest that increasing testosterone levels can enhance certain types of performance, we are not advocating or justifying the use of steroids by humans. There are numerous side effects of prolonged steroid use in humans, including cardiovascular problems, impaired reproductive function, altered behavior, increased risk of certain tumors and cancers, and decreased immune function, among others (reviewed by Pärssinen and Seppälä 2002 ; George 2003 ). These “side-effects” are in accordance with studies of nonhuman animals where higher testosterone levels are associated with such detrimental effects as increased loads of parasites, reduced immunocompetence, decreased body condition, reduced growth, and increased use of energy, ultimately resulting in reduced survival (Marler and Moore 1988 ; Folstad and Karter 1992 ; Salvador et al. 1996 ; Wikelski et al. 1999 , 2004 ; Moore et al. 2000 ; Peters 2000 ; Klukowski and Nelson 2001 ; Wingfield et al. 2001 ; Hau et al. 2004 ). Indeed, it is the presence of these very “side-effects” that has driven a great deal of research on behavioral and life-history tradeoffs mediated by testosterone (Ketterson and Nolan 1999 ; Ketterson et al. 2001 ). Higher levels of testosterone may enhance performance and increase success at some tasks, but its widespread “pleiotropic” effects on other aspects of the phenotype may result in a net detriment to fitness (Raouf et al. 1997 ; Reed et al. 2006 ; Ketterson et al. 2009).
We encourage researchers to complete more detailed studies of the interactions among hormones, morphology, and performance, especially across different types of performance traits (dynamic versus regulatory, see Husak et al. 2009a ). Comparative data on whether the same, or different, hormones affect the same performance traits in different taxa (e.g., burst speed in fish, sprint speed in lizards) would be useful for understanding how different species have evolved unique, or conserved, endocrine control of morphology and function. A comparative approach is important, as other studies have shown different effects of testosterone on performance in different taxa (e.g., an increase in endurance for rats and lizards, but none for humans), and more research is needed to determine whether such differences are valid or purely methodological. Even though testosterone is confined to vertebrates, it is possible that studies with invertebrates may reveal similar effects on performance via different hormones, e.g., recent work showing a seemingly similar role of juvenile hormone for invertebrates as testosterone has for vertebrates (Contreras-Garduno et al. 2009 ; see also Zera 2006 ; Zera et al. 2007 ; Lorenz and Gäde 2009).
Correlative studies relating endogenous circulating hormone levels to natural variation in performance traits can provide valuable insight into potential mechanistic regulators of performance, but manipulations allow a more detailed examination of cause-and-effect relationships. Whether performance can be manipulated by reduction (castration) or supplementation (implants) of testosterone in nonhuman animals will depend on the type of performance and how it is affected by circulating levels of the androgen. Many dynamic performance traits, especially maximal performance, may show different responses to exogenous hormone in the laboratory versus field, compared to coloration or “behavioral” traits. For example, supplementation with testosterone may rapidly increase display behavior or aggression in the laboratory (Lovern et al. 2001 ; Hews and Quinn 2003 ) compared to control animals, or corticosterone supplementation may decrease sexually selected color patterns (reviewed by Husak and Moore 2008 ). These examples are in contrast to supplementing testosterone in the laboratory and testing for an effect on performance. Aggression and coloration will not likely require training of the target trait to reveal an observed effect, whereas some performance traits may require training. Furthermore, regulatory performance traits (e.g., regulation of ions in seawater), on the other hand, may respond more directly to hormonal manipulation (see McCormick 2009), and will likely not require any training, but more empirical data are necessary to make generalizations.
It is also important to more closely inspect those traits that show no significant effect of testosterone on dynamic performance after manipulation in the laboratory. Such a “noneffect” may be due to numerous possibilities, the most obvious of which is that testosterone simply has no effect on a particular type of performance. However, a second possibility is that muscles involved in performance were not adequately trained during administration of supplemental testosterone, or there was no control of exercise during the period of testosterone administration. As an hypothetical example, one might not expect to see a large increase in the maximal flight speed of birds that were never allowed to fly following administration of exogenous testosterone. Indeed, Gallotia galloti lizards given exogenous testosterone were compared to lizards given sham implants and there was no difference in maximal bite force at the end of the experiment (K. Huyghe, J.F. Husak, R. Van Damme, M. Molina-Borja, A. Herrel, in review), despite increases in mass of the jaw muscles in testosterone-supplemented males. One possible explanation for this result is that these lizards did not “train” their jaw muscles enough while in captivity to increase muscle mass sufficiently to result in a measurable enhancement of performance. It is also possible that receptor density is very low or becomes low in trained muscles. Nevertheless, while training in animals seems straightforward in principle, in practice it is far trickier, and there also appear to be striking differences among species in the effects of training. Whereas some studies of mammals have successfully increased performance through training in a laboratory (Brooks and Fahey 1984 ; Astrand and Rodahl 1986 ), similar studies with lizards have found no effect (Gleeson 1979 ; Garland et al. 1987 ). In addition, while training might be successful with animals acclimated to a laboratory setting, inducement of stress, with a concomitant effect on corticosterone (Moore and Jessop 2003 ), and potentially circulating testosterone levels, is a significant confounding factor. Another complementary option is to use field studies, where experimental groups are released into the wild to “train” themselves while accomplishing their day-to-day tasks and performing naturally. Of course, this approach also cannot take into account variation in “training” within experimental groups, as individuals will likely use their performance traits in different ways when left to their own devices. Consequently, this approach could result in unpredictable results in how hormones impact performance, unless one accepts the unlikely assumption that all experimental animals are performing in the same ways. Further, a field approach also does not take into account other “pleiotropic” effects of increased (or decreased) testosterone on the phenotype (e.g., increased activity or conspicuousness to predators), which can eliminate potential benefits to fitness from enhanced performance due to testosterone supplementation.
Studies seeking to manipulate performance with testosterone supplementation should also consider the timing of experiments. For example, testosterone should ideally be increased or decreased during times when the hypothalamic–pituitary–gonad (HPG) axis is responsive and receptors are expressed in the appropriate target tissues. Seasonal sensitivity of the male HPG axis is well documented (Fusani et al. 2000 ; Jawor et al. 2006 ; Ball and Ketterson 2008 ), and such effects should be considered. For example, male green anoles ( Anolis carolinensis ) given exogenous testosterone after the end of the breeding season in a laboratory setting did not increase head size or bite-force performance (J. Henningsen, J. Husak, D. Irschick, and I. Moore, unpublished data), presumably because some or all of the relevant target tissues were no longer sensitive to androgens. On the other hand, male brown anoles ( Anolis sagrei ) did show enhanced maximal bite force when testosterone was supplemented at the beginning of the breeding season when the target tissues are presumably sensitive to androgens (Cox et al., in press). Timing of experimentation is thus critical for designing studies examining hormonal effects, and the interaction between timing and training should also be considered, as training effects may be relevant for some seasonal periods, but not for others.
A related issue concerns how much hormone to administer to experimental subjects. Studies of human steroid use typically involve supraphysiological doses of testosterone, as this is the typical regimen for steroid-abusing athletes (George 2003 ; Hartgens and Kuipers 2004 ). Indeed, many studies of steroid use by humans have been criticized for having experimental groups using physiological doses of testosterone. However, such criticism of seemingly unrealistic dosages highlights the differing goals of studies on human and non-human animals. Whereas studies of humans are focused on the role of supraphysiological doses on performance, those of nonhuman animals are more broadly interested in whether circulating testosterone affects performance within more natural bounds of variation (reviewed by Fusani et al. 2005 ; Fusani 2008 ). Supraphysiological doses can result in unexpected, or even counterintuitive, effects because endocrine systems tend to be homeostatic and compensatory after disruption via up- or down-regulation of various components within the system (Brown and Follett 1977 ).
There are few data on how testosterone affects dynamic performance during different stages of development, either in humans or in non-human animals. Practically all studies examining the effects of exogenous testosterone on humans have been on adults (reviewed by Hartgens and Kuipers 2004 ), but an increasing area of concern is steroid use by teenagers (Johnston et al. 2005 ). Because they are still developing physically, steroids may have dramatically different effects on dynamic performance in developing juveniles versus older adults. For example, steroid use is known to cause closure of growth plates of long bones (George 2003 ), potentially preventing growth to full height. Any manipulative hormone study examining effects on dynamic performance should also take baseline circulating levels into account, as there may be striking differences among age groups. For example, among sexually mature male green anole lizards in a well-studied New Orleans, Louisiana (USA) population, smaller “lightweight” males have lower circulating testosterone levels (Husak et al. 2007 , 2009b ), relatively smaller heads, and lower bite forces than do larger “heavyweight” males (see Lailvaux et al., 2004; Vanhooydonck et al., 2005a), with the difference apparently due to age (Irschick and Lailvaux 2006). Smaller males with low testosterone levels seem unable to produce higher levels (Husak et al. 2009b ), suggesting that testosterone levels are likely suppressed until a critical body size when the individuals become competitive with larger males. At this body size, elevated testosterone levels may accelerate growth of the head and increase bite force, although more data are needed to test this hypothesis. This ontogenetic increase in testosterone levels suggests that exogenous administration will have quite different effects on different age groups. For example, many hormones exert threshold effects (reviewed in Hews and Moore 1997 ) in which increased amounts above a threshold level produce little noticeable effect, suggesting that exogenous administration may accomplish little for larger lizards already with high testosterone levels, but may have substantial effects on smaller lizards with low testosterone levels.
In this context, long-term studies in animal species that focus on younger individuals (see Cox and John-Alder 2005 and references therein) might be useful for understanding the potential costs and benefits of hormones in improving or decreasing dynamic performance. Scientists are well-aware of some of the short-term activational effects of testosterone in humans and nonhuman animals, but while some long-term effects of supraphysiological doses on human health are recognized (see Hartgens and Kuipers 2004 ), we know far less about long-term effects of elevated (but not supraphysiological) testosterone levels on longevity and lifetime reproductive success of nonhuman animals. Ethical considerations may preclude long-term hormone implantation in humans and nonhuman animals, but correlating natural variation in testosterone levels both with performance traits and with other demographic features, such as longevity and lifetime reproductive success, would be useful for understanding chronic effects. Elegant studies with the dark-eyed junco ( Junco hyemalis ) (Ketterson et al. 2001 ; Reed et al. 2006 ) show complex trade-offs between different components of reproductive success (e.g., investment in extra-pair fertilizations versus parental care) as a result of testosterone supplementation; other similar trade-offs might be occurring over longer time spans in other animal species.
Despite popular interest in steroids and their effects on human athletic performance, we still lack a broad understanding of the effects of testosterone on performance in different animal species.
Our review of the literature on human steroids highlights several issues that could prove useful for integrative biologists interested in determining links among hormones, morphology, performance, and fitness in nonhuman animal species. First, studies of steroid use by humans reveal many caveats related to experimental design and interpretation that should be considered by those studying nonhuman animals (e.g., training, diet, dosage effects). Second, because of conflicting results of testosterone on different performance traits (e.g., burst performance versus endurance), more data are needed for such biomechanically opposing performance traits; testosterone may enhance multiple kinds of performance in some species, and only one kind in another. Third, while testosterone may have some general effects on dynamic performance in vertebrates, are there other hormones (e.g., juvenile hormone) that play a similar role in invertebrates? Finally, human steroid abusers often use various systems of “stacking”, where multiple drugs are taken in a specific order (George 2003 ), and such regimens are believed, by those who use them, to markedly increase dynamic performance. However, few studies have specifically examined how these regimes affect performance, or how the different regimes may be more, or less, effective in enhancing performance, either in humans or in non-human animal species. Furthermore, such practices are not restricted to multiple androgens, but may also include other hormones, such as growth hormone and insulin-like growth factor-I, which may, when taken exogenously, also enhance athletic performance and other aspects of the phenotype (Gibney et al. 2007 ). In this manner, the interactive effects of different hormone regimens for increasing animal performance are highly understudied. In conclusion, we have advocated an integrative approach for studying the evolution of morphology, function, and endocrine systems, and increased collaboration between researchers interested in human and in other animal systems may prove fruitful for both groups.
Financial support was provided by the National Science Foundation (IOS 0421917 to DJI and IOS 0852821 to I. T. Moore, JFH and DJI).
We are thankful to the symposium participants for fruitful discussions about hormones and performance. We thank the Society for Integrative and Comparative Biology, especially the Divisions of Animal Behavior, Comparative Endocrinology, and Vertebrate Morphology, for providing logistical and financial support.
Google Scholar
Google Preview
Author notes
Month: | Total Views: |
---|---|
December 2016 | 1 |
January 2017 | 11 |
February 2017 | 28 |
March 2017 | 44 |
April 2017 | 80 |
May 2017 | 59 |
June 2017 | 41 |
July 2017 | 45 |
August 2017 | 169 |
September 2017 | 123 |
October 2017 | 148 |
November 2017 | 35 |
December 2017 | 695 |
January 2018 | 740 |
February 2018 | 723 |
March 2018 | 1,032 |
April 2018 | 1,245 |
May 2018 | 947 |
June 2018 | 921 |
July 2018 | 614 |
August 2018 | 884 |
September 2018 | 860 |
October 2018 | 993 |
November 2018 | 1,374 |
December 2018 | 1,191 |
January 2019 | 798 |
February 2019 | 992 |
March 2019 | 1,202 |
April 2019 | 1,128 |
May 2019 | 1,186 |
June 2019 | 1,187 |
July 2019 | 1,102 |
August 2019 | 1,081 |
September 2019 | 1,024 |
October 2019 | 753 |
November 2019 | 593 |
December 2019 | 575 |
January 2020 | 653 |
February 2020 | 627 |
March 2020 | 483 |
April 2020 | 684 |
May 2020 | 271 |
June 2020 | 322 |
July 2020 | 248 |
August 2020 | 302 |
September 2020 | 392 |
October 2020 | 317 |
November 2020 | 408 |
December 2020 | 366 |
January 2021 | 228 |
February 2021 | 261 |
March 2021 | 299 |
April 2021 | 225 |
May 2021 | 241 |
June 2021 | 141 |
July 2021 | 106 |
August 2021 | 133 |
September 2021 | 284 |
October 2021 | 203 |
November 2021 | 171 |
December 2021 | 126 |
January 2022 | 111 |
February 2022 | 201 |
March 2022 | 190 |
April 2022 | 196 |
May 2022 | 215 |
June 2022 | 112 |
July 2022 | 91 |
August 2022 | 89 |
September 2022 | 114 |
October 2022 | 178 |
November 2022 | 226 |
December 2022 | 171 |
January 2023 | 124 |
February 2023 | 251 |
March 2023 | 287 |
April 2023 | 218 |
May 2023 | 151 |
June 2023 | 93 |
July 2023 | 97 |
August 2023 | 143 |
September 2023 | 202 |
October 2023 | 281 |
November 2023 | 217 |
December 2023 | 226 |
January 2024 | 191 |
February 2024 | 205 |
March 2024 | 194 |
April 2024 | 250 |
May 2024 | 224 |
June 2024 | 120 |
July 2024 | 82 |
August 2024 | 68 |
September 2024 | 142 |
Email alerts
Citing articles via.
- Recommend to your Library
Affiliations
- Online ISSN 1557-7023
- Print ISSN 1540-7063
- Copyright © 2024 The Society for Integrative and Comparative Biology
- About Oxford Academic
- Publish journals with us
- University press partners
- What we publish
- New features
- Open access
- Institutional account management
- Rights and permissions
- Get help with access
- Accessibility
- Advertising
- Media enquiries
- Oxford University Press
- Oxford Languages
- University of Oxford
Oxford University Press is a department of the University of Oxford. It furthers the University's objective of excellence in research, scholarship, and education by publishing worldwide
- Copyright © 2024 Oxford University Press
- Cookie settings
- Cookie policy
- Privacy policy
- Legal notice
This Feature Is Available To Subscribers Only
Sign In or Create an Account
This PDF is available to Subscribers Only
For full access to this pdf, sign in to an existing account, or purchase an annual subscription.

TOBIE SMITH, MD, MPH, Georgetown University Medical Center, Washington, District of Columbia
MATTHEW FEDORUK, PhD, AND AMY EICHNER, PhD, U.S. Anti-Doping Agency, Colorado Springs, Colorado
Am Fam Physician. 2021;103(4):203-204
Author disclosure: No relevant financial affiliations.
Family physicians may be surprised to learn the number of their patients who use performance-enhancing drugs, either deliberately to improve athletic performance or unknowingly through contaminated dietary supplements. Elite athletes account for only a small fraction of the approximately 3 million users of ergogenic drugs in the United States. 1 Sports organizations have broadened their efforts to detect and deter doping (i.e., the use of performance-enhancing drugs in competitive sports) at all levels of competition, resulting in a surprising number of positive doping test results in masters and recreational level athletes. 2 Recreational athletes who have been caught intentionally doping have reported many reasons for doing so, including keeping up with others in their age group in training and competition and for faster recovery from training and competitions so that they can continue to compete at a maximal level. 2 – 4
The prevalence of performance-enhancing drug use among athletes and the general public has led the World Health Organization to recognize the use of these drugs as a public health issue. 3 Over the past decade, the emergence of novel doping agents (particularly drugs aimed at boosting endogenous hormone levels and anabolic agents), increased acceptance of complementary and lifestyle medications, and increased contamination of dietary supplements have contributed to this growing public health concern.
The physical and psychological adverse effects of anabolic androgenic steroids (e.g., kidney and liver damage, acne, gynecomastia, suppression of normal testosterone production, aggression, depression) are well established. What physicians may not recognize are the potential adverse effects of novel, investigational drugs that are being used as doping agents. These include selective androgen receptor modulators (e.g., the investigational drugs ostarine [Enobosarm] and LGD-4033 [Ligandrol]), which have substantial anabolic effects on muscle and bone and significant potential for misuse in sports. These modulators are not approved for human use, and the adverse effects have not been well documented because they are still in clinical trials. Despite their experimental status, selective androgen receptor modulators have been found in dozens of dietary supplements and have caused more than 250 positive doping test results since 2010. 5 – 7 Growth hormone (GH) fragments (e.g., AOD-9604) and GH-releasing peptides, GH secretagogues (e.g., ibutamoren), metabolic modulators (e.g., meldonium), off-market drugs (e.g., sibutramine [Meridia]), and a handful of illegal designer stimulants (e.g., higenamine, 8 methylhexanamine, 9 octodrine 10 ) and other small molecules have also emerged on the doping stage. Consumers can easily buy all of these on the internet.
Even for the astute family physician, it can be difficult to identify patients who are using performance-enhancing drugs. Patients taking dietary supplements may be unintentionally ingesting performance-enhancing drugs because of contamination, and patients commonly do not disclose use of dietary supplements to their physicians. 11 Patients are less likely to disclose supplement use if the physician does not ask about it or if they believe that their physician is not knowledgeable about supplements. 11 Despite anti-doping agencies' warnings to elite athletes about supplement contamination risks, nonelite athletes are rarely educated about these risks.
Patients who are deliberately using performance-enhancing drugs may not disclose use because of shame, legality concerns, or lack of trust. In fact, users of performance-enhancing drugs often are not candid with their physicians about their use of these drugs. In one study, 56% of anabolic steroid users reported that they had never disclosed their use to their physician. 7 The adverse effects of many of the novel performance-enhancing drugs are not well documented or understood and thus may not trigger red flag findings on clinical history and physical examination that would alert a family physician to potential users of these drugs.
Patronage of wellness and antiaging clinics may also put recreational athletes at risk of inadvertent positive doping test results because treatments prescribed at these centers often include hormone replacement. Athletes can apply for a therapeutic use exemption for certain prescribed medications that are prohibited in competition (i.e., beta 2 agonists for asthma, glucocorticoids for inflammatory diseases, hormones for endocrine deficiencies); no exemptions are typically given for medications prescribed solely for symptom relief, antiaging purposes, or other purported health and wellness benefits (i.e., testosterone to treat “low” testosterone levels or nonspecific symptoms).
The family physician is a critical player in addressing the use of performance-enhancing drugs in recreational athletes of all ages. Family physicians should continue to be alert to signs of use of traditional performance-enhancing drugs, such as anabolic-androgenic steroids and stimulants, and also be aware of the emergence and accessibility of novel doping agents. In addition to the potential health risks of the performance-enhancing drug itself, harms of a positive doping test result can include the negative health and social impacts of sanctions prohibiting participation and the potential emotional damage related to being labeled a cheater.
Physicians should be aware of the competition status of athletic patients and consult the appropriate banned substances list (e.g., the World Anti-Doping Agency prohibited list) before prescribing medication and also understand the therapeutic use exemption process ( Table 1 ) . Family physicians should also be aware of the emergence of novel performance-enhancing drugs and their use among the general population; screen patients for use; and be prepared to discuss the safety, effectiveness, legality, and ethics of performance-enhancing drug use. 12
Global Drug Reference Online | Database for the anti-doping status of medications |
U.S. Anti-Doping Agency | |
Therapeutic use exemption: frequently asked questions and application process | |
Online educational module for health care professionals (CME credits available through Stanford University) | |
Information on dietary supplements, including a list of dietary supplements at high risk of contamination | |
Drug reference and email support for anti-doping and medication information | |
World Anti-Doping Agency | Anti-doping e-learning platform, including a module for health care professionals |
Pope HG, Kanayama G, Athey A, et al. The lifetime prevalence of anabolic-androgenic steroid use and dependence in Americans: current best estimates. Am J Addict. 2014;23(4):371-377.
Henning AD, Dimeo P. The complexities of anti-doping violations: a case study of sanctioned cases in all performance levels of USA cycling. Perform Enhanc Health. 2014;3(3–4):159-166.
Dreier F. Wider testing reveals doping among amateur cyclists, too. July 27, 2012. Accessed November 2, 2020. https://www.nytimes.com/2012/07/28/sports/cycling/doping-in-cycling-reaches-into-amateur-ranks.html
Henning AD, Dimeo P. The new front in the war on doping: amateur athletes. Int J Drug Policy. 2018;51:128-136.
Van Wagoner RM, Eichner A, Bhasin S, et al. Chemical composition and labeling of substances marketed as selective androgen receptor modulators and sold via the internet [published correction appears in JAMA . 2018; 319(7):724]. JAMA. 2017;318(20):2004-2010.
U.S. Anti-Doping Agency. Supplement 411: realize, recognize, reduce. High-risk supplement list; 2019. Accessed April 24, 2020. https://www.supplement411.org
World Anti-Doping Agency. Anti-doping testing figures report. December 20, 2019. Accessed April 24, 2020. https://www.wada-ama.org/en/resources/laboratories/anti-doping-testing-figures-report
Cohen PA, Travis JC, Keizers PHJ, et al. The stimulant higenamine in weight loss and sports supplements. Clin Toxicol (Phila). 2019;57(2):125-130.
Eliason MJ, Eichner A, Cancio A, et al. Case reports: death of active duty soldiers following ingestion of dietary supplements containing 1,3-dimethylamylamine (DMAA). Mil Med. 2012;177(12):1455-1459.
Cohen PA, Travis JC, Keizers PHJ, et al. Four experimental stimulants found in sports and weight loss supplements: 2-amino-6-methylheptane (octodrine), 1,4-dimethylamylamine (1,4-DMAA), 1,3-dimethylamylamine (1,3-DMAA) and 1,3-dimethylbutylamine (1,3-DMBA). Clin Toxicol (Phila). 2018;56(6):421-426.
Guzman JR, Paterniti DA, Liu Y, et al. Factors related to disclosure and nondisclosure of dietary supplements in primary care, integrative medicine, and naturopathic medicine. J Fam Med Dis Prev. 2019;5(4) ):10.23937/2469-5793/1510109.
Jenkinson DM, Harbert AJ. Supplements and sports. Am Fam Physician. 2008;78(9):1039-1046. Accessed November 2, 2020. https://www.aafp.org/afp/2008/1101/p1039.html
Continue Reading
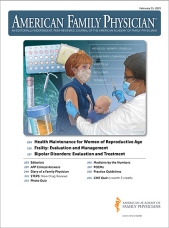
More in AFP
More in pubmed.
Copyright © 2021 by the American Academy of Family Physicians.
This content is owned by the AAFP. A person viewing it online may make one printout of the material and may use that printout only for his or her personal, non-commercial reference. This material may not otherwise be downloaded, copied, printed, stored, transmitted or reproduced in any medium, whether now known or later invented, except as authorized in writing by the AAFP. See permissions for copyright questions and/or permission requests.
Copyright © 2024 American Academy of Family Physicians. All Rights Reserved.

An official website of the United States government
Here’s how you know
Official websites use .gov A .gov website belongs to an official government organization in the United States.
Secure .gov websites use HTTPS A lock ( Lock Locked padlock icon ) or https:// means you’ve safely connected to the .gov website. Share sensitive information only on official, secure websites.
Anabolic Steroids and Other Appearance and Performance Enhancing Drugs (APEDs)
- Anabolic-androgenic steroids are the best-studied class of appearance and performance enhancing drugs (APEDs). APEDs are used to improve appearance by building muscle mass or to enhance athletic performance.
- Although anabolic steroids and other APEDs may directly and indirectly have effects on a person’s mood, they do not typically produce a euphoric high. However, people who use these substances may develop a substance use disorder, defined as continued use despite adverse consequences.
- Anabolic steroids can cause severe, long-lasting, and in some cases, irreversible damage. They can lead to early heart attacks, strokes, liver tumors, kidney failure, and psychiatric problems. In addition, stopping steroid use can cause depression, often leading to resumption of use.
What are anabolic steroids and other appearance and performance enhancing drugs (APEDs)?
Anabolic-androgenic steroids , often shortened to "anabolic steroids," "steroids," or "androgens," 2,3 are the most widely misused APED. These are synthetic substances similar to the male sex hormone testosterone. They promote the growth of skeletal muscle (anabolic effects) and the development of male sexual characteristics (androgenic effects) in both males and females. 2
These compounds are sometimes used medically to treat delayed puberty and muscle loss due to disease 4 and to treat low levels of testosterone in men with an associated medical condition. 5 Anabolic androgenic steroids can also improve feelings of well-being and increase bone strength, but are not approved for these purposes. However, testosterone-supplementation therapy is an increasingly common treatment for mood and sexual performance problems associated with male aging, and it is controversially being prescribed even for younger men. 6
Note that in the context of this report, anabolic steroids refer only to the non-prescribed use (misuse) of testosterone and testosterone-like substances by athletes and non-athlete bodybuilders. This research report will not cover image enhancers, such as dermal fillers , Botox , or the skin tanner, melanotan . 7
Non-steroidal anabolics , include insulin, insulin-like growth hormone (IGF), and human growth hormone (HGH)—substances that are produced by the human body and are prescribed for legitimate medical uses but also sometimes misused for performance enhancement.
Ergo/thermogenics are compounds used to decrease body fat or to promote leanness versus muscle mass in endurance athletes. 8 The three main categories of ergo/thermogenics are:
- Xanthines : compounds that increase attention and wakefulness and suppress appetite. Examples are caffeine, the asthma drug theophylline, and theobromine—a substance found in chocolate, coffee, and tea. 9
- Sympathomimetics : drugs that are similar in structure and action to epinephrine and norepinephrine—natural chemicals in the body that increase heart rate, constrict blood vessels, and raise blood pressure. An example is ephedrine, which is derived from the ephedra plant. Ephedrine/ephedra used to be included in dietary supplements that promoted weight loss, increased energy, and enhanced athletic performance. 10 In 2004, the FDA banned the U.S. sale of dietary supplements containing ephedrine/ephedra due to various possible health risks including cardiovascular and nervous system effects. 11
- Thyroid hormones : substances that regulate metabolism by altering the function of the thyroid. 12 Cytomel is an example.
Nutritional/dietary supplements are substances purchased legally from nutritional stores or via the internet that are often taken in combination with other APEDs. Creatine, which boosts exercise capacity, is one common example.
In the United States, dietary supplements containing steroid precursors such as tetrahydrogestrinone (THG) and androstenedione previously could be purchased legally without a prescription. Athletes took steroid precursors in an effort to boost testosterone levels. Less is known about the side effects of steroid precursors, but if large quantities of these compounds substantially increase testosterone levels in the body, then they also are likely to produce the same side effects as anabolic steroids themselves. 13 The purchase of these supplements, with the notable exception of dehydroepiandrosterone (DHEA), became illegal after the passage of the Anabolic Steroid Control Act of 2004, which amended the Controlled Substances Act. 14
What is the scope of anabolic steroid use in the United States?
All data refer to the United States population.
It is difficult to estimate the prevalence of steroid misuse in the United States because many national surveys that ask about drug use do not include questions about steroids. However, data on steroid misuse among young students are available from the NIDA-supported Monitoring the Future Survey .
How many young students use steroids?
In 2022, an estimated 0.8% of 8th graders, 0.5% of 10th graders, and 1.3% of 12th graders reported misusing steroids in the past 12 months. Source: 2022 Monitoring the Future Survey .
Why are anabolic steroids misused?
Anabolic steroids increase lean muscle mass when used in conjunction with weight training. The aim, for non-athlete weightlifters, is typically improvement of appearance. Steroid use is often associated with a form of male body dysmorphic disorder called muscle dysmorphia, a preoccupation with the perceived inadequate size of their muscles. 19
As a result, some users report taking anabolic steroids to increase confidence and because they feel that they are at a point where they can no longer get bigger through weight training alone. Most users report that anabolic steroids help them achieve their ideal body. 28
Increasing muscle mass may also promote strength, which can improve performance in certain types of sports. More benefit is seen for strength-dependent sports (weightlifting, shot-put throwing, football) than for sports that require speed, agility, flexibility, and/or endurance. 29
Anabolic steroid users also report that their muscles recover faster from intense strain and muscle injury. 30 Research in animals has not conclusively supported this belief, with some showing that anabolic steroids can enhance recovery from certain types of muscle damage, 31,32 but others finding no benefit in taking anabolic steroids to enhance muscle recovery. 33
Anabolic steroid users report using an average of about 11 APEDs per year. They are also more likely than non-steroid users to take supplements such as protein powders and creatine; estrogen blockers; ergo/thermogenics, such as caffeine or ephedrine; medications for erectile dysfunction; and other hormones such as insulin, thyroid hormones, and human growth hormone. 26
How are anabolic steroids used?
Commonly misused steroids.
- Anadrol (oxymetholone)
- Anavar (oxandrolone)
- Dianabol (methandienone )
- Winstrol (stanozolol)
- Restandol (testosterone undecanoate)
Injectable Steroids
- Deca-Durabolin (nandrolone decanoate)
- Durabolin (nandrolone phenpropionate)
- Depo-Testosterone (testosterone cypionate)
- Agovirin (testosterone propionate)
- Retandrol (testosterone phenylpropionate)
- Equipoise (boldenone undecylenate) 29,34
Cycling, stacking, pyramiding, and plateauing
Steroids are often used in patterns called "cycling." This involves taking multiple doses of steroids over a specific period of time, stopping for a period, and starting again. People who misuse steroids also typically "stack" the drugs, meaning that they take two or more different anabolic steroids, mix oral and/or injectable types, and sometimes even take compounds that are designed for veterinary use. 37,38 The belief is that different steroids interact to produce an effect on muscle size that is greater than the effects of each drug individually, 36 a theory that has not been tested scientifically.
Another common mode of steroid misuse is referred to as "pyramiding," which typically involves taking them in a cycle of six to 12 weeks, tapering gradually rather than starting and finishing a cycle abruptly. At the beginning of a cycle, the person starts with low doses of the drugs being stacked and then slowly increases the doses. In the second half of the cycle, the doses are slowly decreased to zero. This is sometimes followed by a second cycle in which the person continues to train but without drugs. Steroid users believe that pyramiding allows the body time to adjust to the high doses, and the drug-free cycle allows the body's hormonal system time to recuperate. 2
A technique called "plateauing" may also be used, whereby steroids are staggered, overlapped, or substituted with another type of steroid to avoid developing tolerance. 36 As with stacking, the effects of pyramiding, cycling, and plateauing have not been substantiated scientifically.
What are the side effects of anabolic steroid misuse?
A variety of side effects can occur when anabolic steroids are misused, ranging from mild effects to ones that are harmful or even life-threatening. Most are reversible if the user stops taking the drugs. However, others may be permanent or semi-permanent.
Most data on the long-term effects of anabolic steroids in humans come from case reports rather than formal epidemiological studies. Serious and life-threatening adverse effects may be underreported, especially since they may occur many years later. One review found 19 deaths in published case reports related to anabolic steroid use between 1990 and 2012; however, many steroid users also used other drugs, making it difficult to show that the anabolic steroid use caused these deaths. 39 One animal study found that exposing male mice for one fifth of their lifespan to steroid doses comparable to those taken by human athletes caused a high frequency of early deaths. 40
Cardiovascular System
Steroid use has been associated with high blood pressure; 41 decreased function of the heart’s ventricles; 23,41,42 and cardiovascular diseases such as heart attacks, 43 artery damage, 44 and strokes, 45,46 even in athletes younger than 30. Steroids contribute to the development of cardiovascular disease partly by increasing the level of low-density lipoprotein (LDL) 47 and decreasing the level of high-density lipoprotein (HDL). 47,48 High LDL and low HDL levels increase the risk of atherosclerosis, a condition in which fatty substances are deposited inside arteries and disrupt blood flow. If blood is prevented from reaching the heart or brain, the result can be a heart attack or stroke, respectively. Steroids also increase the risk that blood clots will form in blood vessels, potentially disrupting blood flow and damaging the heart muscle, so that it does not pump blood effectively. 49
Hormonal System
Steroid use disrupts the normal production of hormones in the body. Changes that can be reversed include decreased sperm production, 56–59 decreased function of the testes (hypogonadism) that leads to low testosterone levels, 60 and shrinking of the testicles (testicular atrophy). 56,61 Irreversible changes include male-pattern baldness and breast development (gynecomastia) in men. 59,62 Anabolic steroids may also act upon the hormone system to increase the risk of testicular cancer, especially when steroids are used in combination with insulin-like growth factor. 63
In females, anabolic steroids cause masculinization. Specifically, breast size and body fat decrease, the skin becomes coarse, and the voice deepens. 64 Women may experience excessive growth of body hair but lose scalp hair. 65 With continued administration of steroids, some of these effects become irreversible. It is commonly believed that anabolic steroids will produce irreversible enlargement of the clitoris in females, although there are no studies on this. 66
Many people who inject anabolic steroids may use nonsterile injection techniques or share contaminated needles with other users. This puts these steroid users at risk for acquiring life threatening viral infections, such as HIV and hepatitis B and C. 76 In addition, animal models indicate that anabolic steroids suppress the immune system, 77 which could worsen infections.
Steroid misuse has been associated with liver damage, 50,51 tumors, 46,52,53 and a rare condition called peliosis hepatis, in which blood-filled cysts form in the liver. 54 The cysts can rupture, causing internal bleeding and even death in rare cases. 55
Musculoskeletal System
Rising levels of testosterone and other sex hormones normally trigger the growth spurt that occurs during puberty and adolescence. These rising levels of testosterone also provide the signals to stop growth. 67 When a child or adolescent takes anabolic steroids, the resulting artificially high sex hormone levels can prematurely signal the bones to stop growing. 68
Evidence suggests that weightlifters who misuse anabolic steroids have stiffer tendons, which could lead to an increased risk for tendon injury. 69
Steroid misuse can cause acne, 70–72 hair loss on the head, cysts, and oily hair and skin. 65 Users who inject steroids may also develop pain and abscess formation at injection sites. 73
Anabolic steroids can also produce jaundice, or yellowing of the skin or eyes, as a result of damage to the liver. 74,75
How does anabolic steroid misuse affect behavior?
Case reports and small studies indicate that anabolic steroids increase irritability and aggression, 75 although findings may be confounded by personality traits that are overrepresented in steroid users (i.e., antisocial, borderline, and histrionic personality disorder) 78 and use of other drugs. 79 People who misuse anabolic steroids report more anger than nonusers, 80 as well as more fights, verbal aggression, and violence toward their significant others, 81 sometimes called "roid rage." One study suggests that the mood and behavioral effects seen during anabolic-androgenic steroid misuse may result from secondary hormonal changes. 82
Scientists have attempted to test the association between anabolic steroids and aggression by administering high steroid doses or placebo for days or weeks to human volunteers and then assessing behavioral symptoms. In one such study, researchers found that testosterone over a six week period was associated with increased aggression, as assessed by a questionnaire and computer-based model of aggressive behavior. 83 In addition, high steroid doses produced greater feelings of irritability and aggression than placebo, 84 although the effects appear to be highly variable across individuals, 19 and other studies have not shown that effect. 85 One possible explanation, according to the researchers, is that some but not all anabolic steroids increase irritability and aggression.
Psychiatric Disorders
Anabolic steroid users are more likely than nonusers to report anxiety. 34,86 Moderate to high doses of anabolic steroids are also associated with major mood disorders such as mania, hypomania, 87 and major depression. 86,87 In one study, manic symptoms were not uniform across individuals, with most showing little psychological change, whereas a few demonstrated prominent effects. 19
Other Drug Use
Anabolic steroid users are more likely to use drugs such as marijuana, prescription opioids, cocaine, 88 or heroin. 86 In a study of men admitted to treatment for opioid use disorders, 25 percent reported prior use of anabolic steroids. Some described first learning about opioids from friends at the gym, and that they first purchased opioids from the same person who had sold them the anabolic steroids. 89 In a study of anabolic steroid users dependent upon the injectable opioid analgesic nalbuphine, most reported that they began using nalbuphine to treat pain from weightlifting injuries. They also described widespread use of nalbuphine in their gyms. 90
Research also indicates that some users might turn to other drugs to alleviate some of the negative effects of anabolic steroids. For example, a study of 227 men admitted in 1999 to a private treatment center for addiction to heroin or other opioids found that 9.3 percent had previously misused anabolic steroids. Of these, most reported using opioids to counteract insomnia, irritability, depression, and withdrawal from anabolic steroids. 91
What are the risks of anabolic steroid use in teens?
Unlike most illicit drug use, misuse of anabolic steroids most commonly begins in young adulthood rather than adolescence. But steroid use in teens is of concern, especially since the hormonal systems they interact with play a critical role in brain development during these years. 92–96 In adolescent rodents, exposure to anabolic steroids increased neuronal spine densities in the hippocampus and amygdala—brain regions involved in learning and emotions (e.g., aggression), respectively. Four weeks after withdrawal, these increases in neuronal spine densities returned to normal in the amygdala, but not in the hippocampus. This suggests that pubertal steroid exposure could produce long-lasting structural changes in certain brain regions. 97
Teens who use anabolic steroids may also be at increased risk for some cognitive side effects compared with adults. For example, males who begin using anabolic steroids during the teen years show increased impulsivity and decreased attention, compared to men who began using steroids in their adult years. 98 In adolescent rats, anabolic steroid exposure is associated with electrolytic imbalances, hyperactivity, anxiety, and increased sympathetic autonomic modulation (e.g., fight or flight response) during adulthood, even when steroid use was discontinued during adolescence. 99 In addition, adolescent male hamsters given anabolic steroids show increased aggression, even after steroid use is discontinued. These aggressive effects are paralleled by changes in levels of serotonin 100,101 and androgen receptors in the rodent brain. 102
How do anabolic steroids work in the brain?
Anabolic steroids act at androgen receptors to influence cellular functioning and gene expression. In addition to regulating pathways involved in the development of male characteristics, 103 activation of androgen receptors also produces rapid increases in calcium levels within skeletal muscle, heart, and brain cells. 104 Calcium plays important roles in neuronal signaling.
Research with human cells demonstrates that anabolic steroids also interact with certain types of GABA A receptors, which could mediate the increased anxiety reported by steroid users. 105,106 In addition, animal studies show that anabolic steroids increase serotonin levels in brain regions involved in mood 107 and dopamine levels in reward-related brain regions. 107,108 Chronic use of anabolic steroids has also been shown to cause dysfunction of these reward pathways in animals. Specifically, rats given twice daily nandrolone injections for four weeks showed loss of sweet preference (a sign of reward dysfunction) that was accompanied by reductions of dopamine, serotonin, and noradrenaline in the nucleus accumbens, a reward-related brain region. 109
Are anabolic steroids addictive?
An undetermined percentage of steroid users may develop a steroid use disorder. Substance use disorders are defined by continued use despite adverse consequences; for steroid users, these may include physical or psychological problems such as breast growth (in men), sexual dysfunction, high blood pressure, excessive fats in the blood, heart disease, mood swings, severe irritability, or aggressiveness. Anabolic steroid users also may give up other important activities for fear that they will miss workouts, violate their dietary restrictions, or be prevented from using steroids. Steroid users also typically spend large amounts of time and money obtaining the drugs, and they may try to reduce or stop anabolic steroid use without success—possibly due to depression, anxiety about losing muscle mass, or and other unpleasant effects of withdrawal. 110
Withdrawal from steroids occurs when an individual develops dependence. A review of the research suggests that about 32 percent of people who misuse anabolic steroids become dependent. 23 Symptoms of dependence can include tolerance, which is needing to take more steroids to achieve the same effects. Another indicator of dependence is withdrawal once anabolic steroid use stops. 110 Withdrawal symptoms can include fatigue, restlessness, loss of appetite, insomnia, reduced sex drive, and steroid cravings. 111 The most dangerous of the withdrawal symptoms is depression, because it sometimes leads to suicide attempts. 112
How are anabolic steroids tested in athletes?
Although non-athlete weightlifters account for the bulk of anabolic steroid misuse, occasional steroid use by professional and Olympic athletes to improve performance or cheat in competition ("doping") has done the most to raise awareness of steroid misuse. The World Anti-Doping Agency (WADA) was founded in 1999 to consistently apply anti-doping policies across sports organizations and governments around the world. Non-compliant organizations can face sanctions such as event cancellation, loss of WADA funding, or ineligibility to host events. 114
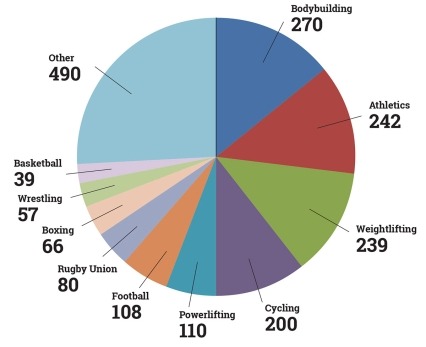
Refinements in drug testing have improved the ability to detect anti-doping violations, resulting in increased numbers of reported violations over recent years. For example, the discovery of long-term steroid metabolites has lengthened the drug detection window, making it more difficult for athletes to pass drug tests by simply discontinuing steroid use just prior to an event. In addition, more sensitive technologies have allowed detection of lower metabolite thresholds. 115
Although testing procedures are now in place to deter steroid use among professional and Olympic athletes, new designer drugs constantly become available that can escape detection and put athletes willing to cheat one step ahead of testing efforts. 116–118 To detect early use of designer steroids and provide more accurate baseline standards for each athlete, testing laboratories store data from each drug testing sample. These samples are then used as reference points for future testing, thereby eliminating the possibility that a person tests positive simply because he or she has naturally elevated levels of testosterone when compared to the general population. 119 Long-term use of designer steroids suppresses levels endogenous steroids in urine samples, which could be the first indication that an athlete is taking a designer steroid. 117

Drug Testing and Nutritional Supplements
Athletes taking over-the-counter nutritional supplements may believe that such products are safe. However, nutritional supplements are not subjected to the same pre-approval requirements and quality tests as FDA-approved medications. 120 For example, some supplements advertised to promote weight loss have been found to contain banned stimulants such as ephedrine 121 or clenbuterol. 122 Other research shows that supplements sometimes contain prohormones or anabolic steroids. 123 In a study looking at 634 nutritional supplements from 13 different countries, 15 percent included some type of prohormone not listed on the label. 115 Another study showed that some non-labeled prohibited substances could be detected by drug tests up to 144 hours later. 124
Nutritional supplements sometimes contain banned substances that are not indicated in their labels. 115,124 The FDA notes that consumers should be wary if a product meets any of these criteria:
- products claiming to be alternatives to FDA-approved drugs or to have effects similar to prescription drugs
- products claiming to be a legal alternative to anabolic steroids
- products that are marketed primarily in a foreign language or those that are marketed through mass e-mails
- sexual enhancement products promising rapid effects such as working in minutes to hours, or long-lasting effects such as 24 hours to 72 hours
- products that provide warnings about testing positive in performance enhancement drug tests 125
According to WADA’s codes, athletes are responsible for any prohibited substance found in their samples, regardless of whether ingestion was intentional or unintentional. However, sanctions may be reduced or avoided if the athlete can demonstrate that the substance was ingested through no significant fault or negligence on his/her part, or in some circumstances where the athlete did not intend to enhance performance. 126
What can be done to prevent steroid misuse?
Research suggests that high school athletes are less likely to use steroids if their peers and parents disapprove, indicating that peers and parents can be strong partners in prevention efforts. 127
However, research shows that simply teaching students about steroids' adverse effects does not convince adolescents that they will be adversely affected, nor does such instruction discourage young people from taking steroids in the future. Presenting both the risks and benefits of anabolic steroid use is more effective in convincing adolescents about steroids' negative effects, apparently because the students find a balanced approach more credible. 128
Research also indicates that some adolescents misuse steroids as part of a pattern of high-risk behaviors such as drinking and driving, carrying a gun, driving a motorcycle without a helmet, and using other illicit drugs. This suggests that a prevention program should focus on comprehensive high-risk behavior screening and counseling among teens who use anabolic steroids. 129
NIDA-Funded Prevention Research Helps Reduce Steroid Misuse
Studies show that one year after completion of the program, compared with a control group, ATLAS-trained football student athletes in 15 high schools had:
- less use of anabolic steroids and less intention to misuse them in the future
- less misuse of alcohol, amphetamines, and narcotics
- less misuse of "athletic enhancing" supplements
- less likelihood of engaging in hazardous behaviors such as drinking and driving
- better knowledge about anabolic steroid, alcohol, and marijuana effects; better knowledge of alternatives to steroid misuse; greater confidence in athletic abilities; and improved nutritional behaviors 130
What treatments are effective for anabolic steroid misuse?
People who use steroids often do not seek treatment for their use, with one study reporting that 56 percent of users had never told their physician about their use. 133 This could be because users feel their physician lacks knowledge about anabolic steroids. 133 In addition, many internet sites devoted to anabolic steroids and other APEDs challenge the professionalism of health care providers and offer their own medically questionable advice on the use of APEDs. 134 This makes it important for health care providers to be educated on the signs and symptoms of steroid use in their patients. 111
Current views recommend that treatment for steroid use address the underlying causes of the steroid use. This can include:
- psychological therapies (and possibly medications) for muscle dysmorphia
- endocrine therapies to restore function in those suffering from hypogonadism and to alleviate symptoms of depression
- antidepressants for those whose depression does not respond to endocrine therapies
- pharmacological and psychosocial treatments for patients who are also dependent on opioids, which appear to also be effective in alleviating signs of anabolic steroid dependence 135
Find More Resources on Anabolic Steroids and Other Appearance and Performance Enhancing Drugs
- Learn more about steroids and their legal status from the U.S. Drug Enforcement Administration
- Review patient resources on anabolic steroids from MedlinePlus .
- Explore publications about steroids from the U.S. Substance Abuse and Mental Health Services Administration.
- Kanayama G, Pope HG. History and epidemiology of anabolic androgens in athletes and non-athletes. Mol Cell Endocrinol . March 2017. doi:10.1016/j.mce.2017.02.039
- Rashid H, Ormerod S, Day E. Anabolic androgenic steroids: what the psychiatrist needs to know. Adv Psychiatr Treat . 2007;13(3):203-211.
- Lipsett MB, Korenman SG. Androgen Metabolism. JAMA . 1964;190(8):757-762. doi:10.1001/jama.1964.03070210063011
- Shahidi NT. A review of the chemistry, biological action, and clinical applications of anabolic-androgenic steroids. Clin Ther . 2001;23(9):1355-1390.
- Testosterone Information. https://www.fda.gov/drugs/drugsafety/ postmarketdrugsafetyinformationforpatientsandproviders/ucm161874.htm . Published March 3, 2015. Accessed May 26, 2017.
- Rao PK, Boulet SL, Mehta A, et al. Trends in Testosterone Replacement Therapy Use from 2003 to 2013 among Reproductive-Age Men in the United States. J Urol . 2017;197(4):1121-1126. doi:10.1016/j.juro.2016.10.063.
- Brennan R, Wells JSG, Van Hout MC. The injecting use of image and performance-enhancing drugs (IPED) in the general population: a systematic review. Health Soc Care Community . January 2016. doi:10.1111/hsc.12326.
- Hildebrandt T, Langenbucher JW, Carr SJ, Sanjuan P. Modeling population heterogeneity in appearance- and performance-enhancing drug (APED) use: applications of mixture modeling in 400 regular APED users. J Abnorm Psychol . 2007;116(4):717-733. doi:10.1037/0021-843X.116.4.71.
- Gennaro MC, Abrigo C. Caffeine and theobromine in coffee, tea and cola-beverages. Fresenius J Anal Chem . 1992;343(6):523-525.
- Ephedra. NCCIH. https://nccih.nih.gov/health/ephedra . Published November 9, 2011. Accessed November 6, 2017.
- Office of Dietary Supplements. Ephedra. https://ods.od.nih.gov/Health_Information/Ephedra.aspx . Published n.d. Accessed December 13, 2017.
- American Thyroid Association. Thyroid and Weight FAQ. June 2012. http://www.thyroid.org/thyroid-and-weight/ . Accessed November 6, 2017.
- 108th Congress FS. Regulation of Dietary Supplements: Hearing Before the Committee on Commerce, Science and Transportation .; 2003. https://www.gpo.gov/fdsys/pkg/CHRG-108shrg20196/pdf/CHRG-108shrg20196.pdf . Accessed February 7, 2018.
- 108th Congress. Anabolic Steroid Control Act of 2004 . Vol S.2195.; 2004. https://www.congress.gov/bill/108th-congress/senate-bill/2195/all-info . Accessed April 28, 2017.
- Freeman ER, Bloom DA, McGuire EJ. A brief history of testosterone. J Urol . 2001;165(2):371-373. doi:10.1097/00005392-200102000-00004.
- Altschule MD, Tillotson KJ. The use of testosterone in the treatment of depressions. N Engl J Med . 1948;239(27):1036-1038. doi:10.1056/NEJM194812302392704.
- Wade N. Anabolic Steroids: Doctors Denounce Them, but Athletes Aren’t Listening. Science . 1972;176(4042):1399-1403. doi:10.1126/science.176.4042.1399.
- Buckley WE, Yesalis CE, Friedl KE, Anderson WA, Streit AL, Wright JE. Estimated prevalence of anabolic steroid use among male high school seniors. JAMA . 1988;260(23):3441-3445.
- Pope HG, Kouri EM, Hudson JI. Effects of supraphysiologic doses of testosterone on mood and aggression in normal men: a randomized controlled trial. Arch Gen Psychiatry . 2000;57(2):133-140; discussion 155-156.
- Significant Dates in U.S. Food and Drug Law History. 2014. https://www.fda.gov/about-fda/fda-history/milestones-us-food-and-drug-law . Accessed June 4, 2016.
- A Dangerous and Illegal Way to Seek Athletic Dominance and Better Appearance - A Guide for Understanding the Dangers of Anabolic Steroids. March 2004. https://www.deadiversion.usdoj.gov/pubs/brochures/steroids/public/. Accessed April 25, 2017.
- Kanayama G, Boynes M, Hudson JI, Field AE, Pope HG. Anabolic steroid abuse among teenage girls: an illusory problem? Drug Alcohol Depend . 2007;88(2-3):156-162. doi:10.1016/j.drugalcdep.2006.10.013.
- Pope HG, Kanayama G, Athey A, Ryan E, Hudson JI, Baggish A. The lifetime prevalence of anabolic-androgenic steroid use and dependence in Americans: current best estimates. Am J Addict Am Acad Psychiatr Alcohol Addict . 2014;23(4):371-377. doi:10.1111/j.1521-0391.2013.12118.x.
- Irving LM, Wall M, Neumark-Sztainer D, Story M. Steroid use among adolescents: findings from Project EAT. J Adolesc Health Off Publ Soc Adolesc Med . 2002;30(4):243-252.
- Pope HG, Khalsa JH, Bhasin S. Body Image Disorders and Abuse of Anabolic-Androgenic Steroids Among Men. JAMA . 2017;317(1):23-24. doi:10.1001/jama.2016.17441.
- Ip EJ, Barnett MJ, Tenerowicz MJ, Perry PJ. The Anabolic 500 survey: characteristics of male users versus nonusers of anabolic-androgenic steroids for strength training. Pharmacotherapy . 2011;31(8):757-766. doi:10.1592/phco.31.8.757.
- Gruber AJ, Pope HG. Compulsive weight lifting and anabolic drug abuse among women rape victims. Compr Psychiatry . 1999;40(4):273-277.
- Wright S, Grogan S, Hunter G. Motivations for Anabolic Steroid use Among Bodybuilders. J Health Psychol . 2000;5(4):566-571. doi:10.1177/135910530000500413.
- American Academy of Pediatrics. Adolescents and anabolic steroids: a subject review. American Academy of Pediatrics. Committee on Sports Medicine and Fitness. Pediatrics . 1997;99(6):904-908.
- Bahrke MS, Wright JE, Strauss RH, Catlin DH. Psychological moods and subjectively perceived behavioral and somatic changes accompanying anabolic-androgenic steroid use. Am J Sports Med . 1992;20(6):717-724.
- Beiner JM, Jokl P, Cholewicki J, Panjabi MM. The effect of anabolic steroids and corticosteroids on healing of muscle contusion injury. Am J Sports Med . 1999;27(1):2-9.
- Ferry A, Noirez P, Page CL, Salah IB, Daegelen D, Rieu M. Effects of anabolic/androgenic steroids on regenerating skeletal muscles in the rat. Acta Physiol Scand . 1999;166(2):105-110. doi:10.1046/j.1365-201x.1999.00549.x.
- Ferry A, Vignaud A, Noirez P, Bertucci W. Respective effects of anabolic/androgenic steroids and physical exercise on isometric contractile properties of regenerating skeletal muscles in the rat. Arch Physiol Biochem . 2000;108(3):257-261. doi:10.1076/1381345520000710831ZFT257.
- Eklöf A-C, Thurelius A-M, Garle M, Rane A, Sjöqvist F. The anti-doping hot-line, a means to capture the abuse of doping agents in the Swedish society and a new service function in clinical pharmacology. Eur J Clin Pharmacol . 2003;59(8-9):571-577. doi:10.1007/s00228-003-0633-z.
- Medline Plus. Testosterone Topical. June 2016. https://www.nlm.nih.gov/medlineplus/druginfo/meds/a605020.html#why .
- Trenton AJ, Currier GW. Behavioural manifestations of anabolic steroid use. CNS Drugs . 2005;19(7):571-595.
- Evans NA. Gym and tonic: a profile of 100 male steroid users. Br J Sports Med . 1997;31(1):54-58.
- Wilson JD. Androgen abuse by athletes. Endocr Rev . 1988;9(2):181-199. doi:10.1210/edrv-9-2-181.
- Frati P, Busardò FP, Cipolloni L, Dominicis ED, Fineschi V. Anabolic Androgenic Steroid (AAS) related deaths: autoptic, histopathological and toxicological findings. Curr Neuropharmacol . 2015;13(1):146-159. doi:10.2174/1570159X13666141210225414.
- Bronson FH, Matherne CM. Exposure to anabolic-androgenic steroids shortens life span of male mice. Med Sci Sports Exerc . 1997;29(5):615-619.
- Urhausen A, Albers T, Kindermann W. Are the cardiac effects of anabolic steroid abuse in strength athletes reversible? Heart Br Card Soc . 2004;90(5):496-501.
- Kaskutas LA. Alcoholics anonymous effectiveness: faith meets science. J Addict Dis . 2009;28(2):145-157. doi:10.1080/10550880902772464.
- Vanberg P, Atar D. Androgenic anabolic steroid abuse and the cardiovascular system. Handb Exp Pharmacol . 2010;(195):411-457. doi:10.1007/978-3-540-79088-4_18.
- Baggish AL, Weiner RB, Kanayama G, et al. Cardiovascular Toxicity of Illicit Anabolic-Androgenic Steroid Use. Circulation . 2017;135(21):1991-2002. doi:10.1161/CIRCULATIONAHA.116.026945.
- El Scheich T, Weber A-A, Klee D, Schweiger D, Mayatepek E, Karenfort M. Adolescent ischemic stroke associated with anabolic steroid and cannabis abuse. J Pediatr Endocrinol Metab JPEM . 2013;26(1-2):161-165. doi:10.1515/jpem-2012-0057.
- Santamarina RD, Besocke AG, Romano LM, Ioli PL, Gonorazky SE. Ischemic stroke related to anabolic abuse. Clin Neuropharmacol . 2008;31(2):80-85. doi:10.1097/WNF.0b013e3180ed4485.
- Palatini P, Giada F, Garavelli G, et al. Cardiovascular effects of anabolic steroids in weight-trained subjects. J Clin Pharmacol . 1996;36(12):1132-1140.
- Bhasin S, Woodhouse L, Casaburi R, et al. Testosterone dose-response relationships in healthy young men. Am J Physiol Endocrinol Metab . 2001;281(6):E1172-1181.
- Linton MF, Yancey PG, Davies SS, Jerome WG (Jay), Linton EF, Vickers KC. The Role of Lipids and Lipoproteins in Atherosclerosis. In: De Groot LJ, Chrousos G, Dungan K, et al., eds. Endotext . South Dartmouth (MA): MDText.com, Inc.; 2000. https://www.ncbi.nlm.nih.gov/books/NBK343489/ . Accessed April 21, 2017.
- Robles-Diaz M, Gonzalez-Jimenez A, Medina-Caliz I, et al. Distinct phenotype of hepatotoxicity associated with illicit use of anabolic androgenic steroids. Aliment Pharmacol Ther . 2015;41(1):116-125. doi:10.1111/apt.13023.
- Schwingel PA, Cotrim HP, Santos CR dos, et al. Recreational Anabolic-Androgenic Steroid Use Associated With Liver Injuries Among Brazilian Young Men. Subst Use Misuse . 2015;50(11):1490-1498. doi:10.3109/10826084.2015.1018550.
- Kosaka A, Takahashi H, Yajima Y, et al. Hepatocellular carcinoma associated with anabolic steroid therapy: report of a case and review of the Japanese literature. J Gastroenterol . 1996;31(3):450-454.
- Socas L, Zumbado M, Pérez-Luzardo O, et al. Hepatocellular adenomas associated with anabolic androgenic steroid abuse in bodybuilders: a report of two cases and a review of the literature. Br J Sports Med . 2005;39(5):e27. doi:10.1136/bjsm.2004.013599.
- Wakabayashi T, Onda H, Tada T, Iijima M, Itoh Y. High incidence of peliosis hepatis in autopsy cases of aplastic anemia with special reference to anabolic steroid therapy. Acta Pathol Jpn . 1984;34(5):1079-1086.
- Hansma P, Diaz FJ, Njiwaji C. Fatal Liver Cyst Rupture Due to Anabolic Steroid Use: A Case Presentation. Am J Forensic Med Pathol . 2016;37(1):21-22. doi:10.1097/PAF.0000000000000218.
- Bonetti A, Tirelli F, Catapano A, et al. Side effects of anabolic androgenic steroids abuse. Int J Sports Med . 2008;29(8):679-687. doi:10.1055/s-2007-965808.
- Liu PY, Swerdloff RS, Christenson PD, Handelsman DJ, Wang C, Hormonal Male Contraception Summit Group. Rate, extent, and modifiers of spermatogenic recovery after hormonal male contraception: an integrated analysis. Lancet Lond Engl . 2006;367(9520):1412-1420. doi:10.1016/S0140-6736(06)68614-5.
- Torres-Calleja J, González-Unzaga M, DeCelis-Carrillo R, Calzada-Sánchez L, Pedrón N. Effect of androgenic anabolic steroids on sperm quality and serum hormone levels in adult male bodybuilders. Life Sci . 2001;68(15):1769-1774.
- Calzada L, Torres-Calleja J, Martinez JM, Pedrón N. Measurement of androgen and estrogen receptors in breast tissue from subjects with anabolic steroid-dependent gynecomastia. Life Sci . 2001;69(13):1465-1469.
- Christou MA, Christou PA, Markozannes G, Tsatsoulis A, Mastorakos G, Tigas S. Effects of Anabolic Androgenic Steroids on the Reproductive System of Athletes and Recreational Users: A Systematic Review and Meta-Analysis. Sports Med Auckl NZ . March 2017. doi:10.1007/s40279-017-0709-z.
- Schürmeyer T, Knuth UA, Belkien L, Nieschlag E. Reversible azoospermia induced by the anabolic steroid 19-nortestosterone. Lancet Lond Engl . 1984;1(8374):417-420.
- Orlandi MA, Venegoni E, Pagani C. Gynecomastia in two young men with histories of prolonged use of anabolic androgenic steroids. J Ultrasound . 2010;13(2):46-48. doi:10.1016/j.jus.2010.07.006.
- Chimento A, Sirianni R, Zolea F, et al. Nandrolone and stanozolol induce Leydig cell tumor proliferation through an estrogen-dependent mechanism involving IGF-I system. J Cell Physiol . 2012;227(5):2079-2088. doi:10.1002/jcp.22936.
- Baker J. A report on alterations to the speaking and singing voices of four women following hormonal therapy with virilizing agents. J Voice Off J Voice Found . 1999;13(4):496-507.
- Scott MJ, Scott AM. Effects of anabolic-androgenic steroids on the pilosebaceous unit. Cutis . 1992;50(2):113-116.
- Nieschlag E, Vorona E. MECHANISMS IN ENDOCRINOLOGY: Medical consequences of doping with anabolic androgenic steroids: effects on reproductive functions. Eur J Endocrinol Eur Fed Endocr Soc . 2015;173(2):R47-58. doi:10.1530/EJE-15-0080.
- Zemel BS, Katz SH. The contribution of adrenal and gonadal androgens to the growth in height of adolescent males. Am J Phys Anthropol . 1986;71(4):459-466. doi:10.1002/ajpa.1330710409.
- Bierich JR. Effects and side effects of anabolic steroids in children. Acta Endocrinol Suppl (Copenh) . 1961;39(Suppl 63):89-110.
- Seynnes OR, Kamandulis S, Kairaitis R, et al. Effect of androgenic-anabolic steroids and heavy strength training on patellar tendon morphological and mechanical properties. J Appl Physiol Bethesda Md 1985 . 2013;115(1):84-89. doi:10.1152/japplphysiol.01417.2012.
- Kraus SL, Emmert S, Schön MP, Haenssle HA. The dark side of beauty: acne fulminans induced by anabolic steroids in a male bodybuilder. Arch Dermatol . 2012;148(10):1210-1212. doi:10.1001/archdermatol.2012.855.
- Melnik B, Jansen T, Grabbe S. Abuse of anabolic-androgenic steroids and bodybuilding acne: an underestimated health problem. J Dtsch Dermatol Ges J Ger Soc Dermatol JDDG . 2007;5(2):110-117. doi:10.1111/j.1610-0387.2007.06176.x.
- Voelcker V, Sticherling M, Bauerschmitz J. Severe ulcerated “bodybuilding acne” caused by anabolic steroid use and exacerbated by isotretinoin. Int Wound J . 2010;7(3):199-201. doi:10.1111/j.1742-481X.2010.00676.x.
- Rich JD, Dickinson BP, Flanigan TP, Valone SE. Abscess related to anabolic-androgenic steroid injection. Med Sci Sports Exerc . 1999;31(2):207-209.
- Cabb E, Baltar S, Powers DW, Mohan K, Martinez A, Pitts E. The Diagnosis and Manifestations of Liver Injury Secondary to Off-Label Androgenic Anabolic Steroid Use. Case Rep Gastroenterol . 2016;10(2):499-505. doi:10.1159/000448883.
- Yoshida EM, Erb SR, Scudamore CH, Owen DA. Severe cholestasis and jaundice secondary to an esterified testosterone, a non-C17 alkylated anabolic steroid. J Clin Gastroenterol . 1994;18(3):268-270.
- Ip EJ, Yadao MA, Shah BM, Lau B. Infectious disease, injection practices, and risky sexual behavior among anabolic steroid users. AIDS Care . 2016;28(3):294-299. doi:10.1080/09540121.2015.1090539.
- Hughes TK, Fulep E, Juelich T, Smith EM, Stanton GJ. Modulation of immune responses by anabolic androgenic steroids. Int J Immunopharmacol . 1995;17(11):857-863.
- Perry PJ, Kutscher EC, Lund BC, Yates WR, Holman TL, Demers L. Measures of aggression and mood changes in male weightlifters with and without androgenic anabolic steroid use. J Forensic Sci . 2003;48(3):646-651.
- Lundholm L, Frisell T, Lichtenstein P, Långström N. Anabolic androgenic steroids and violent offending: confounding by polysubstance abuse among 10,365 general population men. Addict Abingdon Engl . 2015;110(1):100-108. doi:10.1111/add.12715.
- Burnett KF, Kleiman ME. Psychological characteristics of adolescent steroid users. Adolescence . 1994;29(113):81-89.
- Choi PY, Pope HG. Violence toward women and illicit androgenic-anabolic steroid use. Ann Clin Psychiatry Off J Am Acad Clin Psychiatr . 1994;6(1):21-25.
- Daly RC, Su T-P, Schmidt PJ, Pagliaro M, Pickar D, Rubinow DR. Neuroendocrine and behavioral effects of high-dose anabolic steroid administration in male normal volunteers. Psychoneuroendocrinology . 2003;28(3):317-331.
- Kouri EM, Lukas SE, Pope HG, Oliva PS. Increased aggressive responding in male volunteers following the administration of gradually increasing doses of testosterone cypionate. Drug Alcohol Depend . 1995;40(1):73-79.
- Bahrke MS, Yesalis CE, Wright JE. Psychological and behavioural effects of endogenous testosterone and anabolic-androgenic steroids. An update. Sports Med Auckl NZ . 1996;22(6):367-390.
- Tricker R, Casaburi R, Storer TW, et al. The effects of supraphysiological doses of testosterone on angry behavior in healthy eugonadal men--a clinical research center study. J Clin Endocrinol Metab . 1996;81(10):3754-3758. doi:10.1210/jcem.81.10.8855834.
- Ip EJ, Lu DH, Barnett MJ, Tenerowicz MJ, Vo JC, Perry PJ. Psychological and physical impact of anabolic-androgenic steroid dependence. Pharmacotherapy . 2012;32(10):910-919. doi:10.1002/j.1875-9114.2012.01123.
- Pope HG, Katz DL. Psychiatric and medical effects of anabolic-androgenic steroid use. A controlled study of 160 athletes. Arch Gen Psychiatry . 1994;51(5):375-382.
- Kanayama G, Pope HG. Illicit use of androgens and other hormones: recent advances. Curr Opin Endocrinol Diabetes Obes . 2012;19(3):211-219. doi:10.1097/MED.0b013e3283524008.
- Kanayama G, Cohane GH, Weiss RD, Pope HG. Past anabolic-androgenic steroid use among men admitted for substance abuse treatment: an underrecognized problem? J Clin Psychiatry . 2003;64(2):156-160.
- Wines JD, Gruber AJ, Pope HG, Lukas SE. Nalbuphine hydrochloride dependence in anabolic steroid users. Am J Addict . 1999;8(2):161-164.
- Arvary D, Pope HG. Anabolic-androgenic steroids as a gateway to opioid dependence. N Engl J Med . 2000;342(20):1532. doi:10.1056/NEJM200005183422018.
- Keenan BS, Richards GE, Ponder SW, Dallas JS, Nagamani M, Smith ER. Androgen-stimulated pubertal growth: the effects of testosterone and dihydrotestosterone on growth hormone and insulin-like growth factor-I in the treatment of short stature and delayed puberty. J Clin Endocrinol Metab . 1993;76(4):996-1001. doi:10.1210/jcem.76.4.8473416.
- Morris JA, Jordan CL, Breedlove SM. Sexual differentiation of the vertebrate nervous system. Nat Neurosci . 2004;7(10):1034-1039. doi:10.1038/nn1325.
- Romeo RD, Richardson HN, Sisk CL. Puberty and the maturation of the male brain and sexual behavior: recasting a behavioral potential. Neurosci Biobehav Rev . 2002;26(3):381-391.
- Schulz KM, Molenda-Figueira HA, Sisk CL. Back to the future: The organizational-activational hypothesis adapted to puberty and adolescence. Horm Behav . 2009;55(5):597-604. doi:10.1016/j.yhbeh.2009.03.010.
- Zehr JL, Nichols LR, Schulz KM, Sisk CL. Adolescent development of neuron structure in dentate gyrus granule cells of male Syrian hamsters. Dev Neurobiol . 2008;68(14):1517-1526. doi:10.1002/dneu.20675.
- Cunningham RL, Claiborne BJ, McGinnis MY. Pubertal exposure to anabolic androgenic steroids increases spine densities on neurons in the limbic system of male rats. Neuroscience . 2007;150(3):609-615. doi:10.1016/j.neuroscience.2007.09.038.
- Hildebrandt T, Langenbucher JW, Flores A, Harty S, Berlin HA, Berlin H. The influence of age of onset and acute anabolic steroid exposure on cognitive performance, impulsivity, and aggression in men. Psychol Addict Behav J Soc Psychol Addict Behav . 2014;28(4):1096-1104. doi:10.1037/a0036482.
- Olivares EL, Silveira ALB, Fonseca FV, et al. Administration of an anabolic steroid during the adolescent phase changes the behavior, cardiac autonomic balance and fluid intake in male adult rats. Physiol Behav . 2014;126:15-24. doi:10.1016/j.physbeh.2013.12.006.
- Grimes JM, Melloni RH. Prolonged alterations in the serotonin neural system following the cessation of adolescent anabolic-androgenic steroid exposure in hamsters (Mesocricetus auratus). Behav Neurosci . 2006;120(6):1242-1251. doi:10.1037/0735-7044.120.6.1242.
- Ricci LA, Rasakham K, Grimes JM, Melloni RH. Serotonin-1A receptor activity and expression modulate adolescent anabolic/androgenic steroid-induced aggression in hamsters. Pharmacol Biochem Behav . 2006;85(1):1-11. doi:10.1016/j.pbb.2006.06.022.
- Menard CS, Harlan RE. Up-regulation of androgen receptor immunoreactivity in the rat brain by androgenic-anabolic steroids. Brain Res . 1993;622(1-2):226-236.
- Matsumoto T, Sakari M, Okada M, et al. The androgen receptor in health and disease. Annu Rev Physiol . 2013;75:201-224. doi:10.1146/annurev-physiol-030212-183656.
- Vicencio JM, Estrada M, Galvis D, et al. Anabolic androgenic steroids and intracellular calcium signaling: a mini review on mechanisms and physiological implications. Mini Rev Med Chem . 2011;11(5):390-398.
- Yang P, Jones BL, Henderson LP. Mechanisms of anabolic androgenic steroid modulation of alpha(1)beta(3)gamma(2L) GABA(A) receptors. Neuropharmacology . 2002;43(4):619-633.
- Yang P, Jones BL, Henderson LP. Role of the alpha subunit in the modulation of GABA(A) receptors by anabolic androgenic steroids. Neuropharmacology . 2005;49(3):300-316. doi:10.1016/j.neuropharm.2005.03.017.
- Kindlundh AM, Lindblom J, Bergström L, Wikberg JE, Nyberg F. The anabolic-androgenic steroid nandrolone decanoate affects the density of dopamine receptors in the male rat brain. Eur J Neurosci . 2001;13(2):291-296.
- Thiblin I, Finn A, Ross SB, Stenfors C. Increased dopaminergic and 5-hydroxytryptaminergic activities in male rat brain following long-term treatment with anabolic androgenic steroids. Br J Pharmacol . 1999;126(6):1301-1306. doi:10.1038/sj.bjp.0702412.
- Zotti M, Tucci P, Colaianna M, et al. Chronic nandrolone administration induces dysfunction of the reward pathway in rats. Steroids . 2014;79:7-13.
- Kanayama G, Brower KJ, Wood RI, Hudson JI, Pope HG. Issues for DSM-V: clarifying the diagnostic criteria for anabolic-androgenic steroid dependence. Am J Psychiatry . 2009;166(6):642-645. doi:10.1176/appi.ajp.2009.08111699.
- Brower KJ, Blow FC, Young JP, Hill EM. Symptoms and correlates of anabolic-androgenic steroid dependence. Br J Addict . 1991;86(6):759-768.
- Malone DA, Dimeff RJ, Lombardo JA, Sample RH. Psychiatric effects and psychoactive substance use in anabolic-androgenic steroid users. Clin J Sport Med Off J Can Acad Sport Med . 1995;5(1):25-31.
- 2015 Anti-Doping Rule Violations (ADRVs) Report .; 2017. https://www.wada-ama.org/sites/default/files/resources/files/2015_adrvs_report_web_release_0.pdf .
- Compliance Monitoring. https://www.wada-ama.org/en/what-we-do/compliance-monitoring/compliance-monitoring-program . Published 2017. Accessed May 18, 2017.
- Geyer H, Schänzer W, Thevis M. Anabolic agents: recent strategies for their detection and protection from inadvertent doping. Br J Sports Med . 2014;48(10):820-826. doi:10.1136/bjsports-2014-093526.
- Catlin DH, Sekera MH, Ahrens BD, Starcevic B, Chang Y-C, Hatton CK. Tetrahydrogestrinone: discovery, synthesis, and detection in urine. Rapid Commun Mass Spectrom RCM . 2004;18(12):1245-1049. doi:10.1002/rcm.1495.
- Catlin DH, Ahrens BD, Kucherova Y. Detection of norbolethone, an anabolic steroid never marketed, in athletes’ urine. Rapid Commun Mass Spectrom RCM . 2002;16(13):1273-1275. doi:10.1002/rcm.722.
- Sekera MH, Ahrens BD, Chang Y-C, Starcevic B, Georgakopoulos C, Catlin DH. Another designer steroid: discovery, synthesis, and detection of “madol” in urine. Rapid Commun Mass Spectrom RCM . 2005;19(6):781-784. doi:10.1002/rcm.1858.
- Mareck U, Geyer H, Opfermann G, Thevis M, Schänzer W. Factors influencing the steroid profile in doping control analysis. J Mass Spectrom JMS . 2008;43(7):877-891. doi:10.1002/jms.1457.
- Yonamine M, Garcia PR, de Moraes Moreau RL. Non-intentional doping in sports. Sports Med Auckl NZ . 2004;34(11):697-704.
- Jung J, Hermanns-Clausen M, Weinmann W. Anorectic sibutramine detected in a Chinese herbal drug for weight loss. Forensic Sci Int . 2006;161(2-3):221-222. doi:10.1016/j.forsciint.2006.02.052.
- Parr MK, Koehler K, Geyer H, Guddat S, Schänzer W. Clenbuterol marketed as dietary supplement. Biomed Chromatogr BMC . 2008;22(3):298-300. doi:10.1002/bmc.928.
- Geyer H, Braun H, Burke LM, Stear SJ, Castell LM. A-Z of nutritional supplements: dietary supplements, sports nutrition foods and ergogenic aids for health and performance--Part 22. Br J Sports Med . 2011;45(9):752-754. doi:10.1136/bjsports-2011-090180.
- De Cock KJ, Delbeke FT, Van Eenoo P, Desmet N, Roels K, De Backer P. Detection and determination of anabolic steroids in nutritional supplements. J Pharm Biomed Anal . 2001;25(5-6):843-852.
- Tainted Products Marketed as Dietary Supplements. https://www.accessdata.fda.gov/scripts/sda/sdNavigation.cfm?sd=tainted_supplements_cder . Accessed January 9, 2018.
- World Anti-Doping Code. 2009. https://www.wada-ama.org/sites/default/files/resources/files/wada_anti-doping_code_2009_en_0.pdf . Accessed May 23, 2017.
- Elliot D, Goldberg L. Intervention and prevention of steroid use in adolescents. Am J Sports Med . 1996;24(6 Suppl):S46-47.
- Goldberg L, Bents R, Bosworth E, Trevisan L, Elliot DL. Anabolic steroid education and adolescents: do scare tactics work? Pediatrics . 1991;87(3):283-286.
- Middleman AB, Faulkner AH, Woods ER, Emans SJ, DuRant RH. High-risk behaviors among high school students in Massachusetts who use anabolic steroids. Pediatrics . 1995;96(2 Pt 1):268-272.
- Goldberg L, MacKinnon DP, Elliot DL, Moe EL, Clarke G, Cheong J. The adolescents training and learning to avoid steroids program: preventing drug use and promoting health behaviors. Arch Pediatr Adolesc Med . 2000;154(4):332-338.
- Elliot DL, Goldberg L, Moe EL, Defrancesco CA, Durham MB, Hix-Small H. Preventing substance use and disordered eating: initial outcomes of the ATHENA (athletes targeting healthy exercise and nutrition alternatives) program. Arch Pediatr Adolesc Med . 2004;158(11):1043-1049. doi:10.1001/archpedi.158.11.1043.
- Elliot DL, Goldberg L, Moe EL, et al. Long-term Outcomes of the ATHENA (Athletes Targeting Healthy Exercise & Nutrition Alternatives) Program for Female High School Athletes. J Alcohol Drug Educ . 2008;52(2):73-92.
- Pope HG, Kanayama G, Ionescu-Pioggia M, Hudson JI. Anabolic steroid users’ attitudes towards physicians. Addict Abingdon Engl . 2004;99(9):1189-1194. doi:10.1111/j.1360-0443.2004.00781.x.
- Brennan BP, Kanayama G, Pope HG. Performance-enhancing drugs on the web: a growing public-health issue. Am J Addict Am Acad Psychiatr Alcohol Addict . 2013;22(2):158-161. doi:10.1111/j.1521-0391.2013.00311.x.
- Kanayama G, Brower KJ, Wood RI, Hudson JI, Pope HG. Treatment of anabolic-androgenic steroid dependence: Emerging evidence and its implications. Drug Alcohol Depend . 2010;109(1-3):6-13. doi:10.1016/j.drugalcdep.2010.01.011

An official website of the United States government
The .gov means it’s official. Federal government websites often end in .gov or .mil. Before sharing sensitive information, make sure you’re on a federal government site.
The site is secure. The https:// ensures that you are connecting to the official website and that any information you provide is encrypted and transmitted securely.
- Publications
- Account settings
- My Bibliography
- Collections
- Citation manager
Save citation to file
Email citation, add to collections.
- Create a new collection
- Add to an existing collection
Add to My Bibliography
Your saved search, create a file for external citation management software, your rss feed.
- Search in PubMed
- Search in NLM Catalog
- Add to Search
Epidemiological profile of pain and non-steroid anti-inflammatory drug use in collegiate athletes in the United States
Affiliations.
- 1 Department of Physical Therapy Education, Elon University, Elon, NC, USA.
- 2 Department of Exercise Science, Mary Baldwin College, Staunton, VA, USA.
- 3 Centre for Sport, Exercise and Osteoarthritis Research Versus Arthritis, University of Oxford, Oxford, UK.
- 4 Nuffield Department of Orthopaedics, Rheumatology, and Musculoskeletal Sciences, University of Oxford, B4495, Oxford, OX3 7LD, UK.
- 5 Unit of Physiotherapy, Department of Health, Medicine and Caring Sciences (HMV), Linköping University, Linköping, Sweden.
- 6 School of Allied Health, Human Services and Sport, Latrobe University, Melbourne, Victoria, Australia.
- 7 Kinesiology Department, Queens University of Charlotte, Charlotte, NC, USA.
- 8 Centre for Sport, Exercise and Osteoarthritis Research Versus Arthritis, University of Oxford, Oxford, UK. [email protected].
- 9 Nuffield Department of Orthopaedics, Rheumatology, and Musculoskeletal Sciences, University of Oxford, B4495, Oxford, OX3 7LD, UK. [email protected].
- 10 Department of Orthopaedic Surgery, Wake Forest School of Medicine, Winston-Salem, North Carolina, USA. [email protected].
- PMID: 32814544
- PMCID: PMC7437034
- DOI: 10.1186/s12891-020-03581-y
Background: Although athletic endeavours are associated with a high amount of physical stress and injury, the prevalence of pain is underreported in the sports medicine literature with only a few studies reporting pain on collegiate athletes or exploring sex difference of pain. Impact of pain on athlete availability, training and performance can be mitigated when key epidemiological information is used to inform adequate pain management strategies. This study aims to 1) provide an epidemiological profile of self-reported pain experienced by the National Collegiate Athletic Association (NCAA) athletes by sex during the first half of the 2019 season, 2) describe their self-reported non-steroidal anti-inflammatory drug (NSAID) use.
Methods: Online survey was completed by athletes at three NCAA institutions from 1 August to 30 September 2019. Descriptive statistics were used to describe player demographic data, self-reported pain and self-reported NSAID use. Pain incidence proportion were calculated.
Results: Two hundred thirty female athletes and 83 male athletes completed the survey. Self-reported pain incidence proportion for female athletes was 45.0 (95% CI 41.5-48.5) vs 34.9 (95% CI 29.4-40.4) for male athletes. Majority of the athletes did not report pain (55% female vs 62% male) during the first half of the 2019 season. Female athletes reported pain in their back (35%), knee (26%), and ankle/foot (23%) whilst male athletes reported pain in their knee (35%), back (28%), and shoulder (24%). Of all athletes, 28% female vs 20% male athletes reported currently taking NSAIDs. Of athletes that reported pain, 46% female vs 38% male athletes currently took NSAIDs. 70% female vs 61% male athletes self-purchased NSAIDs, and 40% female vs 55% male athletes consumed alcohol.
Conclusions: Half of female athletes and one in three male athletes reported pain. Most commonly back, knee and foot/ankle pain and knee, back and shoulder pain was reported in female and male athletes respectively. One in four female athletes and one in five male athletes use NSAIDs for pain or prophylactic purpose. Majority self-purchase these medications indicating need for health literacy interventions to mitigate potential adverse effects.
Keywords: Athlete health; Elite sport; Female athletes; Health literacy; Injury prevention; Sleep; Sports medicine.
PubMed Disclaimer
Conflict of interest statement
All authors affirm that they have no involvement with any commercial organization that has a direct financial interest in any matter included in this manuscript.
Study Participant Flow Chart
Anatomical location of the self-reported…
Anatomical location of the self-reported joint pain in female and male collegiate athletes…
Similar articles
- The Epidemiology of Stress Fractures in Collegiate Student-Athletes, 2004-2005 Through 2013-2014 Academic Years. Rizzone KH, Ackerman KE, Roos KG, Dompier TP, Kerr ZY. Rizzone KH, et al. J Athl Train. 2017 Oct;52(10):966-975. doi: 10.4085/1062-6050-52.8.01. Epub 2017 Sep 22. J Athl Train. 2017. PMID: 28937802 Free PMC article.
- The Epidemiology of Severe Injuries Sustained by National Collegiate Athletic Association Student-Athletes, 2009-2010 Through 2014-2015. Kay MC, Register-Mihalik JK, Gray AD, Djoko A, Dompier TP, Kerr ZY. Kay MC, et al. J Athl Train. 2017 Feb;52(2):117-128. doi: 10.4085/1062-6050-52.1.01. Epub 2017 Jan 24. J Athl Train. 2017. PMID: 28118030 Free PMC article.
- The Epidemiology of High Ankle Sprains in National Collegiate Athletic Association Sports. Mauntel TC, Wikstrom EA, Roos KG, Djoko A, Dompier TP, Kerr ZY. Mauntel TC, et al. Am J Sports Med. 2017 Jul;45(9):2156-2163. doi: 10.1177/0363546517701428. Epub 2017 Apr 19. Am J Sports Med. 2017. PMID: 28423285
- Injectable Nonsteroidal Anti-Inflammatory Drugs in Sport. Matava MJ. Matava MJ. Clin J Sport Med. 2018 Sep;28(5):443-450. doi: 10.1097/JSM.0000000000000602. Clin J Sport Med. 2018. PMID: 29771750 Review.
- Use of prescription drugs in athletes. Alaranta A, Alaranta H, Helenius I. Alaranta A, et al. Sports Med. 2008;38(6):449-63. doi: 10.2165/00007256-200838060-00002. Sports Med. 2008. PMID: 18489193 Review.
- Painkiller intake and problematic health literacy in sport and music students - A cross-sectional study. Pöppel K, Kreutz G, Müller M, Büsch D. Pöppel K, et al. Sci Rep. 2024 May 31;14(1):12517. doi: 10.1038/s41598-024-63127-3. Sci Rep. 2024. PMID: 38822035 Free PMC article.
- The dose-response effects of flurbiprofen, indomethacin, ibuprofen, and naproxen on primary skeletal muscle cells. Roberts BM, Geddis AV, Matheny RW Jr. Roberts BM, et al. J Int Soc Sports Nutr. 2024 Dec;21(1):2302046. doi: 10.1080/15502783.2024.2302046. Epub 2024 Jan 10. J Int Soc Sports Nutr. 2024. PMID: 38198469 Free PMC article.
- Uses of Health Care System Medical Care Services by Athletes After Injury at the High School Level. Bullock GS, Mobley JF, Brooks JM, Rauh MJ, Gil Gilliland R, Kissenberth MJ, Shanley E. Bullock GS, et al. J Sch Health. 2023 Jan;93(1):5-13. doi: 10.1111/josh.13255. Epub 2022 Oct 20. J Sch Health. 2023. PMID: 36263850 Free PMC article.
- Effect of Quercetin on Injury to Indomethacin-Treated Human Embryonic Kidney 293 Cells. Chen C, Yang JS, Lu CC, Wu YT, Chen FA. Chen C, et al. Life (Basel). 2021 Oct 25;11(11):1134. doi: 10.3390/life11111134. Life (Basel). 2021. PMID: 34833010 Free PMC article.
- National Collegiate Athletic Association. Our three divisions. http://www.ncaa.org/about/resources/media-center/ncaa-101/our-three-divi... . Published 2019. Accessed 1 Feb 2020.
- Nestel D. Athletic scholarships: An imbalance of power between the university and the student-athlete. Ohio St LJ. 1992;53:1401.
- Warner DC, Schnepf G, Barrett MS, Dian D, Swigonski NL. Prevalence, attitudes, and behaviors related to the use of nonsteroidal anti-inflammatory drugs (NSAIDs) in student athletes. J Adolesc Health. 2002;30(3):150–153. - PubMed
- Clarsen B, Myklebust G, Bahr R. Development and validation of a new method for the registration of overuse injuries in sports injury epidemiology: the Oslo sports trauma research Centre (OSTRC) overuse injury questionnaire. Br J Sports Med. 2013;47(8):495–502. - PubMed
- Da Silva ER, De Rose EH, Ribeiro JP, et al. Non-steroidal anti-inflammatory use in the XV pan-American games (2007) Br J Sports Med. 2011;45(2):91–94. - PubMed
- Search in MeSH
Related information
Grants and funding.
- HFR02510/Centre for Sport, Exercise and Osteoarthritis Research Versus Arthritis
LinkOut - more resources
Full text sources.
- BioMed Central
- Europe PubMed Central
- Linkoping University Electronic Press
- PubMed Central
- MedlinePlus Health Information
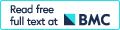
- Citation Manager
NCBI Literature Resources
MeSH PMC Bookshelf Disclaimer
The PubMed wordmark and PubMed logo are registered trademarks of the U.S. Department of Health and Human Services (HHS). Unauthorized use of these marks is strictly prohibited.

An official website of the United States government
The .gov means it’s official. Federal government websites often end in .gov or .mil. Before sharing sensitive information, make sure you’re on a federal government site.
The site is secure. The https:// ensures that you are connecting to the official website and that any information you provide is encrypted and transmitted securely.
- Publications
- Account settings
The PMC website is updating on October 15, 2024. Learn More or Try it out now .
- Advanced Search
- Journal List
- Front Sports Act Living

Stress in Academic and Athletic Performance in Collegiate Athletes: A Narrative Review of Sources and Monitoring Strategies
Marcel lopes dos santos.
1 School of Kinesiology, Applied Health and Recreation, Oklahoma State University, Stillwater, OK, United States
Melissa Uftring
Cody a. stahl, robert g. lockie.
2 Department of Kinesiology, California State University, Fullerton, CA, United States
Brent Alvar
3 Department of Kinesiology, Point Loma Nazarene University, San Diego, CA, United States
J. Bryan Mann
4 Department of Kinesiology and Sport Sciences, University of Miami, Miami, FL, United States
J. Jay Dawes
College students are required to manage a variety of stressors related to academic, social, and financial commitments. In addition to the burdens facing most college students, collegiate athletes must devote a substantial amount of time to improving their sporting abilities. The strength and conditioning professional sees the athlete on nearly a daily basis and is able to recognize the changes in performance and behavior an athlete may exhibit as a result of these stressors. As such, the strength and conditioning professional may serve an integral role in the monitoring of these stressors and may be able to alter training programs to improve both performance and wellness. The purpose of this paper is to discuss stressors experienced by collegiate athletes, developing an early detection system through monitoring techniques that identify the detrimental effects of stress, and discuss appropriate stress management strategies for this population.
Introduction
The college years are a period of time when young adults experience a significant amount of change and a variety of novel challenges. Academic performance, social demands, adjusting to life away from home, and financial challenges are just a few of the burdens college students must confront (Humphrey et al., 2000 ; Paule and Gilson, 2010 ; Aquilina, 2013 ). In addition to these stressors, collegiate athletes are required to spend a substantial amount of time participating in activities related to their sport, such as attending practices and training sessions, team meetings, travel, and competitions (Humphrey et al., 2000 ; López de Subijana et al., 2015 ; Davis et al., 2019 ; Hyatt and Kavazis, 2019 ). These commitments, in addition to the normal stress associated with college life, may increase a collegiate-athlete's risk of experiencing both physical and mental issues (Li et al., 2017 ; Moreland et al., 2018 ) that may affect their overall health and wellness. For these reasons, it is essential that coaches understand the types of stressors collegiate athletes face in order to help them manage the potentially deleterious effects stress may have on athletic and academic performance.
Strength and conditioning coaches are allied health care professionals whose primary job is to enhance fitness of individuals for the purpose of improving athletic performance (Massey et al., 2002 , 2004 , 2009 ). As such, many universities and colleges hire strength and conditioning coaches as part of their athletic staff to help athletes maximize their physical potential (Massey et al., 2002 , 2004 , 2009 ). Strength and conditioning coaches strive to increase athletic performance by the systematic application of physical stress to the body via resistance training, and other forms of exercise, to yield a positive adaptation response (Massey et al., 2002 , 2004 , 2009 ). For this reason, they need to understand and to learn how to manage athletes' stress. Additionally, based on the cumulative nature of stress, it is important that both mental and emotional stressors are also considered in programming. It is imperative that strength and conditioning coaches are aware of the multitude of stressors collegiate athletes encounter, in order to incorporate illness and injury risk management education into their training programs (Radcliffe et al., 2015 ; Ivarsson et al., 2017 ).
Based on the large number of contact hours strength and conditioning coaches spend with their athletes, they are in an optimal position to assist athletes with developing effective coping strategies to manage stress. By doing so, strength and conditioning coaches may be able to help reach the overarching goal of improving the health, wellness, fitness, and performance of the athletes they coach. The purpose of this review article is to provide the strength and conditioning professional with a foundational understanding of the types of stressors collegiate athletes may experience, and how these stressors may impact mental health and athletic performance. Suggestions for assisting athletes with developing effective coping strategies to reduce potential physiological and psychological impacts of stress will also be provided.
Stress and the Stress Response
In its most simplistic definition, stress can be described as a state of physical and psychological activation in response to external demands that exceed one's ability to cope and requires a person to adapt or change behavior. As such, both cognitive or environmental events that trigger stress are called stressors (Statler and DuBois, 2016 ). Stressors can be acute or chronic based on the duration of activation. Acute stressors may be defined as a stressful situation that occurs suddenly and results in physiological arousal (e.g., increase in hormonal levels, blood flow, cardiac output, blood sugar levels, pupil and airway dilation, etc.) (Selye, 1976 ). Once the situation is normalized, a cascade of hormonal reactions occurs to help the body return to a resting state (i.e., homeostasis). However, when acute stressors become chronic in nature, they may increase an individual's risk of developing anxiety, depression, or metabolic disorders (Selye, 1976 ). Moreover, the literature has shown that cumulative stress is correlated with an increased susceptibility to illness and injury (Szivak and Kraemer, 2015 ; Mann et al., 2016 ; Hamlin et al., 2019 ). The impact of stress is individualistic and subjective by nature (Williams and Andersen, 1998 ; Ivarsson et al., 2017 ). Additionally, the manner in which athletes respond to a situational or environmental stressor is often determined by their individual perception of the event (Gould and Udry, 1994 ; Williams and Andersen, 1998 ; Ivarsson et al., 2017 ). In this regard, the athlete's perception can either be positive (eustress) or negative (distress). Even though they both cause physiological arousal, eustress also generates positive mental energy whereas distress generates anxiety (Statler and DuBois, 2016 ). Therefore, it is essential that an athlete has the tools and ability to cope with these stressors in order to have the capacity to manage both acute and chronic stress. As such, it is important to understand the types of stressors collegiate athletes are confronted with and how these stressors impact an athlete's performance, both athletically and academically.
Literature Search/Data Collection
The articles included in this review were identified via online databases PubMed, MEDLINE, and ISI Web of Knowledge from October 15th 2019 through January 15th 2020. The search strategy combined the keywords “academic stress,” “athletic stress,” “stress,” “stressor,” “college athletes,” “student athletes,” “collegiate athletes,” “injury,” “training,” “monitoring.” Duplicated articles were then removed. After reading the titles and abstracts, all articles that met the inclusion criteria were considered eligible for inclusion in the review. Subsequently, all eligible articles were read in their entirety and were either included or removed from the present review.
Inclusion Criteria
The studies included met all the following criteria: (i) published in English-language journals; (ii) targeted college athletes; (iii) publication was either an original research paper or a literature review; (iv) allowed the extraction of data for analysis.
Data Analysis
Relevant data regarding participant characteristics (i.e., gender, academic status, sports) and study characteristics were extracted. Articles were analyzed and divided into two separate sections based on their specific topics: Academic Stress and Athletic Stress. Then, strategies for monitoring and workload management are discussed in the final section.
Academic Stress
Fundamentally, collegiate athletes have two major roles they must balance as part of their commitment to a university: being a college student and an athlete. Academic performance is a significant source of stress for most college students (Aquilina, 2013 ; López de Subijana et al., 2015 ; de Brandt et al., 2018 ; Davis et al., 2019 ). This stress may be further compounded among collegiate athletes based on their need to be successful in the classroom, while simultaneously excelling in their respective sport (Aquilina, 2013 ; López de Subijana et al., 2015 ; Huml et al., 2016 ; Hamlin et al., 2019 ). Davis et al. ( 2019 ) conducted surveys on 173 elite junior alpine skiers and reported significant moderate to strong correlations between perceived stress and several variables including depressed mood ( r = 0.591), sleep disturbance ( r = 0.459), fatigue ( r = 0.457), performance demands ( r = 0.523), and goals and development ( r = 0.544). Academic requirements were the highest scoring source of stress of all variables and was most strongly correlated with perceived stress ( r = 0.467). Interestingly, it was not academic rigor that was viewed by the athletes as the largest source of direct stress; rather, the athletes surveyed reported time management as being their biggest challenge related to academic performance (Davis et al., 2019 ). This further corroborates the findings of Hamlin et al. ( 2019 ). The investigators reported that during periods of the academic year in which levels of perceived academic stress were at their highest, students had trouble managing sport practices and studying. These stressors were also associated with a decrease in energy levels and overall sleep quality. These factors may significantly increase the collegiate athlete's susceptibility to illness and injury (Hamlin et al., 2019 ). For this reason, coaches should be aware of and sensitive to the stressors athletes experience as part of the cyclical nature of the academic year and attempt to help athletes find solutions to balancing athletic and academic demands.
According to Aquilina ( 2013 ), collegiate athletes tend to be more committed to sports development and may view their academic career as a contingency plan to their athletic career, rather than a source of personal development. As a result, collegiate athletes often, but certainly not always, prioritize athletic participation over their academic responsibilities (Miller and Kerr, 2002 ; Cosh and Tully, 2014 , 2015 ). Nonetheless, scholarships are usually predicated on both athletic and academic performance. For instance, the National Collegiate Athletic Association (NCAA) requires collegiate athletes to achieve and maintain a certain grade point average (GPA). Furthermore, they are also often required to also uphold a certain GPA to maintain an athletic scholarship. The pressure to maintain both high levels of academic and athletic performance may increase the likelihood of triggering mental health issues (i.e., anxiety and depression) (Li et al., 2017 ; Moreland et al., 2018 ).
Mental health issues are a significant concern among college students. There has been an increased emphasis placed on the mental health of collegiate athletes in recent years (Petrie et al., 2014 ; Li et al., 2017 , 2019 ; Reardon et al., 2019 ). Based on the 2019 National College Health Assessment survey from the American College Health Association (ACHA) consisting of 67,972 participants, 27.8% of college students reported anxiety, and 20.2% reported experiencing depression which negatively affected their academic performance (American College Health Association American College Health Association-National College Health Assessment II, 2019 ). Approximately 65.7% (50.7% males and 71.8% females) reported feeling overwhelming anxiety in the past 12 months, and 45.1% (37.1% males and 47.6% females) reported feeling so depressed that it was difficult for them to function. However, only 24.3% (13% males and 28.4% females) reported being diagnosed and treated by a professional in the past 12 months. Collegiate athletes are not immune to these types of issues. According to information presented by the NCAA, many certified athletic trainers anecdotally state that anxiety is an issue affecting the collegiate-athlete population (NCAA, 2014 ). However, despite the fact that collegiate athletes are exposed to numerous stressors, they are less likely to seek help at a university counseling center than non-athletes (NCAA, 2014 ), which could be related to stigmas that surround mental health services (NCAA, 2014 ; Kaier et al., 2015 ; Egan, 2019 ). This not only has significant implications related to their psychological well-being, but also their physiological health, and consequently their performance. For instance, in a study by Li et al. ( 2017 ) it was found that NCAA Division I athletes who reported preseason anxiety symptoms had a 2.3 times greater injury incidence rate compared to athletes who did not report. This same study discovered that male athletes who reported preseason anxiety and depression had a 2.1 times greater injury incidence, compared to male athletes who did not report symptoms of anxiety and depression. (Lavallée and Flint, 1996 ) also reported a correlation between anxiety and both injury frequency and severity among college football players ( r = 0.43 and r = 0.44, respectively). In their study, athletes reporting high tension/anxiety had a higher rate of injury. It has been suggested that the occurrence of stress and anxiety may cause physiological responses, such as an increase in muscle tension, physical fatigue, and a decrease in neurocognitive and perception processes that can lead to physical injuries (Ivarsson et al., 2017 ). For this reason, it is reasonable to consider that academic stressors may potentiate effects of stress and result in injury and illness in collegiate athletes.
Periods of more intense academic stress increase the susceptibility to illness or injury (Mann et al., 2016 ; Hamlin et al., 2019 ; Li et al., 2019 ). For example, Hamlin et al. ( 2019 ) investigated levels of perceived stress, training loads, injury, and illness incidence in 182 collegiate athletes for the period of one academic year. The highest levels of stress and incidence of illness arise during the examination weeks occurring within the competitive season. In addition, the authors also reported the odds ratio, which is the occurrence of the outcome of interest (i.e., injury), based off the given exposure to the variables of interest (i.e., perceived mood, sleep duration, increased academic stress, and energy levels). Based on a logistic regression, they found that each of the four variables (i.e., mood, energy, sleep duration, and academic stress) was related to the collegiate athletes' likelihood to incur injuries. In summary, decreased levels of perceived mood (odds ratio of 0.89, 0.85–0.0.94 CI) and sleep duration (odds ratio of 0.94, 0.91–0.97 CI), and increased academic stress (odds ratio of 0.91, 0.88–0.94 CI) and energy levels (odds ratio of 1.07, 1.01–1.14 CI), were able to predict injury in these athletes. This corroborates Mann et al. ( 2016 ) who found NCAA Division I football athletes at a Bowl Championship Subdivision university were more likely to become ill or injured during an academically stressful period (i.e., midterm exams or other common test weeks) than during a non-testing week (odds ratio of 1.78 for high academic stress). The athletes were also less likely to get injured during training camp (odds ratio of 3.65 for training camp). Freshmen collegiate athletes may be especially more susceptible to mental health issues than older students. Their transition includes not only the academic environment with its requirements and expectations, but also the adaptation to working with a new coach and teammates. In this regard, Yang et al. ( 2007 ) found an increase in the likelihood of depression that freshmen athletes experienced, as these freshmen were 3.27 times more likely to experience depression than their older teammates. While some stressors are recurrent and inherent in academic life (e.g., attending classes, homework, etc.), others are more situational (e.g., exams, midterms, projects) and may be anticipated by the strength and conditioning coach.
Athletic Stress
The domain of athletics can expose collegiate athletes to additional stressors that are specific to their cohort (e.g., sport-specific, team vs. individual sport) (Aquilina, 2013 ). Time spent training (e.g., physical conditioning and sports practice), competition schedules (e.g., travel time, missing class), dealing with injuries (e.g., physical therapy/rehabilitation, etc.), sport-specific social support (e.g., teammates, coaches) and playing status (e.g., starting, non-starter, being benched, etc.) are just a few of the additional challenges collegiate athletes must confront relative to their dual role of being a student and an athlete (Maloney and McCormick, 1993 ; Scott et al., 2008 ; Etzel, 2009 ; Fogaca, 2019 ). Collegiate athletes who view the demands of stressors from academics and sports as a positive challenge (i.e., an individual's self-confidence or belief in oneself to accomplish the task outweighs any anxiety or emotional worry that is felt) may potentially increase learning capacity and competency (NCAA, 2014 ). However, when these demands are perceived as exceeding the athlete's capacity, this stress can be detrimental to the student's mental and physical health as well as to sport performance (Ivarsson et al., 2017 ; Li et al., 2017 ).
As previously stated, time management has been shown to be a challenge to collegiate athletes. The NCAA rules state that collegiate athletes may only engage in required athletic activities for 4 h per day and 20 h/week during in-season and 8 h/week during off-season throughout the academic year. Although these rules have been clearly outlined, the most recent NCAA GOALS (2016) study reported alarming numbers regarding time commitment to athletic-related activities. Data from over 21,000 collegiate athletes from 600 schools across Divisions I, II, and III were included in this study. Although a breakdown of time commitments was not provided, collegiate athletes reported dedicating up to 34 h per week to athletics (e.g., practices, weight training, meetings with coaches, tactical training, competitions, etc.), in addition to spending between 38.5 and 40 h per week working on academic-related tasks. This report also showed a notable trend related to athletes spending an increase of ~2 more athletics-related hours per week compared to the 2010 GOALS study, along with a decrease of 2 h of personal time (from 19.5 h per week in 2010 to 17.1 in 2015). Furthermore, ~66% of Division I and II and 50% of Division III athletes reported spending as much or more time in their practices during the off-season as during the competitive season (DTHOMAS, 2013 ). These numbers show how important it is for collegiate athletes to develop time management skills to be successful in both academics and athletics. Overall, most collegiate athletes have expressed a need to find time to enjoy their college experience outside of athletic obligations (Paule and Gilson, 2010 ). Despite that, because of the increasing demand for excellence in academics and athletics, collegiate athletes' free time with family and friends is often scarce (Paule and Gilson, 2010 ). Consequently, trainers, coaches, and teammates will likely be the primary source of their weekly social interactivity.
Social interactions within their sport have also been found to relate to factors that may impact an athlete's perceived stress. Interactions with coaches and trainers can be effective or deleterious to an athlete. Effective coaching includes a coaching style that allows for a boost of the athlete's motivation, self-esteem, and efficacy in addition to mitigating the effects of anxiety. On the other hand, poor coaching (i.e., the opposite of effective coaching) can have detrimental psychological effects on an athlete (Gearity and Murray, 2011 ). In a closer examination of the concept of poor coaching practices, Gearity and Murray ( 2011 ) interviewed athletes about their experiences of receiving poor coaching. Following analysis of the interviews, the authors identified the main themes of the “coach being uncaring and unfair,” “practicing poor teaching inhibiting athlete's mental skills,” and “athlete coping.” They stated that inhibition of an athlete's mental skills and coping are associated with the psychological well-being of an athlete. Also, poor coaching may result in mental skills inhibition, distraction, insecurity, and ultimately team division (Gearity and Murray, 2011 ). This combination of factors may compound the negative impacts of stress in athletes and might be especially important for in injured athletes.
Injured athletes have previously been reported to have elevated stress as a result of heightened worry about returning to pre-competition status (Crossman, 1997 ), isolation from teammates if the injury is over a long period of time (Podlog and Eklund, 2007 ) and/or reduced mood or depressive symptoms (Daly et al., 1995 ). In addition, athletes who experience prolonged negative thoughts may be more likely to have decreased rehabilitation attendance or adherence, worse functional outcomes from rehabilitation (e.g., on measures of proprioception, muscular endurance, and agility), and worse post-injury performance (Brewer, 2012 ).
Monitoring Considerations
In addition to poor coaching, insufficient workload management can hinder an athlete's ability to recover and adapt to training, leading to fatigue accumulation (Gabbett et al., 2017 ). Excessive fatigue can impair decision-making ability, coordination and neuromuscular control, and ultimately result in overtraining and injury (Soligard et al., 2016 ). For instance, central fatigue was found to be a direct contributor to anterior cruciate ligament injuries in soccer players (Mclean and Samorezov, 2009 ). Introducing monitoring tools may serve as a means to reduce the detrimental effects of stress in collegiate athletes. Recent research on relationships between athlete workloads, injury, and performance has highlighted the benefits of athlete monitoring (Drew and Finch, 2016 ; Jaspers et al., 2017 ).
Athlete monitoring is often assessed with the measuring and management of workload associated with a combination of sport-related and non-sport-related stressors (Soligard et al., 2016 ). An effective workload management program should aim to detect excessive fatigue, identify its causes, and constantly adapt rest, recovery, training, and competition loads respectively (Soligard et al., 2016 ). The workload for each athlete is based off their current levels of physical and psychological fatigue, wellness, fitness, health, and recovery (Soligard et al., 2016 ). Accumulation of situational or physical stressors will likely result in day-to-day fluctuations in the ability to move external loads and strength train effectively (Fry and Kraemer, 1997 ). Periods of increased academic stress may cause increased levels of fatigue, which can be identified by using these monitoring tools, thereby assisting the coaches with modulating the workload during these specific periods. Coaches who plan to incorporate monitoring and management strategies must have a clear understanding of what they want to achieve from athlete monitoring (Gabbett et al., 2017 ; Thornton et al., 2019 ).
Monitoring External Loads
External load refers to the physical work (e.g., number of sprints, weight lifted, distance traveled, etc.) completed by the athlete during competition, training, and activities of daily living (Soligard et al., 2016 ). This type of load is independent of the athlete's individual characteristics (Wallace et al., 2009 ). Monitoring external loading can aid in the designing of training programs which mimic the external load demands of an athlete's sport, guide rehabilitation programs, and aid in the detection of spikes in external load that may increase the risk of injury (Clubb and McGuigan, 2018 ).
The means of quantifying external load can involve metrics as simple as pitch counts in baseball and softball (Fleisig and Andrews, 2012 ; Shanley et al., 2012 ) or quantifying lifting session training loads (e.g., sum value of weight lifted during an exercise x number of repetitions × the number of sets). Neuromuscular function testing is another more common way of analyzing external load. This is typically done using such measures such as the counter movement jump, squat jump, or drop jump. A force platform can be used to measure a myriad of outcomes (e.g., peak power, ground contact time, time to take-off, reactive strength index, and jump height), or simply measure jump height in a more traditional manner. Jumping protocols, such as the countermovement jump, have been adopted to examine the recovery of neuromuscular function after athletic competition with significant decreases for up to 72 h commonly reported (Andersson et al., 2008 ; Magalhães et al., 2010 ; Twist and Highton, 2013 ). (Gathercole et al., 2015 ) found reductions in 18 different neuromuscular variables in collegiate athletes following a fatiguing protocol. The variables of eccentric duration, concentric duration, total duration, time to peak force/power, and flight time:contraction time ratio, derived from a countermovement jump were deemed suitable for detecting neuromuscular fatigue with the rise in the use of technology for monitoring, certain sports have adopted specific software that can aid in the monitoring of stress. For example, power output can be measured using devices such as SRM™ or PowerTap™ in cycling (Jobson et al., 2009 ). This data can be analyzed to provide information such as average power or normalized power. The power output can then be converted into a Training Stress Score™ via commercially available software (Marino, 2011 ). More sophisticated measures of external load may involve the use of wearable technology devices such as Global Positioning System (GPS) devices, accelerometers, magnetometer, and gyroscope inertial sensors (Akenhead and Nassis, 2016 ). These devices can quantify external load in several ways, such as duration of movement, total distance covered, speed of movement, acceleration, and decelerations, as well as sport specific movement such as number and height of jumps, number of tackles, or breakaways, etc. (Akenhead and Nassis, 2016 ). The expansion of marketing of wearable devices has been substantial; however, there are questions of validity and reliability related to external load tracking limitations related to proprietary metrics, as well as the overall cost that should be considered when considering the adoption of such devices (Aughey et al., 2016 ; Torres-Ronda and Schelling, 2017 ).
Monitoring Internal Loads
While external load may provide information about an athlete's performance capacity and work completed, it does not provide clear evidence of how athletes are coping with and adapting to the external load (Halson, 2014 ). This type of information comes from the monitoring of internal loads. The term internal load refers to the individual physiological and psychological response to the external stress or load imposed (Wallace et al., 2009 ). Internal load is influenced by a number of factors such as daily life stressors, the environment around the athlete, and coping ability (Soligard et al., 2016 ). Indirect measures, such as the use of heart rate (HR) monitoring, and subjective measurements, such as perceived effort (i.e., ratings of perceived exertion), are examples of internal load monitoring. Using subjective measurement systems is a simple and practical method when dealing with large numbers of athletes (Saw et al., 2016 ; Nässi et al., 2017 ). Subjective reporting of training load (Rating of Perceived Exertion—RPE) (Coyne et al., 2018 ), Session Rating of Perceived Exertion—sRPE) (Coyne et al., 2018 ), perceived stress and recovery (Recovery Stress Questionnaire for Athletes—RESTQ-S), and psychological mood states (Profile of Mood States—POMS) have all been found to be a reliable indicator of training load (Robson-Ansley et al., 2009 ; Saw et al., 2016 ) and only take a few moments to complete. In addition, subjective measures can be more responsive to tracking changes or training responses in athletes than objective measures (Saw et al., 2016 ).
Heart rate (HR) monitoring is a common intrinsic measure of how the body is responding to stress. With training, the reduction of resting HR is typically a clear indication of the heart becoming more efficient and not having to beat as frequently. Alternately, increases of resting HR over time with a continuation of training may be an indicator of too much stress. Improper nutrition, such as regular or ongoing suboptimal intakes of vitamins or minerals, may result in increased ventilation and/or increased heart rate (Lukaski, 2004 ). It has been suggested that the additional stress may lead to parasympathetic hyperactivity, leading to an increase in resting HR (Statler and DuBois, 2016 ). This largely stems from research examining the sensitivity of various HR derived metrics, such as resting HR, HR variability (HRV), and HR recovery (HRR) to fluctuations in training load (Borresen and Ian Lambert, 2009 ). HRR in athlete monitoring is the rate of HR decline after the cessation of exercise. A common measure of HHR is the use of a 2 min step test followed by a 60 s HR measurement. The combination of the exercise (stress) on the cardiovascular system and then its subsequent return toward baseline has been used as an indicator of autonomic function and training status in athletes (Daanen et al., 2012 ). In collegiate athletes it was found that hydration status impacted HRR following moderate to hard straining sessions (Ayotte and Corcoran, 2018 ). Athletes who followed a prescription hydration plan performed better in the standing long jump, tracked objects faster, and showed faster HRR vs. athletes who followed their normal self-selected hydration plan (Ayotte and Corcoran, 2018 ). To date, HR monitoring and the various derivatives have mainly been successful in detecting changes in training load and performance in endurance athletes (Borresen and Ian Lambert, 2009 ; Lamberts et al., 2009 ; Thorpe et al., 2017 ). Although heart rate monitoring can provide additional physiological insight for aerobic sessions or events, it thus far has not been found to be an accurate measurement for quantifying internal load during many explosive, short duration anaerobic activities (Bosquet et al., 2008 ).
A multitude of studies have reported the reliability and validity of using RPE and sRPE across a range of training modalities (Foster, 1998 ; Impellizzeri et al., 2004 ; Sweet et al., 2004 ). This measure can be used to create a number of metrics such as session load (sRPE × duration in minutes), daily load (sum of all session loads for that day), weekly training load (sum of all daily training loads for entire week), monotony (standard deviation of weekly training load), and strain (daily or weekly training load × monotony) (Foster, 1998 ). Qualitative questionnaires that monitor stress and fatigue have been well-established as tools to use with athletes (see Table 1 for examples of commonly used questionnaires in research). Using short daily wellness questionnaires may allow coaches to generate a wellness score which then can be adjusted based off of the stress the athlete may be feeling to meet the daily load target (Foster, 1998 ; Robson-Ansley et al., 2009 ). However, strength and conditioning coaches need to be mindful that these questionnaires may require sports psychologist or other licensed professional to examine and provide the results. An alternative that may be better suited for strength and conditioning professionals to use could be to incorporate some of the themes of those questionnaires into programing.
Overview of common tool/measures used by researchers to monitor training load.
: defined as the work completed by the athlete, measured independently of their individual characteristics (Wallace et al., ) | Power output | Various devices | Pyne and Martin, |
Neuromuscular function | Jump tests—CMJ or SJ performance | Twist and Highton, | |
Sprint performance | Twist and Highton, | ||
Time-motion analysis | GPS tracking | Aughey, ; Halson, | |
Movement pattern analysis via digital video | Taylor et al., ; Halson ( ) | ||
related to physiological and psychological stress imposed (Wallace et al., ) | Perception of effort | Rating of Perceived Exertion (RPE) | Borresen and Ian Lambert, |
Session Rating of Perceived Exertion (sRPE) | Foster, | ||
Heart rate measures | Heart rate (HR) | Hopkins, | |
HR to RPE | Martin and Andersen, | ||
HR recovery (HRR) | Daanen et al., | ||
HR variability (HRV) | Plews et al., | ||
Training Impulse (TRIMP) | Morton et al., ; Pyne and Martin, | ||
Qualitative questionnaires | Profile of Mood States (ROMS) | Morgan et al., | |
Recovery Stress Questionnaires for Athletes (REST-Q) | Kallus and Kellmann, | ||
Daily Analysis of Life Demands for Athletes (DALDA) | Rushall, | ||
Total Recovery Scale (TQR) | Kenttä and Hassmén, |
A Multifaceted Approach
Dissociation between external and internal load units may be indicative of the state of fatigue of an athlete. Utilizing a monitoring system in which the athlete is able to make adjustments to their training loads in accordance with how they are feeling in that moment can be a useful tool for assisting the athlete in managing stress. Auto-regulation is a method of programming that allows for adjustments based on the results of one or more readiness tests. When implemented properly, auto regulation enables the coach or athlete to optimize training based on the athlete's given readiness for training on a particular day, thereby aiming to avoid potential overtraining (Kraemer and Fleck, 2018 ). Several studies have found that using movement velocity to designate resistance training intensities can result in significant improvements in maximal strength and athletic performance (Pareja-Blanco et al., 2014 , 2017 ; Mann et al., 2015 ). Velocity based training allows the coach and athlete to view real time feedback for the given lifts, thereby allowing them to observe how the athlete is performing in that moment. If the athlete is failing to meet the prescribed velocity or the velocity drops greater than a predetermined amount between sets, then this should signal the coach to investigate. If there is a higher than normal amount of stress on that athlete for the day, that could be a potential reason. This type of combination style program of using a quantitative or objective measurement (s) and a subjective measure of wellness (qualitative questionnaire) has recently been reported to be an effective tool in monitoring individuals apart of a team (Starling et al., 2019 ). The subjective measure in this study was the readiness to train questionnaire (RTT-Q) and the objective measures were the HRR 6min test (specifically the HRR 60s = recorded as decrease in HR in the 60 s after termination of the test) to assess autonomic function and the standing long jump (SLJ) to measure neuromuscular function. The findings found that, based on the absolute typical error of measurement, the HRR 60s and SLJ could detect medium and large changes in fatigue and readiness. The test took roughly 8 min for the entire team, which included a group consisting of 24 college-age athletes. There are many other combinations of monitoring variables and strategies that coaches and athletes may utilize.
Data Analysis – How to Utilize the Measures
Regardless of what type of monitoring tool a coach or athlete may incorporate, it is essential to understand how to analyze this data. There are excellent resources available which discuss this topic in great detail (Gabbett et al., 2017 ; Clubb and McGuigan, 2018 ; Thornton et al., 2019 ). This section will highlight two main conclusions from these sources and briefly describe two of the main statistical practices and concepts discussed. The use of z-scores or modified z-scores has been proposed as a method of detecting meaningful change in athlete data (Clubb and McGuigan, 2018 ; Thornton et al., 2019 ). For different monitoring tools listed in Table 1 , the following formula would be an example of how to assess changes: (Athlete daily score—Baseline score)/Standard deviation of baseline. The baseline would likely be based off an appropriate period such as the scores across 2 weeks during the preseason.
In sports and sports science, the use of a magnitude-based inference (MBI) has been suggested as more appropriate and easier to understand when examining meaningful changes in athletic data, than null-hypothesis significance testing (NHST) (Buchheit, 2014 ). Additional methods to assess meaningful change that are similar to MBI are using standard deviation, typical error, effect sizes, smallest worthwhile change (SWC), and coefficient of variation (Thornton et al., 2019 ). It should be noted that all of these methods have faced criticism from sources such as statisticians. It is important to understand that the testing methods, measurements, and analysis should be based on the resources and intended goals from use, which will differ from every group and individual. Once identified, it is up to the practitioner to keep this system the same, in order to collect data that can then be examined to understand meaningful information for each setting (Thornton et al., 2019 ).
Managing and Coping Strategies
Once the collegiate-athlete has been able to identify the need to balance their stress levels, the athlete may then need to seek out options for managing their stress. Coaches are be able to assist them by sharing information on health and wellness resources available for the students, both on and off campus. Another way a coach can potentially support their athletes is by establishing an open-door policy, wherein the team members feel comfortable approaching a member of the strength and conditioning staff in order to seek out resources for coping with challenges related to stress.
There are some basic skills that strength and conditioning coaches can teach (while staying within their scope of practice). Coaches can introduce their athletes to basic lifestyle concepts, such as practicing deep breathing techniques, positive self-talk, and developing healthy sleep habits (i.e., turning off their mobile devices 1 h before bed and aiming for 8 h of sleep each night, etc.). A survey of strength and conditioning practitioners by Radcliffe et al. ( 2015 ) found that strategies used by practitioners included a mix of cognitive and behavioral strategies, which was used as justification for recommending practitioners find opportunities to guide professional development toward awareness strategies. Practitioners reported using a wide variety of psychological skills and strategies, which following survey analysis, highlighted a significant emphasis on strategies that may influence athlete self-confidence and goal setting. Themes identified by Radcliffe et al. ( 2015 ) included confidence building, arousal management, and skill acquisition. Additionally, similar lower level themes that are connected (i.e., goal setting, increasing, or decreasing arousal intensities, self-talk, mental imagery) are all discussed in the 4th edition of the NSCA Essentials of Strength and Conditioning book (Haff et al., 2016 ). When the interventions aiming to improve mental health expand from basic concepts to mental training beyond a coach's scope, it would be pertinent for the coach to refer the collegiate-athlete to a sport psychology or other mental health consultant (Fogaca, 2019 ). Moreover, strength and conditioning coaches may find themselves in a position to become key players in facilitating management strategies for collegiate athletes, thereby guiding the athlete in their quest to learn how to best manage the mental and physical energy levels required in the quest for overall optimal performance (Statler and DuBois, 2016 ).
Conclusion and Future Directions
This review article has summarized some of the ways that strength and conditioning professionals may be able to gain a better understanding of the types of stressors encountered by collegiate athletes, the impact these stressors may have on athletic performance, and suggestions for assisting athletes with developing effective coping strategies to reduce the potential negative physiological and psychological impacts of stress. It has been suggested that strategies learned in the context of training may have a carry-over effect into other areas such as competition. More education is needed in order for strength and conditioning professionals to gain a greater understanding of how to support their athletes with stress-management techniques and resources. Some ways to disseminate further education on stress-management tools for coaches to share with their athletes may include professional development events, such as conferences and clinics.
Author Contributions
All of the authors have contributed to the development of the manuscript both in writing and conceptual development.
Conflict of Interest
The authors declare that the research was conducted in the absence of any commercial or financial relationships that could be construed as a potential conflict of interest. The handling editor declared a past collaboration with one of the authors RL.
- Akenhead R., Nassis G. P. (2016). Training load and player monitoring in high-level football: current practice and perceptions . Int. J. Sports Physiol. Perform. 11 , 587–593. 10.1123/ijspp.2015-0331 [ PubMed ] [ CrossRef ] [ Google Scholar ]
- American College Health Association and American College Health Association-National College Health Assessment II (2019). Reference Group Executive Summary Spring 2019 . Silver Spring, MD: American College Health Association. [ Google Scholar ]
- Andersson H., Raastad T., Nilsson J., Paulsen G., Garthe I., Kadi F. (2008). Neuromuscular fatigue and recovery in Elite female soccer: effects of active recovery . Med. Sci. Sports Exerc. 40 , 372–380. 10.1249/mss.0b013e31815b8497 [ PubMed ] [ CrossRef ] [ Google Scholar ]
- Aquilina D. (2013). A study of the relationship between elite athletes' educational development and sporting performance . Int. J. Hist. Sport 30 , 374–392. 10.1080/09523367.2013.765723 [ CrossRef ] [ Google Scholar ]
- Aughey R. J. (2011). Applications of GPS technologies to field sports . Int. J. Sports Physiol. Perform. 6 , 295–310. 10.1123/ijspp.6.3.295 [ PubMed ] [ CrossRef ] [ Google Scholar ]
- Aughey R. J., Elias G. P., Esmaeili A., Lazarus B., Stewart A. M. (2016). Does the recent internal load and strain on players affect match outcome in elite Australian football? J. Sci. Med. Sport. 19 , 182–186. 10.1016/j.jsams.2015.02.005 [ PubMed ] [ CrossRef ] [ Google Scholar ]
- Ayotte D., Corcoran M. P. (2018). Individualized hydration plans improve performance outcomes for collegiate athletes engaging in in-season training . J. Int. Soc. Sports Nutr. 15 :27. 10.1186/s12970-018-0230-2 [ PMC free article ] [ PubMed ] [ CrossRef ] [ Google Scholar ]
- Borresen J., Ian Lambert M. (2009). The quantification of training load, the training response and the effect on performance . Sports Med. 39 , 779–795. 10.2165/11317780-000000000-00000 [ PubMed ] [ CrossRef ] [ Google Scholar ]
- Bosquet L., Merkari S., Arvisais D., Aubert A. E. (2008). Is heart rate a convenient tool to monitor over-reaching? a systematic review of the literature. Br. J. Sports Med. 42 , 709–714. 10.1136/bjsm.2007.042200 [ PubMed ] [ CrossRef ] [ Google Scholar ]
- Brewer B. W. (2012). Psychology of sport injury rehabilitation, in Handbook of Sport Psychology , eds G. Tenenbaum, and R. C. Eklund (Hoboken, NJ: John Wiley & Sons, Inc; ), 404–424. 10.1002/9781118270011.ch18 [ CrossRef ] [ Google Scholar ]
- Buchheit M. (2014). Monitoring training status with HR measures: do all roads lead to Rome? Front. Physiol. 5 :73. 10.3389/fphys.2014.00073 [ PMC free article ] [ PubMed ] [ CrossRef ] [ Google Scholar ]
- Clubb J., McGuigan M. (2018). Developing cost-effective, evidence-based load monitoring systems in strength and conditioning practice . Strength Cond. J. 40 , 75–81. 10.1519/SSC.0000000000000396 [ CrossRef ] [ Google Scholar ]
- Cosh S., Tully P. J. (2014). All I have to do is pass: a discursive analysis of student athletes' talk about prioritising sport to the detriment of education to overcome stressors encountered in combining elite sport and tertiary education . Psychol. Sport Exerc. 15 , 180–189. 10.1016/j.psychsport.2013.10.015 [ CrossRef ] [ Google Scholar ]
- Cosh S., Tully P. J. (2015). Stressors, coping, and support mechanisms for student athletes combining elite sport and tertiary education: implications for practice . Sport Psychol. 29 , 120–133. 10.1123/tsp.2014-0102 [ CrossRef ] [ Google Scholar ]
- Coyne J., Haff G., Coutts A., Newton R., Nimphius S. (2018). The current state of subjective training load monitoring—a practical perspective and call to action . Sports Med. 4 :58. 10.1186/s40798-018-0172-x [ PMC free article ] [ PubMed ] [ CrossRef ] [ Google Scholar ]
- Crossman J. (1997). Psychological rehabilitation from sports injuries . Sports Med. 23 , 333–339. 10.2165/00007256-199723050-00005 [ PubMed ] [ CrossRef ] [ Google Scholar ]
- Daanen H. A. M., Lamberts R. P., Kallen V. L., Jin A., van Meeteren N. L. U. (2012). A systematic review on heart-rate recovery to monitor changes in training status in athletes . Int. J. Sports Physiol. Perform. 7 , 251–260. 10.1123/ijspp.7.3.251 [ PubMed ] [ CrossRef ] [ Google Scholar ]
- Daly J. M., Brewer B. W., van Raalte J. L., Petitpas A. J., Sklar J. H. (1995). Cognitive appraisal, emotional adjustment, and adherence to rehabilitation following knee surgery . J. Sport Rehabil. 4 , 23–30. 10.1123/jsr.4.1.23 [ CrossRef ] [ Google Scholar ]
- Davis P., Halvarsson A., Lundström W., Lundqvist C. (2019). Alpine ski coaches' and athletes' perceptions of factors influencing adaptation to stress in the classroom and on the slopes . Front. Psychol. 10 :1641. 10.3389/fpsyg.2019.01641 [ PMC free article ] [ PubMed ] [ CrossRef ] [ Google Scholar ]
- de Brandt K., Wylleman P., Torregrossa M., Schipper-van Veldhoven N., Minelli D., Defruyt S., et al. (2018). Exploring the factor structure of the dual career competency questionnaire for Athletes in European pupil- and student-athletes . J. Sport. Exercise. Psy. 1–18. 10.1080/1612197X.2018.1511619 [ CrossRef ] [ Google Scholar ]
- Drew M. K., Finch C. F. (2016). The relationship between training load and injury, illness and soreness: a systematic and literature review . Sports Med. 46 , 861–883. 10.1007/s40279-015-0459-8 [ PubMed ] [ CrossRef ] [ Google Scholar ]
- DTHOMAS (2013). NCAA GOALS Study. NCAA.Org - The Official Site of the NCAA . Available online at: http://www.ncaa.org/about/resources/research/ncaa-goals-study (accessed October 5, 2019).
- Egan K. P. (2019). Supporting mental health and well-being among student-athletes . Clin. Sports Med. 38 , 537–544. 10.1016/j.csm.2019.05.003 [ PubMed ] [ CrossRef ] [ Google Scholar ]
- Etzel E. F. ed. (2009). Counseling and Psychological Services for College Student-Athletes . Morgantown, WV: Fitness Information Technology. [ Google Scholar ]
- Fleisig G. S., Andrews J. R. (2012). Prevention of elbow injuries in youth baseball pitchers . Sports Health 4 , 419–424. 10.1177/1941738112454828 [ PMC free article ] [ PubMed ] [ CrossRef ] [ Google Scholar ]
- Fogaca J. L. (2019). Combining mental health and performance interventions: coping and social support for student-athletes . J. Appl. Sport Psychol. 1–16. 10.1080/10413200.2019.1648326 [ CrossRef ] [ Google Scholar ]
- Foster C. (1998). Monitoring training in athletes with reference to overtraining syndrome . Med. Sci. Sports Exerc. 30 , 1164–1168. 10.1097/00005768-199807000-00023 [ PubMed ] [ CrossRef ] [ Google Scholar ]
- Fry A. C., Kraemer W. J. (1997). Resistance exercise overtraining and overreaching: neuroendocrine responses . Sports Med. 23 , 106–129. 10.2165/00007256-199723020-00004 [ PubMed ] [ CrossRef ] [ Google Scholar ]
- Gabbett T. J., Nassis G. P., Oetter E., Pretorius J., Johnston N., Medina D., et al.. (2017). The athlete monitoring cycle: a practical guide to interpreting and applying training monitoring data . Br. J. Sports Med. 51 , 1451–1452. 10.1136/bjsports-2016-097298 [ PubMed ] [ CrossRef ] [ Google Scholar ]
- Gathercole R. J., Sporer B. C., Stellingwerff T., Sleivert G. G. (2015). Comparison of the capacity of different jump and sprint field tests to detect neuromuscular fatigue . J. Strength Cond. Res. 29 , 2522–2531. 10.1519/JSC.0000000000000912 [ PubMed ] [ CrossRef ] [ Google Scholar ]
- Gearity B. T., Murray M. A. (2011). Athletes' experiences of the psychological effects of poor coaching . Psychol. Sport Exerc. 12 , 213–221. 10.1016/j.psychsport.2010.11.004 [ CrossRef ] [ Google Scholar ]
- Gould D., Udry E. (1994). Psychological skills for enhancing performance: arousal regulation strategies . Med. Sci. Sports Exerc. 26 , 478–485. [ PubMed ] [ Google Scholar ]
- Haff G., Triplett N. T., National Strength Conditioning Association (U.S.) eds. (2016). Essentials of Strength Training and Conditioning . 4th Edn Champaign, IL: Human Kinetics. [ Google Scholar ]
- Halson S. L. (2014). Monitoring training load to understand fatigue in athletes . Sports Med. 44 , 139–147. 10.1007/s40279-014-0253-z [ PMC free article ] [ PubMed ] [ CrossRef ] [ Google Scholar ]
- Hamlin M. J., Wilkes D., Elliot C. A., Lizamore C. A., Kathiravel Y. (2019). Monitoring training loads and perceived stress in young elite university athletes . Front. Physiol. 10 :34. 10.3389/fphys.2019.00034 [ PMC free article ] [ PubMed ] [ CrossRef ] [ Google Scholar ]
- Hopkins W. G. (1991). Quantification of training in competitive sports: methods and applications . Sports Med. 12 , 161–183. 10.2165/00007256-199112030-00003 [ PubMed ] [ CrossRef ] [ Google Scholar ]
- Huml M. R., Hambrick M. E., Hums M. A. (2016). Coaches' perceptions of the reduction of athletic commitment for division II student-athletes: development and validation of a measure of athletic/academic balance . J. Intercoll. Sport 9 , 303–325. 10.1123/jis.2015-0055 [ CrossRef ] [ Google Scholar ]
- Humphrey J. H., Yow D. A., Bowden W. W. (2000). Stress in College Athletics: Causes, Consequences, Coping . New York, NY: Haworth Half-Court Press. [ Google Scholar ]
- Hyatt H. W., Kavazis A. N. (2019). Body composition and perceived stress through a calendar year in NCAA I female volleyball players . Int. J. Exerc. Sci. 12 , 433–443. [ PMC free article ] [ PubMed ] [ Google Scholar ]
- Impellizzeri F. M., Rampinini E., Coutts A. J., Sassi A., Marcora S. M. (2004). Use of RPE-based training load in Soccer . Med. Sci. Sports Exerc. 36 , 1042–1047. 10.1249/01.MSS.0000128199.23901.2F [ PubMed ] [ CrossRef ] [ Google Scholar ]
- Ivarsson A., Johnson U., Andersen M. B., Tranaeus U., Stenling A., Lindwall M. (2017). Psychosocial factors and sport injuries: meta-analyses for prediction and prevention . Sports Med. 47 , 353–365. 10.1007/s40279-016-0578-x [ PubMed ] [ CrossRef ] [ Google Scholar ]
- Jaspers A., Brink M. S., Probst S. G. M., Frencken W. G. P., Helsen W. F. (2017). Relationships between training load indicators and training outcomes in professional Soccer . Sports Med. 47 , 533–544. 10.1007/s40279-016-0591-0 [ PubMed ] [ CrossRef ] [ Google Scholar ]
- Jobson S. A., Passfield L., Atkinson G., Barton G., Scarf P. (2009). The analysis and utilization of cycling training data . Sports Med. 39 , 833–844. 10.2165/11317840-000000000-00000 [ PubMed ] [ CrossRef ] [ Google Scholar ]
- Kaier E., Cromer L. D., Johnson M. D., Strunk K., Davis J. L. (2015). Perceptions of mental illness stigma: comparisons of athletes to nonathlete peers . J. Coll. Stud. Dev. 56 , 735–739. 10.1353/csd.2015.0079 [ CrossRef ] [ Google Scholar ]
- Kallus W., Kellmann M. (2016). The Recovery-Stress . Questionnaires: User Manual . Frankfurt: Pearson Assessment & Information GmbH. [ Google Scholar ]
- Kenttä G., Hassmén P. (1998). Overtraining and recovery: a conceptual model . Sports Med. 26 , 1–16. 10.2165/00007256-199826010-00001 [ PubMed ] [ CrossRef ] [ Google Scholar ]
- Kraemer W. J., Fleck S. J. (2018). Optimizing Strength Training: Designing Nonlinear Periodization Workouts . Champaign: Human Kinetics; Available online at: https://public.ebookcentral.proquest.com/choice/publicfullrecord.aspx?p=5730619 (accessed January 30, 2020). [ Google Scholar ]
- Lamberts R. P., Swart J., Capostagno B., Noakes T. D., Lambert M. I. (2009). Heart rate recovery as a guide to monitor fatigue and predict changes in performance parameters: heart rate recovery to monitor of performance . Scand. J. Med. Sci. Sports 20 , 449–457. 10.1111/j.1600-0838.2009.00977.x [ PubMed ] [ CrossRef ] [ Google Scholar ]
- Lavallée L., Flint F. (1996). The relationship of stress, competitive anxiety, mood state, and social support to athletic injury . J. Athl. Train. 31 , 296–299. [ PMC free article ] [ PubMed ] [ Google Scholar ]
- Li C., Ivarsson A., Lam L. T., Sun J. (2019). Basic psychological needs satisfaction and frustration, stress, and sports injury among university athletes: a four-wave prospective survey . Front. Psychol. 10 :665. 10.3389/fpsyg.2019.00665 [ PMC free article ] [ PubMed ] [ CrossRef ] [ Google Scholar ]
- Li H., Moreland J. J., Peek-Asa C., Yang J. (2017). Preseason anxiety and depressive symptoms and prospective injury risk in collegiate athletes . Am. J. Sports Med. 45 , 2148–2155. 10.1177/0363546517702847 [ PubMed ] [ CrossRef ] [ Google Scholar ]
- López de Subijana C., Barriopedro M., Conde E. (2015). Supporting dual career in Spain: Elite athletes' barriers to study . Psychol. Sport Exerc. 21 , 57–64. 10.1016/j.psychsport.2015.04.012 [ CrossRef ] [ Google Scholar ]
- Lukaski H. C. (2004). Vitamin and mineral status: effects on physical performance . Nutrition 20 , 632–644. 10.1016/j.nut.2004.04.001 [ PubMed ] [ CrossRef ] [ Google Scholar ]
- Magalhães J., Rebelo A., Oliveira E., Silva J. R., Marques F., Ascensão A. (2010). Impact of loughborough intermittent shuttle test versus soccer match on physiological, biochemical and neuromuscular parameters . Eur. J. Appl. Physiol. 108 , 39–48. 10.1007/s00421-009-1161-z [ PubMed ] [ CrossRef ] [ Google Scholar ]
- Maloney M. T., McCormick R. E. (1993). An examination of the role that intercollegiate athletic participation plays in academic achievement: athletes' feats in the classroom . J. Hum. Resour. 28 :555 10.2307/146160 [ CrossRef ] [ Google Scholar ]
- Mann J. B., Bryant K. R., Johnstone B., Ivey P. A., Sayers S. P. (2016). Effect of physical and academic stress on illness and injury in division 1 college football players . J. Strength Cond. Res. 30 , 20–25. 10.1519/JSC.0000000000001055 [ PubMed ] [ CrossRef ] [ Google Scholar ]
- Mann J. B., Ivey P. A., Sayers S. P. (2015). Velocity-based training in football . Strength Cond. J. 37 , 52–57. 10.1519/SSC.0000000000000177 [ CrossRef ] [ Google Scholar ]
- Marino F. E. (ed.). (2011). Regulation of Fatigue in Exercise . Hauppauge, NY: Nova Science Publishers. [ Google Scholar ]
- Martin D. T., Andersen M. B. (2000). Heart rate-perceived exertion relationship during training and taper . J. Sports Med. Phys. Fitness 40 , 201–208. [ PubMed ] [ Google Scholar ]
- Massey C. D., Maneval M. W., Phillips J., Vincent J., White G., Zoeller B. (2002). An analysis of teaching and coaching behaviors of elite strength and conditioning coaches . J. Strength Cond. Res. 16 , 456–460. 10.1519/00124278-200208000-00019 [ PubMed ] [ CrossRef ] [ Google Scholar ]
- Massey C. D., Schwind J. J., Andrews D. C., Maneval M. W. (2009). An analysis of the job of strength and conditioning coach for football at the division II Level . J. Strength Cond. Res. 23 , 2493–2499. 10.1519/JSC.0b013e3181bbe9b6 [ PubMed ] [ CrossRef ] [ Google Scholar ]
- Massey C. D., Vincent J., Maneval M. (2004). Job analysis of college division I-A football strength and conditioning coaches . J. Strength Cond. Res. 18 , 19–25. 10.1519/1533-4287(2004)018<0019:jaocdi>2.0.co;2 [ PubMed ] [ CrossRef ] [ Google Scholar ]
- Mclean S. G., Samorezov J. E. (2009). Fatigue-induced ACL injury risk stems from a degradation in central control . Med. Sci. Sport Exerc. 41 , 1662–1673. 10.1249/MSS.0b013e31819ca07b [ PubMed ] [ CrossRef ] [ Google Scholar ]
- Miller P. S., Kerr G. (2002). The athletic, academic and social experiences of intercollegiate student athletes . J. Sport Behav. 25 , 346–367. [ Google Scholar ]
- Moreland J. J., Coxe K. A., Yang J. (2018). Collegiate athletes' mental health services utilization: A systematic review of conceptualizations, operationalizations, facilitators, and barriers . J. Sport Health Sci. 7 , 58–69. 10.1016/j.jshs.2017.04.009 [ PMC free article ] [ PubMed ] [ CrossRef ] [ Google Scholar ]
- Morgan W. P., Brown D. R., Raglin J. S., O'Connor P. J., Ellickson K. A. (1987). Psychological monitoring of overtraining and staleness . Br. J. Sports Med. 21 , 107–114. 10.1136/bjsm.21.3.107 [ PMC free article ] [ PubMed ] [ CrossRef ] [ Google Scholar ]
- Morton R. H., Fitz-Clarke J. R., Banister E. W. (1990). Modeling human performance in running . J. Appl. Physiol. 69 , 1171–1177. 10.1152/jappl.1990.69.3.1171 [ PubMed ] [ CrossRef ] [ Google Scholar ]
- Nässi A., Ferrauti A., Meyer T., Pfeiffer M., Kellmann M. (2017). Psychological tools used for monitoring training responses of athletes . Perform. Enhanc. Health 5 , 125–133. 10.1016/j.peh.2017.05.001 [ CrossRef ] [ Google Scholar ]
- NCAA (2014). Mind Body and Sport: Understanding and Supporting Student-Athlete Mental Wellness. Independent Publisher . Available online at: https://books.google.com/books?id=JA-5rQEACAAJ (accessed October 01, 2019).
- Pareja-Blanco F., Rodríguez-Rosell D., Sánchez-Medina L., Gorostiaga E., González-Badillo J. (2014). Effect of movement velocity during resistance training on neuromuscular performance . Int. J. Sports Med. 35 , 916–924. 10.1055/s-0033-1363985 [ PubMed ] [ CrossRef ] [ Google Scholar ]
- Pareja-Blanco F., Rodríguez-Rosell D., Sánchez-Medina L., Sanchis-Moysi J., Dorado C., Mora-Custodio R., et al.. (2017). Effects of velocity loss during resistance training on athletic performance, strength gains and muscle adaptations . Scand. J. Med. Sci. Sports 27 , 724–735. 10.1111/sms.12678 [ PubMed ] [ CrossRef ] [ Google Scholar ]
- Paule A. L., Gilson T. A. (2010). Current collegiate experiences of big-time, non-revenue, NCAA athletes . J. Intercoll. Sport 3 , 333–347. 10.1123/jis.3.2.333 [ CrossRef ] [ Google Scholar ]
- Petrie T. A., Deiters J., Harmison R. J. (2014). Mental toughness, social support, and athletic identity: Moderators of the life stress–injury relationship in collegiate football players . Sport Exerc. Perform. Psychol. 3 , 13–27. 10.1037/a0032698 [ CrossRef ] [ Google Scholar ]
- Plews D. J., Laursen P. B., Stanley J., Kilding A. E., Buchheit M. (2013). Training adaptation and heart rate variability in elite endurance athletes: opening the door to effective monitoring . Sports Med. 43 , 773–781. 10.1007/s40279-013-0071-8 [ PubMed ] [ CrossRef ] [ Google Scholar ]
- Podlog L., Eklund R. C. (2007). Professional coaches' perspectives on the return to sport following serious injury . J. Appl. Sport Psychol. 19 , 207–225. 10.1080/10413200701188951 [ CrossRef ] [ Google Scholar ]
- Pyne D. B., Martin D. T. (2011). Fatigue – insights from individual and team sports. in Regulation of Fatigue in Exercise , ed F. E. Marino, (New York, NY: Nova Publishers; ), 177–186. [ Google Scholar ]
- Radcliffe J. N., Comfort P., Fawcett T. (2015). Psychological strategies included by strength and conditioning coaches in applied strength and conditioning: J. Strength . Cond. Res. 29 , 2641–2654. 10.1519/JSC.0000000000000919 [ PubMed ] [ CrossRef ] [ Google Scholar ]
- Reardon C. L., Hainline B., Aron C. M., Baron D., Baum A. L., Bindra A., et al.. (2019). Mental health in elite athletes: international olympic committee consensus statement 2019 . Br. J. Sports Med. 53 , 667–699. 10.1136/bjsports-2019-100715 [ PubMed ] [ CrossRef ] [ Google Scholar ]
- Robson-Ansley P. J., Gleeson M., Ansley L. (2009). Fatigue management in the preparation of Olympic athletes . J. Sports Sci. 27 , 1409–1420. 10.1080/02640410802702186 [ PubMed ] [ CrossRef ] [ Google Scholar ]
- Rushall B. S. (1990). A tool for measuring stress tolerance in elite athletes . J. Appl. Sport Psychol. 2 , 51–66. 10.1080/10413209008406420 [ CrossRef ] [ Google Scholar ]
- Saw A. E., Main L. C., Gastin P. B. (2016). Monitoring the athlete training response: subjective self-reported measures trump commonly used objective measures: a systematic review . Br. J. Sports Med. 50 :281. 10.1136/bjsports-2015-094758 [ PMC free article ] [ PubMed ] [ CrossRef ] [ Google Scholar ]
- Scott B. M., Paskus T. S., Miranda M., Petr T. A., McArdle J. J. (2008). In-season vs. out-of-season academic performance of college student-athletes . J. Intercoll. Sport 1 , 202–226. 10.1123/jis.1.2.202 [ CrossRef ] [ Google Scholar ]
- Selye H. (ed.). (1976). The Stress of Life . New York, NY : McGraw-Hill. [ Google Scholar ]
- Shanley E., Michener L., Ellenbecker T., Rauh M. (2012). Shoulder range of motion, pitch count, and injuries among interscholastic female softball pitchers: a descriptive study . Int. J. Sports Phys. Ther. 7 , 548–557. [ PMC free article ] [ PubMed ] [ Google Scholar ]
- Soligard T., Schwellnus M., Alonso J.-M., Bahr R., Clarsen B., Dijkstra H. P., et al.. (2016). How much is too much? (Part 1) International Olympic Committee consensus statement on load in sport and risk of injury . Br. J. Sports Med. 50 , 1030–1041. 10.1136/bjsports-2016-096581 [ PubMed ] [ CrossRef ] [ Google Scholar ]
- Starling L. T., Nellemann S., Parkes A., Lambert M. I. (2019). The Fatigue and Fitness Test for Teams (FFITT): a practical option for monitoring athletes in a team as individuals . Eur. J. Sport Sci . 20 , 1–9. 10.1080/17461391.2019.1612951 [ PubMed ] [ CrossRef ] [ Google Scholar ]
- Statler T., DuBois A. (2016). Psychology of athletic preparation and performance, in Essentials of Strength Training and Conditioning , eds. G. Haff, and N. T. Triplett (Champaign, IL: Human Kinetics; ), 155–172. [ Google Scholar ]
- Sweet T. W., Foster C., McGuigan M. R., Brice G. (2004). Quantitation of resistance training using the session rating of perceived exertion method . J. Strength Cond. Res. 18 :796. 10.1519/14153.1 [ PubMed ] [ CrossRef ] [ Google Scholar ]
- Szivak T. K., Kraemer W. J. (2015). Physiological readiness and resilience: pillars of military preparedness . J. Strength Cond. Res. 29 , S34–S39. 10.1519/JSC.0000000000001073 [ PubMed ] [ CrossRef ] [ Google Scholar ]
- Taylor K.-L., Chapman D., Cronin J., Newton M., Gill N. (2012). Fatigue monitoring in high performance sport: a survey of current trends . J. Aust. Strength Cond. 20 , 12–23. [ Google Scholar ]
- Thornton H. R., Delaney J. A., Duthie G. M., Dascombe B. J. (2019). Developing athlete monitoring systems in team sports: data analysis and visualization . Int. J. Sports Physiol. Perform. 14 , 698–705. 10.1123/ijspp.2018-0169 [ PubMed ] [ CrossRef ] [ Google Scholar ]
- Thorpe R. T., Atkinson G., Drust B., Gregson W. (2017). Monitoring fatigue status in elite team-sport athletes: implications for practice . Int. J. Sports Physiol. Perfrom. 12 , S2-27-S2–34. 10.1123/ijspp.2016-0434 [ PubMed ] [ CrossRef ] [ Google Scholar ]
- Torres-Ronda L., Schelling X. (2017). Critical process for the implementation of technology in sport organizations . Strength Cond. J. 39 , 54–59. 10.1519/SSC.0000000000000339 [ CrossRef ] [ Google Scholar ]
- Twist C., Highton J. (2013). Monitoring fatigue and recovery in rugby league players . Int. J. Sports Physiol. Perform. 8 , 467–474. 10.1123/ijspp.8.5.467 [ PubMed ] [ CrossRef ] [ Google Scholar ]
- Wallace L. K., Slattery K. M., Coutts A. J. (2009). The ecological validity and application of the session-rpe method for quantifying training loads in swimming . J. Strength Cond. Res. 23 , 33–38. 10.1519/JSC.0b013e3181874512 [ PubMed ] [ CrossRef ] [ Google Scholar ]
- Williams J. M., Andersen M. B. (1998). Psychosocial antecedents of sport injury: review and critique of the stress and injury model . J. Appl. Sport Psychol. 10 , 5–25. 10.1080/10413209808406375 [ CrossRef ] [ Google Scholar ]
- Yang J., Peek-Asa C., Corlette J. D., Cheng G., Foster D. T., Albright J. (2007). Prevalence of and risk factors associated with symptoms of depression in competitive collegiate student athletes . Clin. J. Sport Med. 17 , 481–487. 10.1097/JSM.0b013e31815aed6b [ PubMed ] [ CrossRef ] [ Google Scholar ]

IMAGES
VIDEO
COMMENTS
Regarding doping control in human sports, the International Olympic Committee (IOC) Medical Commission designated anabolic steroids as a banned class in 1974. 35 The term "anabolic agents" emerged in the 1990s to encompass substances like clenbuterol and other β2-agonists, which also possess anabolic activity and are subject to out-of ...
SYNOPSIS This consensus statement is an update of the previous position stand from the American College of Sports Medicine (ACSM), published in 1987 ().Since then, a substantial amount of scientific data on anabolic-androgenic steroids (AAS) has emerged and the circumstances of AAS use has evolved in the athletic, recreational, and clinical communities.
Some athletes consume multiple drugs in addition to anabolic steroids such as alcohol, opioids, cocaine, marijuana, and gamma hydroxybutyrate, some of which can interact adversely with AASs. Polydrug assumption makes it hard to attribute the observed effects to a single drug. AAS effects are also related to sex, dose and duration of administration.
Gynecomastia is a common adverse effect associated with anabolic steroid use. Research has demonstrated a prevalence rate of 37% in anabolic steroid users (O'Sullivan et al., 2000). Gynecomastia isa benign enlargement of the male breast resulting from an altered estrogen-androgen balance, or increased breast sensitivity to a circulating ...
This consensus statement is an update of the 1987 American College of Sports Medicine (ACSM) position stand on the use of anabolic-androgenic steroids (AAS). Substantial data have been collected since the previous position stand, and AAS use patterns have changed significantly. ... Anabolic-Androgenic Steroid Use in Sports, Health, and Society ...
Athletes often take androgenic steroids in an attempt to increase their strength. The efficacy of these substances for this purpose is unsubstantiated, however. We randomly assigned 43 normal men t...
A total of 27 papers evaluating 5 pharmacological interventions met inclusion criteria. ... Guskiewicz KM. Self-reported anabolic-androgenic steroids use and musculoskeletal injuries: findings from the center for the study of retired athletes health survey of retired NFL players. ... et al. ISSN exercise & sports nutrition review update ...
Background The COVID-19 pandemic has had a significant impact on individual health and fitness routines globally. Resistance training, in particular, has become increasingly popular among men and women looking to maintain or improve their physical fitness during the pandemic. However, using Anabolic Steroids (AS) for performance enhancement in resistance training has known adverse effects ...
the Italian athletes on doping practices (Scarpino et al., 1990). 1015 athletes and 216 sports professionals where interviewed during the survey. In total, 30% of athletes, coaches and sports managers and 21% of doctors stated that the athletic perfor-mance can be improved by using drugs or other doping tech-niques.
Generally, athletes administer anabolic steroids by stacking, or progressively increasing the dose over 6-12 weeks in order to then decrease it in the second part of the cycle. ... These modulating agents with specific action on different androgenic receptors are a field for future research in the development of new molecules indicated for ...
Background: Anabolic-androgenic steroids (AAS) are said to be frequently used by both professional and amateur athletes. In addition to having a negative impact on health, steroids defy the concept of fair play. The aim of the study was to evaluate the scale of the problem related to using anabolic-androgenic steroids (AAS) by gym athletes and to analyze possible causes.
Overall, anabolic steroid stigma may dissuade queer men who use steroids and have body image concerns from seeking professional help or being open and honest in-session with practitioners, which ...
abound of the incredible benefits attributed to steroids, the research literature is more skeptical. The use of steroids has been declared illegal in both national and international competition, but this has not deterred the determined athlete. The bottom line is, the use of steroids is said to be commonplace in professional and amateur athletics.
Androgenic-anabolic steroids (AAS) are synthetic derivatives of the male hormone testosterone. ... Effects of androgenic-anabolic steroids in athletes Sports Med. 2004;34(8):513-54. doi: 10.2165/00007256-200434080-00003. Authors Fred Hartgens 1 , Harm Kuipers. Affiliation 1 Department ... Research Support, Non-U.S. Gov't
Doping from the beginning to the present day. Over time, there have been several definitions of doping. Beckmann's sports dictionary describes doping as the use of performance-increasing substances, which would place the athlete on a superior position than that he would normally have obtained. 7 The first official definition of doping dates from 1963 and it was issued by the European Committee ...
This article reviews how steroids affect human muscle physiology and athletic performance, and compares the results with nonhuman animals. It argues that more research is needed on the long-term effects of hormone manipulation on fitness and performance.
This article reviews the prevalence, reasons, and risks of performance-enhancing drug use among recreational athletes, including novel and experimental agents. It also provides guidance for family ...
Learn about the effects, misuse, and testing of anabolic steroids and other appearance and performance enhancing drugs (APEDs) from the National Institute on Drug Abuse (NIDA). Find out how these substances can cause serious health problems, substance use disorders, and legal issues.
Athletes and Steroid Use Croaker5 they surveyed student athletes in Divisions 1, 2, and 3. They found that 80.5 of those students who participated reported to having used alcohol. "Perhaps the most impressive and exhaustive survey of athlete drug use was done by the NCAA (2012) itself in 2009.
Introduction. Doping, defined as use of drugs or other substances for performance enhancement, has become an important topic in virtually every sport1 and has been discovered in athletes of all ages and at every level of competition.2-4 See Table 1 for rates of use of a variety of substances, whether doping agents or recreational substances, among different populations of athletes as ...
The major bibliographical research for this paper was performed by using a search strategy exploring PubMed, Google Scholar as well as PsycNet (accessed 19 January 2022 to 9 May 2022). ... There is limited information in relation to treatment of addiction in athletes which will be a primary focus of this paper. Most of the discussion will focus ...
Background: Although athletic endeavours are associated with a high amount of physical stress and injury, the prevalence of pain is underreported in the sports medicine literature with only a few studies reporting pain on collegiate athletes or exploring sex difference of pain. Impact of pain on athlete availability, training and performance can be mitigated when key epidemiological ...
The purpose of this paper is to discuss stressors experienced by collegiate athletes, developing an early detection system through monitoring techniques that identify the detrimental effects of stress, and discuss appropriate stress management strategies for this population. ... Recent research on relationships between athlete workloads, injury ...